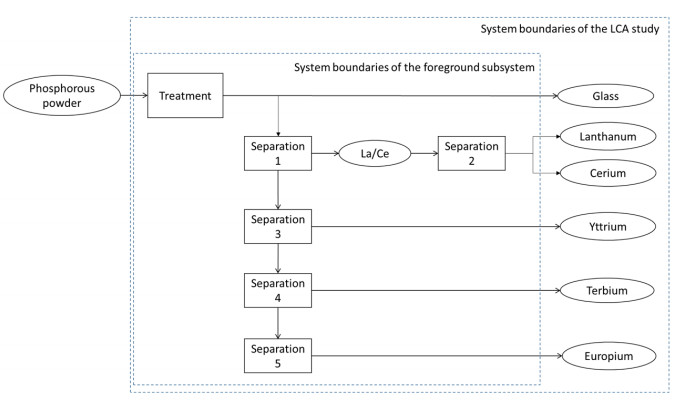
After the rare earth element (REE) crisis in 2011, companies invested in new supply routes of REEs, such as the recycling from end-of-life fluorescent lamps. Although recycling is in the current market situation not economically profitable anymore, it does fit in a strategy towards a low-carbon and a circular economy, for example to mitigate the supply risk of REEs. However, is recycling of REEs indeed environmentally beneficial? Should their recycling therefore be subsidized? This is assessed with a Consequential Life Cycle Assessment (CLCA). The results show that the answer to this question strongly depends on the market situation of the REEs, and the applications in which they are used. At the time that the recycling process was operating—where fluorescent lamps could still displace halogen lamps and there was sufficient demand for the REE europium and yttrium—environmental benefits could be achieved by increasing the recovery of REEs from end-of-life fluorescent lamps. The results of this study can be used to increase the understanding on the type of market interactions that could be considered in the decision-making processes regarding the supply and recycling of raw materials—especially materials that are often produced as by-products, such as many critical raw materials.
Citation: Dieuwertje L. Schrijvers, Philippe Loubet, Guido W. Sonnemann. The influence of market factors on the potential environmental benefits of the recycling of rare earth elements[J]. Clean Technologies and Recycling, 2022, 2(1): 64-79. doi: 10.3934/ctr.2022004
[1] | Grace Inman, Denis Prodius, Ikenna C. Nlebedim . Recent advances in acid-free dissolution and separation of rare earth elements from the magnet waste. Clean Technologies and Recycling, 2021, 1(2): 112-123. doi: 10.3934/ctr.2021006 |
[2] | Andrea Di Maria, Annie Levasseur, Karel Van Acker . Assessing the long term effects on climate change of metallurgical slags valorization as construction material: a comparison between static and dynamic global warming impacts. Clean Technologies and Recycling, 2021, 1(1): 88-111. doi: 10.3934/ctr.2021005 |
[3] | Majid Alipanah, Apurba Kumar Saha, Ehsan Vahidi, Hongyue Jin . Value recovery from spent lithium-ion batteries: A review on technologies, environmental impacts, economics, and supply chain. Clean Technologies and Recycling, 2021, 1(2): 152-184. doi: 10.3934/ctr.2021008 |
[4] | Yi Ji, Edwin E. Kpodzro, Chad T. Jafvert, Fu Zhao . Direct recycling technologies of cathode in spent lithium-ion batteries. Clean Technologies and Recycling, 2021, 1(2): 124-151. doi: 10.3934/ctr.2021007 |
[5] | Nighat Afroz Chowdhury, Apurba Kumar Saha, Gwendolyn Bailey, Andrea Di Maria, Dieuwertje Schrijvers, Hongyue Jin . Life cycle assessment of clean technologies and recycling. Clean Technologies and Recycling, 2023, 3(1): 1-3. doi: 10.3934/ctr.2023001 |
[6] | Kyle Pender, Liu Yang . Glass fibre composites recycling using the fluidised bed: A study into the economic viability in the UK. Clean Technologies and Recycling, 2023, 3(3): 221-240. doi: 10.3934/ctr.2023014 |
[7] | Congying Wang, Fu Zhao, Carol Handwerker . Transforming and integrating informal sectors into formal e-waste management system: A case study in Guiyu, China. Clean Technologies and Recycling, 2022, 2(4): 225-246. doi: 10.3934/ctr.2022012 |
[8] | Joana Beigbeder, Ahmed Allal, Nathalie Robert . Ex-ante life cycle assessment of a partially reusable packaging system for dry-cured ham slices. Clean Technologies and Recycling, 2022, 2(3): 119-135. doi: 10.3934/ctr.2022007 |
[9] | Wilson Uzochukwu Eze, Reginald Umunakwe, Henry Chinedu Obasi, Michael Ifeanyichukwu Ugbaja, Cosmas Chinedu Uche, Innocent Chimezie Madufor . Plastics waste management: A review of pyrolysis technology. Clean Technologies and Recycling, 2021, 1(1): 50-69. doi: 10.3934/ctr.2021003 |
[10] | Susana J. Castillo, Anna Hayes, Greg Colvin, Barrett G. Potter, Rongguang Liang, Krishna Muralidharan . Characterization of recycled Inconel 718 metal powder for assessing its reusability in the laser powder bed fusion process. Clean Technologies and Recycling, 2022, 2(1): 32-46. doi: 10.3934/ctr.2022002 |
After the rare earth element (REE) crisis in 2011, companies invested in new supply routes of REEs, such as the recycling from end-of-life fluorescent lamps. Although recycling is in the current market situation not economically profitable anymore, it does fit in a strategy towards a low-carbon and a circular economy, for example to mitigate the supply risk of REEs. However, is recycling of REEs indeed environmentally beneficial? Should their recycling therefore be subsidized? This is assessed with a Consequential Life Cycle Assessment (CLCA). The results show that the answer to this question strongly depends on the market situation of the REEs, and the applications in which they are used. At the time that the recycling process was operating—where fluorescent lamps could still displace halogen lamps and there was sufficient demand for the REE europium and yttrium—environmental benefits could be achieved by increasing the recovery of REEs from end-of-life fluorescent lamps. The results of this study can be used to increase the understanding on the type of market interactions that could be considered in the decision-making processes regarding the supply and recycling of raw materials—especially materials that are often produced as by-products, such as many critical raw materials.
Rare earth elements (REEs) have been on the radar of many researchers, policymakers, and companies, since the price peak around the year 2011 after export restrictions from China [1,2]. The high prices motivated the rest of the world to find alternative sources of REEs, and many initiatives to increase resource efficiency, find substitutes, increase recycling, and explore new mining areas have been launched [2,3]. Among these efforts, Molycorp reopened the Mountain Pass mine in the United States, which shut down in 2002, and the Mount Weld deposit in Australia increased its production [4]. Furthermore, the company Solvay developed a recycling route that enabled to recover REEs from end-of-life fluorescent lamps [5].
The efforts to increase efficient use of REEs and find substitutes after the REE crisis in 2011 led to a decreased demand for the elements [2]. As a result, prices of REEs decreased by 80% in 2013 [1]. This price drop had as a consequence that several of the recent initiatives were no longer profitable. The Mountain Pass deposit stopped producing in 2015 [6]. The Mount Weld deposit in Australia is still operating, although without being profitable [7]. Solvay suffered the same fate as Molycorp: due to the decreasing prices of the elements, the recycling facility stopped being profitable and had to shut down again [8].
Tukker [9] stated that investments in REE supply chains should not be stalled until the next crisis when operations become economically beneficial for the stakeholders. It takes years until mines become operational so their response will be too slow. The fact that REE prices have stabilized does not take away the problems related to supply risks. These risks include a high concentration of production from China [10], the mismatch between supply and demand of REEs due to the fact that REEs are always produced as by-products of other metals or of each other [11], and the environmental impacts related to mining and refining, which are a few of the reasons why REEs are often identified as "critical" elements for national economies, companies, or low-carbon technologies [12]. Furthermore, recycling fits in the European strategy towards a circular economy and could be expected to receive policy support [13,14]. However, recycling is not necessarily an environmentally friendly alternative for the primary production of REEs, as recycling processes require resources and generate emissions to the environment as well. Therefore, the following question can be raised: "Did the recycling of REEs from end-of-life fluorescent lamps by Solvay after the REE crisis have a beneficial effect on the environment?". This question is still relevant as it enables to (1) formulate and demonstrate an approach to evaluate similar initiatives now and in the future and (2) identify which factors determine the potential environmental benefits of current or future investments in recycling processes as well as in the opening of new mines for REEs and other critical elements. This question is addressed in this paper by a consequential Life Cycle Assessment.
A fluorescent lamp can emit light due to interactions between mercury and phosphors. These phosphors contain the REEs yttrium, europium, terbium, lanthanum, cerium, and gadolinium. At the end of life of the lamp, the lamp is collected to remove the toxic mercury and to recycle glass and aluminum [15]. During this process, a powder is produced that contains the phosphors—which is usually landfilled. The recycling process of Solvay (Figure 1) enables to recover the REEs from the phosphorous powder. The rare earth elements that are produced during the recycling process show similar properties as their primary rare earth oxide (REO) equivalent. The recycled lanthanum, cerium, and terbium can be used to make the green phosphor LaPO4:Ce3+, Tb3+ (LAP). The recycled yttrium and europium are used for the red phosphor Y2O3:Eu3+ (YOX).
Life Cycle Assessment (LCA) is a well-recognized method for the environmental evaluation of products and processes. A distinction can be made between attributional (ALCA) and consequential LCA (CLCA) [17]. ALCA assesses which impacts have taken place and can be attributed to the product under study, limiting the environmental evaluation to the value chain of the product. An attributional LCA on the same recycling process is presented by [16]. CLCA evaluates the impacts that are caused by an additional production or consumption of a product or a service, considering both direct effects in the product value chain and indirect effects related to market interactions that take place as a consequence of this change in production or consumption. To have a complete overview of the environmental impacts caused by the recycling of the REEs, we conduct a CLCA following the steps described by ISO 14040 [18] and ISO 14044 [19]: (1) the goal and scope definition, (2) the life cycle inventory analysis (LCI), (3) the impact assessment, and (4) interpretation of the results.
The method for CLCA that is applied is based on the work published by [20,21], which is implemented in the consequential system model of ecoinvent v.3 [22] and systematically summarized in [23].
The processes that are affected by the changing consumption or production of a product or service depend on whether the increased consumption (or production) of products is followed by an increased (or decreased) supply by the market, or not. By default, supply could be considered unconstrained (increased demand is met by an increased supply, in this paper and by [23] indicated by parameter S = 1) for determining co-products in competitive markets in the long term, where a determining co-product is "a joint product (a product from joint production) for which a change in demand will affect the production volume of the co-producing unit process" [21]. Dependent co-products, including recycled products, are ultimately supply constrained (S = 0) [23]. The determining co-products are identified by the procedure that is presented by [21] and [24,25,26]. For products that are not supply constrained, the marginally affected supplier is the producer that has the lowest long-term operating costs [21,27].
Dependent co-products are produced independently of the existence of a demand. Therefore, for any dependent co-product produced or consumed within the analyzed product system, it is determined whether an additional production is absorbed by the market (unconstrained demand, A = 1, again following the terminology of [23]), or whether it will lead to a surplus (constrained demand, A = 0). The changing consumption or production of a dependent co-product affects storage or waste treatment in case of constrained demand, or the use of this product in other applications in the case of unconstrained demand. The latter results in a changed consumption of an alternative product—i.e. substitution—and potential downstream effects induced by this substitution, for example in distribution, or during the use or end-of-life treatment of the product. The user or product application that is most likely to change its consumption of the dependent co-product, at the expense of an alternative product, is called the marginal user. Ideally, the marginal user is identified by a high price elasticity of demand.
The functional unit of the analysis is "end-of-life treatment of 1 kg of phosphorous powder". The specific research question that is evaluated is: "Did the treatment of 1 kg of phosphorous powder cause lower environmental impacts, or generate higher environmental benefits, relative to the treatment of 1 kg of phosphorous powder by landfilling?". The system boundaries of the two end-of-life treatment processes are presented in Figures 1 and 2 respectively. The foreground subsystem contains the processes that are operated by Solvay. Flows from the foreground subsystem to the background subsystem are products that are sold to the market. It is assumed that the consequences of the decision to recycle or landfill the phosphorous powder are marginal [21]. The time horizon of the assessment is the long term, allowing for investment decisions considering technologies that were available during the operation timeframe of the recycling facility (2013–2016).
The intended audience are decision-makers that wish to evaluate the potential contribution of recycling to a more circular use and environmentally friendly supply of critical raw materials. This audience could include:
- the recycling company that wishes to evaluate the potential benefits of the recycling activity, as well as
- policymakers that wish to decide whether this recycling route merits policy support from an environmental point of view and whether therefore subsidies are justified to foster the recycling from an economic cost-benefits point of view.
Inventory data of the foreground processes are provided by Solvay and are treated confidentially (F. Lartigue-Peyrou, Solvay (2016), personal communication). Data of the background processes are mostly taken from the consequential system model of ecoinvent 3.7.1. If these data are not available from this database, they are based on literature. Data are as much as possible collected from the period in which the recycling facility was in operation (2013–2016). The results of the inventory analysis are presented in the Results section.
The impact assessment is done in Simapro 9.1.1.1 with the method EF 3.0 Method (adapted) 1.01, which is based on the impact assessment method of the Environmental Footprint initiative and includes some adaptations for SimaPro [28].
During the valorization process, some glass and several REEs—lanthanum, cerium, terbium, europium, and yttrium are valorized. It is assumed that recycled glass can substitute Ordinary Portland cement after additional grinding [29]. The recycled REEs can be used in the same applications and by the same users as their primary equivalent. The consequences of the substitution of primary REEs are discussed below.
The following paragraphs describe the consequences of the substitution of primary REEs, due to the production of REEs by the recycling process, which are reflected in the LCI. REEs are in most cases produced from REE-rich tailings of mining activities of other metals, making REEs dependent co-products of the host metal [30]. In the assessed period, REEs were the primary product from the Mountain Pass mine in California, from mines in Sichuan and the ion adsorption-type ores in China. Also the Mt. Weld, Yangibana and John Galt deposits in Australia (had the potential to) produce REEs as the main product [30,31]. An analysis of the determining co-product of these deposits and the subsequent identification of the marginal supplier is provided in Section S1 in the Supplementary Information (SI). It appears that only neodymium, yttrium, and dysprosium are determining co-products of mines that are expected to change their output with a decreased demand for primary REEs. It must, however, be noted that the identification of yttrium as a determining co-product is unlikely to be stable in the long term, due to changing market conditions (as discussed below). These products will have a value of S = 1 (Table 1)—i.e. supply is considered unconstrained. Supply of REEs that are not a determining co-product from a primary production route (e.g. europium and terbium) is assumed to be fully constrained. These REEs have a value of S = 0 in Table 1.
Rare earth element | Supply constrained? (S)a | Current situation | Market outlook (2020) | Demand constrained? (A)b | Affected process |
Lanthanum | 0 | Surplus | Surplus | 0 | Storage |
Cerium | 0 | Surplus | Surplus | 0 | Storage |
Praseodymium | 0 | Surplus | Surplus | 0 | Storage |
Neodymium | 1 | Surplus | Tight | 1 | Xunwu deposit |
Samarium | 0 | Large surplus | Large surplus | 0 | Storage |
Europium | 0 | Shortage | Shortage | 1 | Marginal user (scenarios) |
Gadolinium | 0 | Surplus | Surplus | 0 | Storage |
Terbium | 0 | Surplusc | Shortage | 1 | Marginal user (permanent magnet) |
Dysprosium | 1 | Surplus | Tight | 1 | Southeast Guangdong deposit |
Holmium | 0 | Large surplus | Large surplus | 0 | Storage |
Erbium | 0 | Surplusc | Surplus | 0 | Storage |
Thulium | 0 | Large surplus | Large surplus | 0 | Storage |
Ytterbium | 0 | Large surplus | Large surplus | 0 | Storage |
Lutetium | 0 | Large surplus | Large surplus | 0 | Storage |
Yttrium | 1 | Shortage | Shortage | 1 | Longnan deposit |
Notes: aIf S = 1, supply is unconstrained and if S = 0, supply is constrained. bIf A = 1, demand is unconstrained and if A = 0, demand is constrained. c[4] is not conclusive about the market situation of terbium and erbium. The text of the report indicates a significant surplus, while a graph shows a current shortage of both elements and a small surplus for erbium and a shortage for terbium in 2020. |
Secondly, we identify demand constraints of REEs. The market situation for all REEs in the relevant time horizon has been analyzed by [4] and is summarized in Table 1. A material that is produced in surplus and which is stored has a value of A = 0, meaning that demand is constrained. This is the case for lanthanum, cerium, praseodymium, samarium, gadolinium, holmium, erbium, thulium, ytterbium and lutetium [4,11]. Neodymium, europium, terbium, dysprosium, and yttrium are not produced in surplus and have a value of A = 1.
The marginal suppliers of the determining co-products neodymium, dysprosium, and yttrium are the Xunwu, Southeast Guangdong, and Longnan deposits in China, respectively (Section S1 of the SI), which are all deposits of ionic ore. Therefore, for these production routes, the data of [32] in combination with the consequential system model of ecoinvent 3.7.1 are used. Due to lack of deposit-specific data, it is assumed that the inventory of the mining process for each site is the same. Only the elementary flows of REEs and the outputs of rare earth oxides (REOs) are adjusted to the composition corresponding to each mine [33]. A relatively low recovery rate of 40% is assumed and the high inventory estimates without reuse of the leachate are used, corresponding to a low ore grade, which we consider to be the marginally affected ore grade.
REEs that are only produced as dependent co-products (S = 0) and that are produced in surplus (A = 0) can be supplied from storages. Elements could be stored in separated form, or in combination with other elements. Therefore, the supply of some elements from storage might require additional separation. In this LCA, the inventory due to the separation process is scaled down to the inventory that is needed to only extract the determining co-product. It is assumed that the inventory of the separation effort is linearly related to the mass output of the separation activity, regardless of the composition of the REE concentrate. A detailed description of the modeling of the separation process is given in Section S2 of the SI. [32] presents low and high inventory estimates for the separation process. We used the low estimates, and we did not consider potential impacts related to the storage activity itself.
Neodymium, yttrium, and dysprosium are not only produced as determining co-products but also as dependent co-products of other REE-containing ores. Each dependent co-product could substitute their determining equivalent.
Europium and terbium are only supplied as dependent co-products (S = 0), while the demand for these elements is unconstrained (A = 1). An additional availability of these materials will lead to an increased use by their marginal user, i.e., the application that can most easily use an alternative material if the price of one of these materials becomes too high. In this LCA, it is assumed that the marginal user of terbium are permanent magnets, in which terbium displaces dysprosium in a 1:1 mass ratio. A more detailed discussion about the marginal user of terbium is provided in Section S3 of the SI.
Europium is mostly used within phosphors for compact fluorescent lamps (CFLs), LEDs and video screens [4]. The income elasticity and own-price elasticity of demand for CFLs are relatively high, which indicates that a CFL is a luxury good [34]. From this, we conclude that the CFL is the marginal user of europium. There is no direct substitute for europium in phosphors [2]. However, substitutes could be identified on different levels [35]: detailed composition, component, sub-assembly and conceptual—such as the use of a CFL to provide the function of light. The mechanism of substitution is based on the assumption that an increased (or decreased) demand for a product results in an increased (or decreased) price and subsequently a decreased (or increased) demand by the marginal user [36,37]. While it is debatable whether an increased price for europium directly leads to a decreased production of fluorescent lamps due to the small quantities used, an unstable market for an essential raw material does motivate the development of substitutes [2]. LEDs are often identified as good substitutes for the use of europium in CFLs, even though they still had a higher purchasing price than CFLs in the evaluated time frame (2010–2014) [2,4,38]. It must, however, be noted that LEDs are cheaper when energy consumption during use and lamp replacements are taken into account. A decreasing price for europium—due to an increased availability on the market via the recycling process—could delay the transition towards more energy efficient LED lamps. However, in 2016, CFLs still competed with less energy efficient halogen lamps, and in some cases even with incandescent lamps. Both LED and halogen lamps are considered to be good substitutes of CFLs. However, substitution is associated with relevant downstream effects, due to the differences in energy efficiency and the lifetime of the products. As it is not exactly known what lamp would be substituted by CFLs if more europium becomes available, three scenarios are investigated that represent different market situations of the CFL:
(1) Fluorescent lamps are only competing with LED lamps: All the europium on the market is fully used, resulting in a value of A = 1 for primary europium. The increased production of europium leads to an increased availability of europium for CFLs. Consequently, the demand for LED lamps decreases. The supply of LED lamps is assumed to be unconstrained. Hence, fewer LED lamps will be produced, which however have a longer lifetime and a higher energy efficiency than fluorescent lamps.
(2) Fluorescent lamps are only competing with halogen lamps: All the europium on the market is fully used, resulting in a value of A = 1 for primary europium. The increased production of europium leads to an increased availability of europium for CFLs. Consequently, the demand for halogen lamps decreases. The supply of halogen lamps is assumed to be unconstrained. Hence, fewer halogen lamps will be produced, which have a shorter lifetime and a lower energy efficiency than fluorescent lamps.
(3) Fluorescent lamps are becoming superfluous, and europium is supplied from storage: If CFLs are widely displaced by LED lights, there could be an oversupply of europium [2,39]. In this scenario, europium has a value of A = 0. Consequently, the increased production of europium results in the additional storage of europium.
Supplying 1 kg of europium to the market means that 33.000 more CFLs can be fabricated in Scenarios 1 and 2 [40]. This results in an increased consumption of the materials that are required for a CFL, as well as the increased energy use and end-of-life treatment of this lamp. On the contrary, it leads to a decreased demand for either 6.600 LED lamps (Scenario 1) or 193.000 halogen lamps (Scenario 2)—as well as their full life cycles, respectively. The inventory related to the three types of lamps is based on [41] and described in detail in Section S4 of the SI. In this study, no impacts are associated with the storage of europium in Scenario 3.
Landfilling of phosphorous powder is modelled by the ecoinvent dataset "Hazardous waste, for underground deposit {RER}| market for hazardous waste, for underground deposit | Conseq, S", referring to a European process ("RER"), from the aggregated consequential system model of ecoinvent ("Conseq, S"). The waste can be considered "hazardous" due to the mercury content. This dataset is in line with the life cycle model of the end-of-life of fluorescent lamps of [15].
Figure 3 presents the relative impacts that are caused by the additional end-of-life treatment of 1 kg of phosphorous powder via landfilling or recycling via the three scenarios for the marginal use of europium. Figure 4 shows the comparison between recycling (Scenario 3) and landfilling, as these results are less visible in Figure 3. The absolute results are shown in Table 2.
Impact category | Unit | Recycling (Scenario 1) | Recycling (Scenario 2) | Recycling (Scenario 3) | Landfilling |
Climate change | kg CO2 eq | 1.7E+03 | -1.6E+03 | 2.0E-01 | 3.3E-01 |
Ozone depletion | kg CFC11 eq | 1.8E-04 | -3.0E-05 | -1.3E-07 | 1.7E-08 |
Ionising radiation | kBq U-235 eq | -1.7E+01 | -8.0E+01 | -2.2E-01 | 2.1E-03 |
Photochemical ozone formation | kg NMVOC eq | 9.0E+00 | -1.3E+01 | -4.0E-03 | 1.6E-03 |
Particulate matter | disease inc. | 1.4E-04 | -2.4E-04 | -1.4E-07 | 2.1E-08 |
Human toxicity. non-cancer | CTUh | 9.8E-05 | -1.8E-04 | -1.1E-09 | 6.9E-09 |
Human toxicity. cancer | CTUh | 2.8E-06 | -8.8E-06 | -3.4E-10 | 1.3E-09 |
Acidification | mol H + eq | 8.2E+01 | 7.0E+01 | -1.1E-02 | 1.2E-03 |
Eutrophication. freshwater | kg P eq | 1.6E-02 | -8.5E-01 | -3.9E-04 | 1.5E-04 |
Eutrophication. marine | kg N eq | 3.6E+00 | -6.3E+00 | -3.3E-01 | 3.1E-04 |
Eutrophication. terrestrial | mol N eq | 1.9E+01 | -8.8E+01 | -7.5E-03 | 3.1E-03 |
Ecotoxicity. freshwater | CTUe | 6.6E+03 | -3.4E+05 | -7.6E+02 | 8.3E+00 |
Land use | Pt | 1.0E+05 | -4.5E+05 | -9.5E+02 | 4.0E+00 |
Water use | m3 depriv. | 4.1E+02 | -5.4E+02 | -7.0E+00 | 4.9E-02 |
Resource use. fossils | MJ | 1.7E+04 | -1.5E+04 | 3.3E+00 | 3.5E+00 |
Resource use. minerals and metals | kg Sb eq | -1.3E-01 | -7.1E-01 | -1.2E-05 | -5.1E-06 |
The recycling of phosphorous powder seems to cause higher environmental impacts than the landfilling of the powder in Scenario 1, and lower impacts in Scenarios 2 and 3. The end-of-life treatment option that causes the highest environmental impacts is the recycling of the powder, in Scenario 1. This is explained by the fact that the recycling process produces recycled europium. In this scenario, the increased availability of europium in the market results in an increased production, use, and end-of-life treatment of CFLs, at the expense of more energy-efficient LED lamps. An opposite effect is observed in Scenario 2, where the recycling of the powder causes high environmental benefits. This is the result of the energy savings obtained in Scenario 2, due to the use of CFLs instead of halogen lamps, which have a higher energy consumption. However, the high contribution to acidification in Scenario 1 and Scenario 2 is caused by the use of the capacitor in the CFL. Even if primary europium is supplied from storage (Scenario 3), recycling of phosphorous is still environmentally beneficial compared with landfilling, due to the substitution of the primary extraction of yttrium.
It must be noted that the results are extremely sensitive to the different scenarios for europium and that the differences in the impacts of recycling and landfilling are very small in Scenario 3. If Scenario 3 is considered most relevant among the recycling scenarios, it is recommended to do a more detailed comparison between Scenario 3 and the landfilling scenario, including case-specific data collection for the landfilling process. This allows to investigate the potential influence of data uncertainty on the results.
The inventory data of primary REEs is determined by three lines of calculations: (1) identification of marginal supply routes, (2) identification of the determining co-product, and (3) the calculation of the inventory of the supply route. The market for REEs is rapidly changing [11,42], which therefore limits the validity of the LCA results over time. For example, ion adsorption deposits are only operational for 1–5 years and the Longnan deposit that was identified as the marginal supplier for yttrium appeared to be no longer operational in 2017 [32]. The identification of yttrium, neodymium, and dysprosium as determining co-products via the procedure outlined by [26] is uncertain due to lacking information about up-to-date market prices and marginal operating costs, as well as highly-fluctuating prices of REEs. However, cross-checking the outcomes of our calculations with the market analysis of [4] suggested that this identification was correct for the studied time period. In the future, the demand for yttrium is expected to decrease, due to a declining need for REO in lighting applications [13]. Hence, is interesting to evaluate whether the recycling of YOX is environmentally beneficial when not only europium, but also yttrium is supplied from storage. A sensitivity analysis evaluating this scenario, shown in Figure 5, shows that recycling is environmental beneficial for the impact categories ionizing radiation, marine eutrophication, freshwater ecotoxicity, land use, and water use, due to the co-production of terbium and the subsequent substitution of the mining of dysprosium. Recycling causes furthermore lower environmental impacts than landfilling regarding the emission of particulate matter. However, regarding the other impact categories, the potential environmental benefits of recycling do not compensate the impacts caused by recycling process itself, hence, recycling is more environmentally damaging than landfilling of the phosphorous powder.
A limited set of supply and demand constraints is investigated, and determining co-products are assumed to be free of supply constraints. This assumption was considered reasonable in a competitive market in the long term. However, the market for REEs is not a typical competitive market. China has a monopoly-like position in the supply of REEs and showed their capability to influence market prices by supply restrictions [3], while in a competitive market suppliers are price-takers [43]. This means that the supply of primary yttrium, neodymium, and dysprosium is probably not fully elastic. In that case, marginal users of these elements should be identified as well. It is expected that the consideration of marginal users for these elements has a similar effect on the results as the identification of the marginal user for primary europium—i.e. the additional or decreased availability of REEs for the use in energy-efficient or low-carbon technologies (e.g. the use of neodymium and dysprosium in wind turbines) will have a beneficial or harmful effect on the environment, respectively.
Finally, uncertainty regarding inventory data merits discussion. We used the same inventory data to represent the three different production routes of yttrium, neodymium, and dysprosium—only the elementary flows of the metals contained in the respective deposits were adapted—whereas these production routes could be expected to generate different inventory flows. Also, the separation process of the REEs is highly simplified. Parameterized data adapted to the ratio of the different REEs in the REE mixture would be ideal to represent the different steps of the separation procedure. Furthermore, dependent co-products (REEs) for which the demand is constrained are in this analysis supplied by storage. No inventory is associated with storage. It should be further analyzed what type of impacts can take place during storage, and how the time dimension of storage can be captured in an inventory dataset. However, our results suggest that the inclusion or exclusion of processes within the LCI—represented by the different market scenarios of primary europium and primary yttrium—has a larger influence on the results than the remaining data uncertainties after the determination of the processes that are part of the inventory. Also the inventory data for the landfilling process is prone to uncertainties and geographical variability. A more detailed analysis of landfilling impacts is recommended in the scenario where europium and yttrium are supplied from stockpiles, as specific landfilling conditions may affect the relative environmental performance of recycling. Finally, the consequential system model of ecoinvent v. 3.7.1. was used to provide background data. This is currently the only consequential database available, and the database is relatively immature regarding the modelled consequences and the data risk to be quickly outdated. The relevance of a consequential analysis should encourage future development and updating of consequential background data.
This paper aimed to answer the question "Did the recycling of REEs from end-of-life fluorescent lamps by Solvay after the REE crisis have a beneficial effect on the environment?" applying a consequential LCA. The results show that the answer to this question strongly depends on the market situation of the REEs, and the applications in which they are used: Increased recycling of REEs from fluorescent lamps was beneficial for the environment in the analyzed market situation (for the years 2013–2016) where fluorescent lamps still displaced halogen lamps, there were no surpluses of europium, and yttrium was still a determining product for some REE mines. Under these circumstances, the recycling process would have merited policy support, e.g. in the form of subsidies, when the recycling of the REEs was not economically viable due to market circumstances (such as governmental interventions in other producing countries or unfair competition due to differences in environmental and social mining standards) that may permit to keep prices of primary REEs low. Increased recovery of REEs is no longer beneficial for the environment, either when primary europium and yttrium are produced as an excess by-product for storage, or when the expected reduction in price for europium might lead to an increased production of fluorescent lamps, delaying the transition towards more energy efficient (LED) technologies. In such market situations, subsidies to continue the recycling of these REEs cannot be supported on environmental grounds. Therefore, we argue that public decisions on supporting recycling activities should take such shifts in primary metal markets into consideration.
The results of this paper can be used to increase the understanding on the type of market interactions that should be considered in the decision-making processes regarding the supply and demand of raw materials—especially materials that are often produced as by-products, such as many critical raw materials. The approach applied in this study has been proven to be a useful tool to identify whether future recycling projects or investments in new primary supply routes of these materials can have a beneficial effect on the environment. This approach is similarly applicable in any other sector where investment decisions must be made. In order to update our analysis to more recent market developments, up-to-date data are needed, including a list of (soon-to-be) operational mines that produce REEs as main output, an overview of the relative content of the individual REEs in these deposits, market prices and market trends for each individual element, and a market analysis on the uses of the individual elements—ideally supplemented by price elasticities of demand for each application. However, we could conclude that it is generally environmentally beneficial to recycle critical raw materials under the following conditions:
- The increased availability of the recycled material results in either a decreased production of a primary material or an increased use of this material by a relatively "clean" technology,
- Alternatives to this clean technology are more impactful, not only on the level of primary production but considering the whole product system. This includes downstream impacts related to manufacturing, transport, use and waste treatment,
- The impacts related to the recycling process do not outweigh the benefits of using the cleaner technology.
While these key points might be straightforward for an LCA expert, it is important that these considerations are also highlighted in more diverse audiences, including investors and policy makers that are, for example, in charge of mitigation measures for critical raw materials or the transition towards a circular economy.
This work has been funded by Solvay Group and the French National Association for Technical Research (CIFRE Convention N°2013/1146). The inventory data of the rare earth recycling process was provided by Françoise Lartigue-Peyrou on behalf of Solvay. The Solvay Research & Innovation team "Eco-design, Modeling & Simulation" in the Department of Research & Development for Sustainable Processes supported this work, which was greatly appreciated by the authors. The authors would like to thank in particular Jean-François Viot and Françoise Lartigue-Peyrou for their contributions to the manuscript. Finally, we thank the reviewers of the manuscript for their valuable feedback.
The authors declare no competing financial interest.
[1] |
Guyonnet D, Planchon M, Rollat A, et al. (2015) Material flow analysis applied to rare earth elements in Europe. J Clean Prod 107: 215-228. https://doi.org/10.1016/j.jclepro.2015.04.123 doi: 10.1016/j.jclepro.2015.04.123
![]() |
[2] | Salla Ahonen, Arvanitidis N, Auer A, et al. (2015) Strengthening the European Rare Earths Supply-Chain: Challenges and policy options-A report by the European Rare Earths Competency Network (ERECON). Available from: https://hal-cea.archives-ouvertes.fr/cea-01550114/document. |
[3] |
Schlinkert D, van den Boogaart KG (2015) The development of the market for rare earth elements: Insights from economic theory. Resour Policy 46: 272-280. https://doi.org/10.1016/j.resourpol.2015.10.010 doi: 10.1016/j.resourpol.2015.10.010
![]() |
[4] | European Commission (2015) Report on critical raw materials for the EU-Critical raw materials profiles. Available from: https://ec.europa.eu/docsroom/documents/11911/attachments/1/translations. |
[5] | Solvay (2012) Solvay launches its rare earth recycling activity in France. Available from: http://www.solvay.com/nl/media/press_releases/20120927-coleopterre.html. |
[6] | GlobeNewswire (2015) Molycorp to move its mountain pass rare earth facility to 'care and maintenance' mode. Available from: http://globenewswire.com/news-release/2015/08/26/763530/0/en/Molycorp-to-Move-Its-Mountain-Pass-Rare-Earth-Facility-to-Care-and-Maintenance-Mode.html. |
[7] | Lynas Corporation LTD (2016) FY16 Financial Report. Available from: https://www.lynascorp.com/Shared Documents/Investors and media/Reporting Centre/Annual reports/2016/160929 FY16 Financial Report 1548914.pdf. |
[8] | Sud Ouest (2016) La Rochelle: closure of Solvay's rare earth recycling plant by the end of 2016 (in French). Available from: https://www.sudouest.fr/economie/la-rochelle-fermeture-de-l-atelier-de-recyclage-des-terres-rares-de-solvay-d-ici-fin-2016-4728900.php#:~:text=ArchivesL%C3%A9galesCarnet-,La%20Rochelle%20%3A%20fermeture%20de%20l'atelier%20de%20recyclage%20des%20terres, Solvay%20d'ici%20fin%202016&text=Le%20groupe%20Solvay%20(ex%2DRhodia, personnes%2C%20les%20deux%20sites%20confondus. |
[9] |
Tukker A (2014) Rare earth elements supply restrictions: Market failures, not scarcity, hamper their current use in high-tech applications. Environ Sci Technol 48: 9973-9974. https://doi.org/10.1021/es503548f doi: 10.1021/es503548f
![]() |
[10] | European Commission (2017) Study on the review of the list of critical raw materials-Critical raw materials factsheets. Available from: https://op.europa.eu/en/publication-detail/-/publication/7345e3e8-98fc-11e7-b92d-01aa75ed71a1/language-en. |
[11] |
Binnemans K, Jones PT, Van Acker K, et al. (2013) Rare-earth economics: the balance problem. JOM 65: 10-12. https://doi.org/10.1007/s11837-013-0639-7 doi: 10.1007/s11837-013-0639-7
![]() |
[12] |
Schrijvers D, Hool A, Blengini GA, et al. (2020) A review of methods and data to determine raw material criticality. Resour Conserv Recy 155: 104617. https://doi.org/10.1016/j.resconrec.2019.104617 doi: 10.1016/j.resconrec.2019.104617
![]() |
[13] |
Rollat A, Guyonnet D, Planchon M, et al. (2015) Prospective analysis of the flows of certain rare earths in Europe at the 2020 horizon. Waste Manage 49: 427-436. https://doi.org/10.1016/j.wasman.2016.01.011 doi: 10.1016/j.wasman.2016.01.011
![]() |
[14] | European Commission (2018) Circular economy-Implementation of the circular economy action plan. Available from: http://ec.europa.eu/environment/circular-economy/index_en.htm. |
[15] |
Tan Q, Song Q, Li J (2015) The environmental performance of fluorescent lamps in China, assessed with the LCA method. Int J Life Cycle Assess 20: 807-818. https://doi.org/10.1007/s11367-015-0870-2 doi: 10.1007/s11367-015-0870-2
![]() |
[16] |
Schrijvers DL, Loubet P, Sonnemann G (2021) "Allocation at the point of substitution" applied to recycled rare earth elements: what can we learn? Int J Life Cycle Assess 26: 1403-1416. https://doi.org/10.1007/s11367-021-01884-3 doi: 10.1007/s11367-021-01884-3
![]() |
[17] | Sonnemann GW, Vigon BW (2011) Global guidance principles for life cycle assessment databases-A basis for greener processes and products. Available from: https://www.lifecycleinitiative.org/wp-content/uploads/2012/12/2011%20-%20Global%20Guidance%20Principles.pdf. |
[18] | ISO 14040: Environmental management-Life cycle assessment-Principles and framework. The International Organization for Standardization, 2006. Available from: https://www.iso.org/standard/37456.html. |
[19] | ISO 14044: Environmental management-Life cycle assessment-Requirements and guidelines. The International Organization for Standardization, 2006. Available from: https://www.iso.org/obp/ui/#iso:std:iso:14044:en. |
[20] |
Ekvall T, Weidema BP (2004) System boundaries and input data in consequential life cycle inventory analysis. Int J Life Cycle Assess 9: 161-171. https://doi.org/10.1007/BF02994190 doi: 10.1007/BF02994190
![]() |
[21] | Weidema BP, Ekvall T, Heijungs R (2009) Guidelines for application of deepened and broadened LCA-Deliverable D18 of work package 5 of the CALCAS project. Available from: http://citeseerx.ist.psu.edu/viewdoc/download?doi=10.1.1.628.948&rep=rep1&type=pdf. |
[22] | Weidema BP, Bauer C, Hischier R, et al. (2013) Overview and Methodology: Data Quality Guideline for the Ecoinvent Database Version 3. St. Gallen: The Ecoinvent Centre. |
[23] |
Schrijvers DL, Loubet P, Weidema BP (2021) To what extent is the Circular Footprint Formula of the Product Environmental Footprint Guide consequential? J Clean Prod 320: 128800. https://doi.org/10.1016/j.jclepro.2021.128800 doi: 10.1016/j.jclepro.2021.128800
![]() |
[24] | Consequential-LCA (2015) Further theory on normalising market trends. Available from: https://consequential-lca.org/. |
[25] | Consequential-LCA (2015) Further theory on marginal production costs. Available from: https://consequential-lca.org/. |
[26] | Consequential-LCA (2015) When all co-products have alternatives. Available from: https://consequential-lca.org/. |
[27] |
Kätelhön A, von der Assen N, Suh S, et al. (2015) Industry-cost-curve approach for modeling the environmental impact of introducing new technologies in life cycle assessment. Environ Sci Technol 49: 7543-7551. https://doi.org/10.1021/es5056512 doi: 10.1021/es5056512
![]() |
[28] | PRé (2022) About SimaPro. Available from: https://simapro.com/about/. |
[29] |
Kara P, Korjakins A, Kovalenko K (2012) The usage of fluorescent waste glass powder in concrete. Constr Sci 13: 26-32. https://doi.org/10.2478/v10311-012-0004-z doi: 10.2478/v10311-012-0004-z
![]() |
[30] | Krishnamurthy N, Gupta CK (2016) Extractive Metallurgy of Rare Earths, 2 Ed., Boca Raton: CRC Press. https://doi.org/10.1201/b19055 |
[31] | Long KR, Van Gosen BS, Foley NK, et al. (2012) The principal rare earth elements deposits of the United States-A summary of domestic deposits and a global perspective. In: Sinding-Larsen R, Wellmer FW, Non-Renewable Resource Issues. International Year of Planet Earth, 1 Ed., Dordrecht: Springer. https://doi.org/10.1007/978-90-481-8679-2_7 |
[32] |
Schulze R, Lartigue-Peyrou F, Ding J, et al. (2017) Developing a life cycle inventory for rare earth oxides from ion-adsorption deposits: key impacts and further research needs. J Sustain Metall 3: 753-771. https://doi.org/10.1007/s40831-017-0139-z doi: 10.1007/s40831-017-0139-z
![]() |
[33] | USGS (2015) 2012 Minerals Yearbook-Rare Earths. Available from: https://s3-us-west-2.amazonaws.com/prd-wret/assets/palladium/production/mineral-pubs/rare-earth/myb1-2012-raree.pdf. |
[34] | Holland C, Energy N, Alliance E (2014) Are LEDs the next CFL: a diffusion of innovation analysis. ACEEE Summer Study Energy Effic Build 2014: 184-196. |
[35] | Habib K (2015) Critical resources in clean energy technologies and waste flows[PhD's thesis]. University of Southern Denmark, Denmark. |
[36] | National Research Council (2008) Minerals, Critical Minerals, and the U.S. Econom, Washington DC: National Academies Press. |
[37] |
Eggert RG (2011) Minerals go critical. Nat Chem 3: 688-691. https://doi.org/10.1038/nchem.1116 doi: 10.1038/nchem.1116
![]() |
[38] | Guyonnet D, Planchon M, Rollat A, et al. (2014) Primary and secondary sources of rare earths in the EU-28: results of the ASTER project. ERES 2014-1st Conference on European Rare Earth Resources, 66-72. |
[39] | Binnemans K (2014) Economics of rare earths: the balance problem. Proceedings of the 1st European Rare Earth Resources Conference (ERES 2014), 37-46. |
[40] | Chu S (2011) Critical Materials Strategy, Collingdale: DIANE Publishing. |
[41] | Scholand M, Dillon H (2013) Life-Cycle Assessment of Energy and Environmental Impacts of LED Lighting Products-Part 2: LED Manufacturing and Performance, Richland: U.S. Department of Energy. https://doi.org/10.2172/1044508 |
[42] |
Binnemans K, Jones PT, Müller T, et al. (2018) Rare earths and the balance problem: how to deal with changing markets? J Sustain Metall 4: 126-146. https://doi.org/10.1007/s40831-018-0162-8 doi: 10.1007/s40831-018-0162-8
![]() |
[43] | Weidema BP (2003) Market Information in Life Cycle Assessment, Copenhagen: Denmark. |
![]() |
![]() |
1. | Nighat Afroz Chowdhury, Apurba Kumar Saha, Gwendolyn Bailey, Andrea Di Maria, Dieuwertje Schrijvers, Hongyue Jin, Life cycle assessment of clean technologies and recycling, 2023, 3, 2770-4580, 1, 10.3934/ctr.2023001 |
Rare earth element | Supply constrained? (S)a | Current situation | Market outlook (2020) | Demand constrained? (A)b | Affected process |
Lanthanum | 0 | Surplus | Surplus | 0 | Storage |
Cerium | 0 | Surplus | Surplus | 0 | Storage |
Praseodymium | 0 | Surplus | Surplus | 0 | Storage |
Neodymium | 1 | Surplus | Tight | 1 | Xunwu deposit |
Samarium | 0 | Large surplus | Large surplus | 0 | Storage |
Europium | 0 | Shortage | Shortage | 1 | Marginal user (scenarios) |
Gadolinium | 0 | Surplus | Surplus | 0 | Storage |
Terbium | 0 | Surplusc | Shortage | 1 | Marginal user (permanent magnet) |
Dysprosium | 1 | Surplus | Tight | 1 | Southeast Guangdong deposit |
Holmium | 0 | Large surplus | Large surplus | 0 | Storage |
Erbium | 0 | Surplusc | Surplus | 0 | Storage |
Thulium | 0 | Large surplus | Large surplus | 0 | Storage |
Ytterbium | 0 | Large surplus | Large surplus | 0 | Storage |
Lutetium | 0 | Large surplus | Large surplus | 0 | Storage |
Yttrium | 1 | Shortage | Shortage | 1 | Longnan deposit |
Notes: aIf S = 1, supply is unconstrained and if S = 0, supply is constrained. bIf A = 1, demand is unconstrained and if A = 0, demand is constrained. c[4] is not conclusive about the market situation of terbium and erbium. The text of the report indicates a significant surplus, while a graph shows a current shortage of both elements and a small surplus for erbium and a shortage for terbium in 2020. |
Impact category | Unit | Recycling (Scenario 1) | Recycling (Scenario 2) | Recycling (Scenario 3) | Landfilling |
Climate change | kg CO2 eq | 1.7E+03 | -1.6E+03 | 2.0E-01 | 3.3E-01 |
Ozone depletion | kg CFC11 eq | 1.8E-04 | -3.0E-05 | -1.3E-07 | 1.7E-08 |
Ionising radiation | kBq U-235 eq | -1.7E+01 | -8.0E+01 | -2.2E-01 | 2.1E-03 |
Photochemical ozone formation | kg NMVOC eq | 9.0E+00 | -1.3E+01 | -4.0E-03 | 1.6E-03 |
Particulate matter | disease inc. | 1.4E-04 | -2.4E-04 | -1.4E-07 | 2.1E-08 |
Human toxicity. non-cancer | CTUh | 9.8E-05 | -1.8E-04 | -1.1E-09 | 6.9E-09 |
Human toxicity. cancer | CTUh | 2.8E-06 | -8.8E-06 | -3.4E-10 | 1.3E-09 |
Acidification | mol H + eq | 8.2E+01 | 7.0E+01 | -1.1E-02 | 1.2E-03 |
Eutrophication. freshwater | kg P eq | 1.6E-02 | -8.5E-01 | -3.9E-04 | 1.5E-04 |
Eutrophication. marine | kg N eq | 3.6E+00 | -6.3E+00 | -3.3E-01 | 3.1E-04 |
Eutrophication. terrestrial | mol N eq | 1.9E+01 | -8.8E+01 | -7.5E-03 | 3.1E-03 |
Ecotoxicity. freshwater | CTUe | 6.6E+03 | -3.4E+05 | -7.6E+02 | 8.3E+00 |
Land use | Pt | 1.0E+05 | -4.5E+05 | -9.5E+02 | 4.0E+00 |
Water use | m3 depriv. | 4.1E+02 | -5.4E+02 | -7.0E+00 | 4.9E-02 |
Resource use. fossils | MJ | 1.7E+04 | -1.5E+04 | 3.3E+00 | 3.5E+00 |
Resource use. minerals and metals | kg Sb eq | -1.3E-01 | -7.1E-01 | -1.2E-05 | -5.1E-06 |
Rare earth element | Supply constrained? (S)a | Current situation | Market outlook (2020) | Demand constrained? (A)b | Affected process |
Lanthanum | 0 | Surplus | Surplus | 0 | Storage |
Cerium | 0 | Surplus | Surplus | 0 | Storage |
Praseodymium | 0 | Surplus | Surplus | 0 | Storage |
Neodymium | 1 | Surplus | Tight | 1 | Xunwu deposit |
Samarium | 0 | Large surplus | Large surplus | 0 | Storage |
Europium | 0 | Shortage | Shortage | 1 | Marginal user (scenarios) |
Gadolinium | 0 | Surplus | Surplus | 0 | Storage |
Terbium | 0 | Surplusc | Shortage | 1 | Marginal user (permanent magnet) |
Dysprosium | 1 | Surplus | Tight | 1 | Southeast Guangdong deposit |
Holmium | 0 | Large surplus | Large surplus | 0 | Storage |
Erbium | 0 | Surplusc | Surplus | 0 | Storage |
Thulium | 0 | Large surplus | Large surplus | 0 | Storage |
Ytterbium | 0 | Large surplus | Large surplus | 0 | Storage |
Lutetium | 0 | Large surplus | Large surplus | 0 | Storage |
Yttrium | 1 | Shortage | Shortage | 1 | Longnan deposit |
Notes: aIf S = 1, supply is unconstrained and if S = 0, supply is constrained. bIf A = 1, demand is unconstrained and if A = 0, demand is constrained. c[4] is not conclusive about the market situation of terbium and erbium. The text of the report indicates a significant surplus, while a graph shows a current shortage of both elements and a small surplus for erbium and a shortage for terbium in 2020. |
Impact category | Unit | Recycling (Scenario 1) | Recycling (Scenario 2) | Recycling (Scenario 3) | Landfilling |
Climate change | kg CO2 eq | 1.7E+03 | -1.6E+03 | 2.0E-01 | 3.3E-01 |
Ozone depletion | kg CFC11 eq | 1.8E-04 | -3.0E-05 | -1.3E-07 | 1.7E-08 |
Ionising radiation | kBq U-235 eq | -1.7E+01 | -8.0E+01 | -2.2E-01 | 2.1E-03 |
Photochemical ozone formation | kg NMVOC eq | 9.0E+00 | -1.3E+01 | -4.0E-03 | 1.6E-03 |
Particulate matter | disease inc. | 1.4E-04 | -2.4E-04 | -1.4E-07 | 2.1E-08 |
Human toxicity. non-cancer | CTUh | 9.8E-05 | -1.8E-04 | -1.1E-09 | 6.9E-09 |
Human toxicity. cancer | CTUh | 2.8E-06 | -8.8E-06 | -3.4E-10 | 1.3E-09 |
Acidification | mol H + eq | 8.2E+01 | 7.0E+01 | -1.1E-02 | 1.2E-03 |
Eutrophication. freshwater | kg P eq | 1.6E-02 | -8.5E-01 | -3.9E-04 | 1.5E-04 |
Eutrophication. marine | kg N eq | 3.6E+00 | -6.3E+00 | -3.3E-01 | 3.1E-04 |
Eutrophication. terrestrial | mol N eq | 1.9E+01 | -8.8E+01 | -7.5E-03 | 3.1E-03 |
Ecotoxicity. freshwater | CTUe | 6.6E+03 | -3.4E+05 | -7.6E+02 | 8.3E+00 |
Land use | Pt | 1.0E+05 | -4.5E+05 | -9.5E+02 | 4.0E+00 |
Water use | m3 depriv. | 4.1E+02 | -5.4E+02 | -7.0E+00 | 4.9E-02 |
Resource use. fossils | MJ | 1.7E+04 | -1.5E+04 | 3.3E+00 | 3.5E+00 |
Resource use. minerals and metals | kg Sb eq | -1.3E-01 | -7.1E-01 | -1.2E-05 | -5.1E-06 |