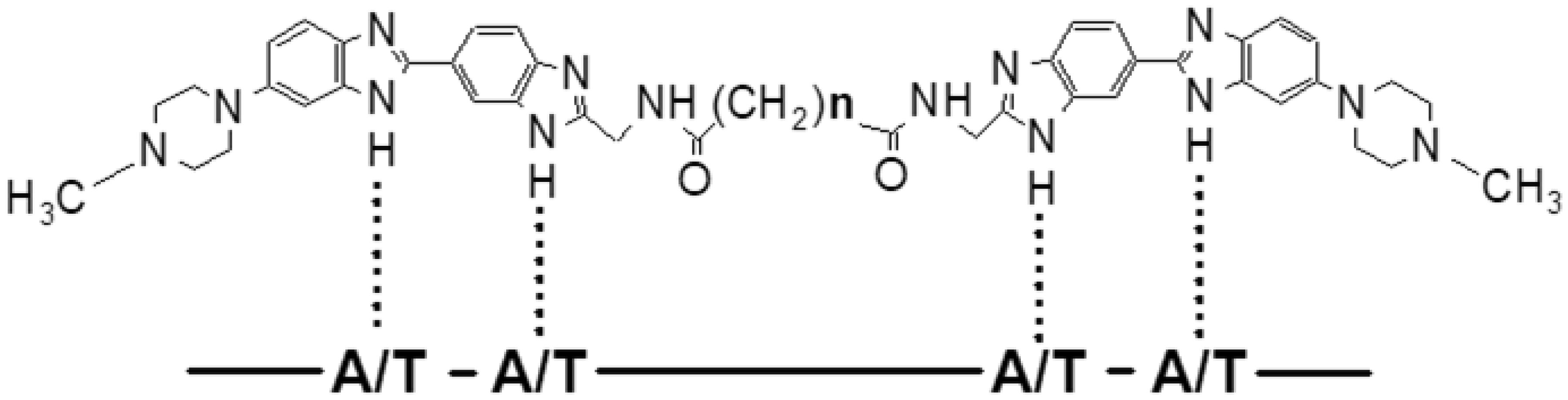
Citation: Irina A. Zamulaeva, Kristina A. Churyukina, Olga N. Matchuk, Alexander A. Ivanov, Vyacheslav O. Saburov, Alexei L. Zhuze. Dimeric bisbenzimidazoles DB(n) in combination with ionizing radiation decrease number and clonogenic activity of MCF-7 breast cancer stem cells[J]. AIMS Biophysics, 2020, 7(4): 339-361. doi: 10.3934/biophy.2020024
[1] | Francesca Ballarini, Mario P. Carante, Alessia Embriaco, Ricardo L. Ramos . Effects of ionizing radiation in biomolecules, cells and tissue/organs: basic mechanisms and applications for cancer therapy, medical imaging and radiation protection. AIMS Biophysics, 2022, 9(2): 108-112. doi: 10.3934/biophy.2022010 |
[2] | Jaouhra Cherif, Anis Raddaoui, Ghofrane Ben Fraj, Asma Laabidi, Nada Souissi . Escherichia coli's response to low-dose ionizing radiation stress. AIMS Biophysics, 2024, 11(2): 130-141. doi: 10.3934/biophy.2024009 |
[3] | Haliz Hussein, Hazhmat Ali, Zeki Mohamed, Majeed Mustafa, Khairi Abdullah, Asaad Alasady, Mayada Yalda . Thyroid function and hematological alterations in cardiac catheterization workers: a pre-post observational study on x-ray exposure. AIMS Biophysics, 2025, 12(1): 43-53. doi: 10.3934/biophy.2025004 |
[4] | Emmanuel Reyes-Uribe, Nathalia Serna-Marquez, Eduardo Perez Salazar . DDRs: receptors that mediate adhesion, migration and invasion in breast cancer cells. AIMS Biophysics, 2015, 2(3): 303-317. doi: 10.3934/biophy.2015.3.303 |
[5] | Bradley Vis, Jonathan J. Powell, Rachel E. Hewitt . Imaging flow cytometry methods for quantitative analysis of label-free crystalline silica particle interactions with immune cells. AIMS Biophysics, 2020, 7(3): 144-166. doi: 10.3934/biophy.2020012 |
[6] | Ola A Al-Ewaidat, Sopiko Gogia, Valiko Begiashvili, Moawiah M Naffaa . The multifaceted role of calcium signaling dynamics in neural cell proliferation and gliomagenesis. AIMS Biophysics, 2024, 11(3): 296-328. doi: 10.3934/biophy.2024017 |
[7] | Mikayel Ginovyan, Agnieszka Bartoszek, Izabela Koss-Mikołajczyk, Barbara Kusznierewicz, Pierre Andreoletti, Mustapha Cherkaoui-Malki, Naira Sahakyan . Growth inhibition of cultured cancer cells by Ribes nigrum leaf extract. AIMS Biophysics, 2022, 9(3): 282-293. doi: 10.3934/biophy.2022024 |
[8] | Qiao Yi Chen, Max Costa, Hong Sun . Structure and function of histone acetyltransferase MOF. AIMS Biophysics, 2015, 2(4): 555-569. doi: 10.3934/biophy.2015.4.555 |
[9] | Sweta Raikundalia, Ling Ling Few, Siti Asma' Hassan, Get Bee Yvonne-Τee, Wei Cun See Too . Choline kinase and miR-32-5p: A crucial interaction promoting apoptosis and delaying wound repair in cervical cancer cells. AIMS Biophysics, 2024, 11(3): 281-295. doi: 10.3934/biophy.2024016 |
[10] | Ahmad Sohrabi Kashani, Muthukumaran Packirisamy . Cellular deformation characterization of human breast cancer cells under hydrodynamic forces. AIMS Biophysics, 2017, 4(3): 400-414. doi: 10.3934/biophy.2017.3.400 |
Radiotherapy is widely used in clinical practice as one of the principal methods of cancer treatment. Despite permanent improvement of current methods and development of new ones (hadron, binary, tissue, photodynamic therapy, etc.) the problem of recurrences and metastases in some of the treated patients is yet to be addressed.
Numerous radioresistance mechanisms, which can provide survival of a fraction of cancer cells as a source of recurrence after radiation therapy, have been being studied from different viewpoints for several dozens of years. Recently, the radiation oncology researchers focused their attention on the concept of cancer stem cells (CSCs) [1]. This small sub-population of cancer cells, which had been found in vitro in stable and primary tumor cell cultures as well as in vivo in most malignant tumors of experimental animals and human subjects, displayed higher radio- and chemoresistance than the other cancer cells [2]–[5]. These facts, firstly, suggest that these are CSCs that determine the unfavorable long-term outcomes of radiation and combined therapy and secondly, they warrant the need for the search for compounds capable of CSC elimination and/or increase in their sensitivity towards the known anticancer agents. In this regard, it should be noted a rapidly growing number of recent studies reporting the results of search and development of such compounds not only at the stage of preclinical, but also clinical trials [6]–[8]. The targets for elimination of CSCs or modification of their properties are surface antigens of CSCs, tumor microenvironment, signal pathways, which are active predominantly in CSCs, including Hedgehog, Notch, Stat, Wnt/β-catenin, etc., cell efflux transporters, DNA repair systems, and cell death pathways [9]–[13].
Recent investigations have demonstrated the important role of epigenetic mechanisms, such as DNA methylation and histones modification, in the processes of cancer cell differentiation /dedifferentiation and maintenance of CSC resistance to anticancer agents (including ionizing radiation) [14]–[15]. In this context, compounds capable of rendering influence on chromatin remodeling and other epigenetic modifications might be of interest in finding compounds for CSC elimination and/or increasing CSC radiosensitivity. In particular, it can be surmised that such compounds include dimeric bisbenzimidazoles - DB(n), the structure of which contains two 2,6-substituted benzimidazoles connected by an oligomethylene linker of different length (n, the number of methyl groups in the linker, may vary between 1 and 11) (Figure 1) [16],[17]. In aqueous solution, DB(n) bind non-covalently in the minor groove of DNA B form occupying up to one DNA coil. Binding locations of these dimeric molecules to DNA consist of two sites (each consisting of two A-T pairs), separated by one or more pairs of any nucleotides. Earlier, we demonstrated in extracellular model systems that these compounds effectively inhibited DNA-methyltransferase and DNA-topoisomerase [18],[19].
At the first stage of this work, we investigated intracellular accumulation and subcellular localization of DB(n), where n = 1, 3, 5, 7, 9, 11, in MCF-7 breast cancer cells. Two compounds were selected: DB(5) and DB(7), which had been characterized by the highest intracellular content and nuclear localization. It was also found that both compounds possessed the ability to reduce significantly the total number and viability of cancer cell. During the second stage, we studied the influence of the selected compounds on CSCs in case of their single application or in combination with ionizing radiation. The effects were evaluated by the criteria of relative and absolute numbers of CSCs after incubation of the whole cancer cell culture with DB(5), DB(7) and irradiation, as well as by clonogenic activity of preliminarily sorted CSCs and non-CSCs subsequently treated with these compounds and irradiated. Besides, at this stage, the intracellular content of the compounds in CSCs and non-CSCs was analyzed. At the third stage, we examined inhibition of radiation-induced epithelial to mesenchymal transition (EMT) of cancer cells under the influence of DB(5) and DB(7) as a possible mechanism of the effects discovered at the second stage.
The human mammary adenocarcinoma line MCF-7 was obtained from the Russian Cell Cultures Collection of the RAS Institute of Cytology (Saint Petersburg, Russia). The cells were cultured in standard conditions in 25-cm2 flasks (Corning, USA) in Dulbecco's Modified Eagle Medium (PanEco, Russia), supplemented with 10% fetal bovine serum (FBS) (Biosera, France), penicillin (50000 U/l, PanEco, Russia), streptomycin (50 mg/l, PanEco, Russia), and glutamine (292 mg/l, PanEco, Russia) in a CO2 incubator (Shellab, USA) at + 37 °C in a humidified environment containing 5% CO2. Cells were passaged twice a week and removed from substrate with versene and trypsin solutions (PanEco, Russia).
Dimeric bisbenzimidazoles DB(n) were synthesized in Engelhardt Institute of Molecular Biology, Russian Academy of Sciences, Moscow, Russia, following the methodology detailed by Ivanov et al. [16] and Ivanov et al. [17]. The key stage in the process of DB(n) production was the synthesis of its AT-specific structural block – monomer bisbenzimidazole (MB) containing two covalently 2,6′-bound benzimidazole nuclei. MB synthesis was carried out based on commercially available p-aminobenzonitrile and 3,4-di-aminobenzonitrile produced according to Loewe and Urbanietz [20].
After the multi-step synthesis, the resultant MB was subjected to condensation reaction with α,ω-alkanedicarboxylic acids (in a molar ratio equal to 2: 1) involving formation of dimeric bisbenzimidazoles DB(1, 3, 5, 7, 9, 11), the yield being 50–78%.
Fluorescence of DB(n) complexes with double-stranded DNA was previously shown to have similar spectral characteristics [16],[17],[21]. Besides, it turned out that the obtained spectral characteristics allow using flow cytometry with UV-laser to estimate the quantity of DB(n) bound to cells. Therefore, here we carried out a comparative study of the intensity of DB(n) binding to MCF-7 cells using flow cytometry (n = 1, 3, 5, 7, 9, 11). DB(n) at the final concentration of 5, 10, 20, 30, and 40 µM were added into flasks 24 hours after cells were passaged. The cells were removed from flasks into PBS, pH 7.2, 72 hours after DB(n) addition and analyzed by FACS Vantage (Becton Dickinson - BD, USA), equipped with two lasers (488 nm and 364 nm). DB(n) fluorescence was measured in blue spectral range (424/44 nm) at λexcitation = 364 nm. The first laser was used to analyze light scattering and to select region for subsequent measuring the fluorescence.
The subcellular distribution of DB(n) was studied using confocal laser scanning microscopy after 72 hour cell incubation with the compounds. Laser confocal microscope TCS SPE 4000 (Leica Microsystems, Germany) was used for the analysis. Excitation of DB(n) fluorescence was implemented with the help of 405-nm solid laser. The fluorescence recording interval was set within 410–550 nm.
In a separate series of experiments, the intracellular content of DB(5) and DB(7) in CD44+CD24−/low CSCs and non-CSCs was examined. The whole culture was incubated with the compounds at a concentration of 20 µM for 72 hours and stained using antibodies to CD44 and CD24 labeled with FITC and phycoerythrin (PE) (BD, USA). The analysis was performed using flow cytofluorimeter FACS Vantage. Standard bandpass optical filters for 530/30 nm and 585/42 nm were applied to measure FITC and PE fluorescence, respectively, at λexcitation = 488 nm. Fluorescence intensity of DB(5) and DB(7) was assessed within a range of 424/44nm at λexcitation = 365 nm. The FITC, PE, DB(5), and DB(7) fluorescence data were recorded in a file. During subsequent data processing using CellQuestPro software (BD, USA), the region of CD44+CD24−/low cells was selected and the intensity of DB(5) or DB(7) fluorescence was analyzed separately in gated and other cells.
Cells were treated with DB(5) or DB(7) at concentrations of 5 to 60 µM in complete medium for 72 hours and their number was determined using Goryaev's chamber. Viability of cells was assessed by standard MTT-assay. The data were used to determine inhibitory concentration at 50% (IC50) and at 30% (IC30) by the criteria of the cell total number and viability.
Cells were exposed to γ-radiation at doses of 1 to 10 Gy (at a dose rate of 1.5 Gy/min) using 60Co-based therapeutic facility ‘Rokus-AM’ (Russia). The quantity of survived cells was determined 48 hours after irradiation, and the dose that had caused a reduction of this index by 30% compared to control was determined.
During the next series of experiments, the dependence of the cell survival rate on the time after exposure to γ-radiation at a dose of 4 Gy was studied. The quantity of cells that remained on the flask bottom was estimated 24, 48, 72, and 96 hours after exposure.
Cells were incubated with DB(5) or DB(7) at a final concentration of 20 µM for 24 hours under standard conditions and then exposed to γ-radiation at a dose of 4 Gy (at dose rate of 1.5 Gy/min) using ‘Rokus-AM’. After radiation exposure, incubation of cells with the compounds continued for 48 hours. Some samples were only incubated with these compounds for 72 hours; some were only exposed to radiation at the same dose and analyzed 48 hours after exposure. Intact cells served as the control.
Cells were removed from flasks into cold Hanks' solution and incubated with a mixture of CD44 monoclonal antibodies labeled with FITC (BD, USA), and CD24 antibodies labeled with PE (BD, USA) at each antibody concentration of 20 µl per 1 million cells. Isotype control antibodies to snail hemocyanin conjugated with the same fluorochromes (BD, USA) were used to evaluate nonspecific binding.
The specimens were incubated with antibodies for 30 min on ice in darkness, then washed in PBS, pH 7.2, and analyzed using FACS Vantage. Bandpass filters for 530/30 nm and 585/42 nm were used to measure fluorescence of FITC and PE, respectively. The obtained data on the intensity of forward and side light scattering, FITC and PE fluorescence were recorded in a file that was processed using CellQuestPro software (BD, USA). The relative number (%) of CD44+CD24−/low CSCs was determined among undamaged cells gated by forward and side light scattering. The absolute number of CD44+CD24−/low CSCs was established by multiplying the proportion of these cells by the total quantity of cells in a flask.
To study the clonogenic activity of CSCs and non-CSCs after a single and combined exposure, these cell populations were pre-sorted. CSCs were isolated due to their ability to efflux fluorescent dye Hoechst33342 (Ho342) from the cells and form a so-called side population (SP) in contrast to non-CSCs. After a 70% confluence had been obtained, cells were removed from the flask bottom using the versene/trypsin solution (1: 1) into serum-free medium at a dilution of 1 million cells per 1 ml of medium. Some specimens were incubated for 15 min at + 37 °C with verapamil (12.5 mg/ml) (EBEWE Pharma, Austria), which is an ATP-binding transporter blocker preventing reverse transport from cells of a number of substances including Ho342. Then cells with and without verapamil were stained with Ho342 dye (Calbiochem – Behring Corp., Germany) at a concentration of 5 µg/ml during 90 min at + 37 °C. After incubation, cells were centrifuged at 250 × g, the sediment was resuspended in cold Hanks'solution (PanEco, Russia), containing 2% FBS, 10 mM Hepes (PanEco, Russia), and 2 µg/ml propidium iodide (PI) (Sigma, USA). The samples were analyzed and sorted by FACS Vantage. Ho342 fluorescence was measured in red (675 ± 20 nm) and blue (424 ± 20 nm) spectral intervals at λexcitation of 364 nm. PI fluorescence was measured in a range of 585 ± 20 nm at λexcitation of 488 nm. SP was identified among PI.− cells which were selected after routine scatter gate procedure (Figure 2). Samples with verapamil served as negative control, in which the proportion of SP cells was significantly reduced. SP and other (non-SP) cells were sorted under sterile conditions from samples without verapamil into 6-well cultural plates (Corning, USA), 400 cells per well containing 2 ml of complete medium were plated.
DB(5) or DB(7) was added into culture medium at a concentration of 20 µM 24 hours after sorting when cells attached to the substrate and Ho342 was excluded as it was shown in preliminary experiments. After 24 hours of incubation with the compounds studied, cells were irradiated at a dose of 4 Gy using ‘Rokus-AM’ and cultured under standard conditions for 48 h; thereafter, the DB(5, 7) containing medium was removed and pure medium was added. Cells were cultured under standard conditions for 8 days; then, colonies were fixed in 96% ethanol for 10 min and stained with 0.4% trypan blue solution (w/v) at room temperature for 10 min. Colonies containing at least 50 cells were counted using light microscopy. The average number of colonies grown in the control was calculated for each population separately and taken as 100%. All colony counts in exposed samples were normalized to the sham treated control group for each cell population and clonogenic activity of exposed cells was determined as percentage of control level. The plates were set up in triplicate and independent experiments were repeated three times.
To assess radiomodifying effects, the synergism coefficient was calculated by the formula suggested by Petin and Kim [22]. At first, N – the number of damage induced by the corresponding agents in SP and non-SP cells was calculated: N= − lnS, where S is the cell survival probability (i.e. the quantity of colonies expressed as a fraction of the control level in the cell population under study) in case of a combined exposure to DB(n) and γ-radiation (comb.), exposure to DB(n) only, and exposure to γ-radiation only. Then, the synergism coefficient was calculated separately for SP and non-SP populations:
During the preliminary series of experiments, the expression of vimentin (a protein of intermediate filaments of connective tissue), which is used in cells of epithelial origin as an EMT marker, was studied at different time points after irradiation at a dose of 4 Gy. The protein expression was determined in the total cell population 24, 48, 72, and 96 hours after exposure to radiation using flow cytometry following by acetone fixation and intracellular staining with monoclonal antibodies to vimentin labeled with PE (BD Biosciences, USA) at a ratio of 20 µl antibodies/1 million cells for 1 hour. Isotype control monoclonal antibodies to snail hemocyanin, conjugated with PE (BD Biosciences, USA), were used to check nonspecific binding. Cells were washed from unbound antibodies in PBS and immediately analyzed using FACS Calibur (BD, USA) for intensity of forward and side light scattering and PE fluorescence. CellQuestPro software was used to establish the mean PE fluorescence intensity of cells after routine scatter gate procedure.
In a part of experiments, SP and non-SP were sorted onto slides 48 hours after exposure to radiation at a dose of 4 Gy. SP and non-SP cells sorted from unexposed samples were used as the control. Cells were fixed by acetone at −20 °C for 20 min, then incubated with vimentin polyclonal goat antibodies labeled with PE (Santa Cruz Biotechnology, USA), according to manufacturer's instructions, for 20 min. Thereafter, cells were washed twice from unbound antibodies in PBS, pH 7.2, and placed into MOUNT-QUICK medium (Daido Sangyo CO., LTD, Japan). Goat immunoglobulins labeled with PE (R&G Systems, USA) were used as the control of nonspecific binding. Samples were analyzed using confocal laser scanning microscope TCS SPE 4000 (Leica Microsystems, Germany) with spectral detection by a multi-channel analyzer. Solid laser with a wavelength of 488 nm was used for fluorescence excitation; fluorescence intensity was assessed within a range of 550 to 610 nm. Subsequent processing of obtained images was carried out in Imaris 7.2.3 software (Bitplane AG, Switzerland). The mean intensity of cell fluorescence per 1 pixel was determined and used to judge the level of vimentin expression. In each sample, at least 200 SP and non-SP cells were analyzed.
In the next series of experiments, the influence of DB(5), DB(7) and irradiation on vimentin expression in the total cell population was studied. The same conditions of combined exposure as in the investigation of the quantitative changes in the CSCs pool were used, i.e. the concentration of the compounds was equal to 20 µM, radiation dose of 4 Gy, and the time after irradiation (48 hours) were chosen based on the findings of the preliminary experiments described above. The methodology of specimen preparation and flow cytometry analysis in the experiments with combined exposure to DB(n) and radiation did not differ from the above-described methodology used in the preliminary experiments for examination of dependence of vimentin expression on the time after single irradiation.
Statistical data processing was carried out using the Mann-Whitney test with the help of Statistica 6.0 software (StatSoft., Inc.). Diagrams were built using Origin 6.0 software (Microcal Software, Inc.). All experiments were carried out at least in triplicate. The data were pooled. Differences were considered significant at p < 0.05.
The flow cytometry data showed that the fluorescence intensity of cells incubated with DB(3), DB(5), or DB(7) was significantly higher than that in the control for all concentrations used (Figure 3, Supplementary Figure 1). Linear dependence of fluorescence intensity on the concentration of the compounds in the culture medium was observed. The compounds penetrated into cell nuclei where they distributed unevenly with forming intensively fluorescing spots in the nucleolus areas (Figure 4). Cytoplasm was stained with the compounds relatively weakly, especially in case of DB(3).
DB(1), DB(9), and DB(11) bound to cells significantly weaker than the rest of compounds (p < 0.0001). As shown by flow cytometry, the cell fluorescence at a concentration of 20 µM and more was significantly higher than in the control without the compounds (Figure 3, p < 0.05). The intensity of nuclear fluorescence with DB(1), DB(9), and DB(11) was significantly lower compared to that after incubation with DB(3), DB(5), DB(7) (Figure 4).
Hence, DB(n) with the number of methylene groups 5 and 7 demonstrated the tightest binding to cells; they also featured the ability to penetrate into nuclei in contrast to DB(1), DB(9), and DB(11). DB(5) and DB(7) were chosen for further investigation of the effects on the whole mass of cancer cells and separately on CSCs.
The chosen compounds reduced considerably the quantity of cancer cells and their viability (Figure 5).
The average IC50 values (± SE) were approximately the same for both compounds: 35.7 ± 5.2 µM for DB(5) and 39.5 ± 5.2 µM for DB(7) according to MTT-assay after 72 hour incubation (Table 1). The 20 µM concentration corresponded approximately to IC30 (MTT-assay) and was chosen to investigate the combined effects of these compounds and radiation.
Сriteria for effect assessment | DB(5) |
DB(7) |
||
IC30, µM | IC50, µM | IC30, µM | IC50, µM | |
Total number of cells | 15.0 ± 2.2 | 29.6 ± 2.6 | 13.5 ± 1.6 | 27.5 ± 1.3 |
Cell viability (МТТ-assay) | 20.2 ± 2.3 | 35.7 ± 3.1 | 23.2 ± 1.8 | 39.5 ± 3.2 |
The radiation dose of 4 Gy corresponded to 30% inhibition of cell culture growth 48–72 hours after single irradiation compared to control (Figure 6, Figure 7). This dose was chosen for investigation of combined effects.
Thus, a scheme of further experiments to study the effects of combined exposure was developed on the base of dose effects of single exposure to DB(5), DB(7) and γ-radiation on the whole population of cancer cells. The scheme included incubation of cells in the logarithmic phase of growth with DB(5) or DB(7) at a concentration of 20 µM for 24 hours, irradiation at a dose of 4 Gy (in the same medium with the compounds), and quantification of CSCs 48 hours after irradiation (correspondingly, 72 hours after addition of the compounds). Irradiation (4 Gy) was also performed 24 hours after addition of the compounds (20 µM) to study the clonogenic activity of sorted CSCs and non-CSCs, but the experiment was terminated 8 days after irradiation when the grown colonies were fixed, stained, and counted.
The intracellular content of DB(5) and DB(7) was studied in the cancer cell populations selected by immunophenotype 72 hours after incubation with the compounds, i.e. after a rather long period during which both accumulation and efflux of the compounds from live cells could occur. As Table 2 shows, the fluorescence intensity of both DB(5) and DB(7) in CSCs and non-CSCs was approximately equal, but considerably higher than the control autofluorescence (p < 0.001). The findings indicate that these compounds are capable of penetrating into live cells and remaining therein for a long time. Importantly, the content of DB(5) and DB(7) in CSCs and non-CSCs did not differ, though it is known that CSCs are capable of transporting many chemical compounds including conventional chemotherapeutic agents into extracellular environment more effectively. In future, it would be promising to study the processes of DB (n) accumulation and efflux separately for these cancer cell populations.
Mean fluorescence intensity ± SE, relative units |
||
CSCs | Non-CSCs | |
Control autofluorescence | 20.0 ± 3.6 | 10.2 ± 1.4 |
DB(5) | 95.3 ± 14.4*^ | 92.3 ± 4.9* |
DB(7) | 102.8 ± 10.9*^ | 109.2 ± 10.5* |
*p < 0.001 in comparison with corresponding control autofluorescence; ^p > 0.90 in comparison with non-CSCs incubated with the corresponding compound
The effects of a single and combined exposure to the compounds and γ-radiation were evaluated by the change in the number of cells with CD44+CD24 −/low immunophenotype which is frequently used to detect CSCs in breast cancer derived cell cultures including MCF-7 line (Supplementary Figure 2).
Exposure of MCF-7 cells to radiation was found to increase the relative number of CD44+CD24−/low CSCs by a factor of 1.7 compared to the control (p = 0.04, Figure 8). Pre-incubation with DB(5) or DB(7) prior to irradiation significantly decreased this index compared to both single irradiation and control. It has been shown that DB(5) combined with ionizing radiation reduces the relative number of CD44+CD24−/low cells by a factor of 4.8, DB(7) by a factor of 2.4 compared to the control (p = 0.002 and p = 0.006, respectively). Importantly, DB(5) and DB(7) in combination with ionizing radiation reduced the relative number of CSCs compared to single irradiation even greater: 8.2-fold and 4.1-fold (p = 0.003 and p = 0.004, respectively), thus abolishing the stimulating effect of radiation on CSCs population.
Interestingly, a single exposure to DB(5) or DB(7) also reduced significantly the relative number of CSCs compared to control by 4.9-fold (p = 0.001) and 3.7-fold (p = 0.003), respectively.
Figure 9 presents the changes in absolute CD44+CD24−/low cell numbers after a single and combined exposure to the compounds and γ-radiation. Irradiation was shown to result in 1.6-fold increase in the absolute CSC number vs the control (p = 0.008), while the quantity of non-CSCs, on the contrary, decreased by a factor of 1.7 (p = 0.003). We established that both DB(5) and DB(7) in combination with ionizing radiation significantly reduced the absolute CD44+CD24−/low cell number compared to single irradiation and to the control. In combination with irradiation, DB(5) led to a 10.4-fold decrease in the absolute number of CSCs compared to the control (p = 0.004) and a 16.6-fold decrease compared to irradiation (p = 0.006), DB(7) led to a 8.9-fold decline compared to the control (p = 0.004) and a 14.1-fold decrease compared to irradiation (p = 0.006). At the same time, the quantity of non-CSCs also decreases after combined exposure to DB(5) or DB(7) and irradiation, but to a lesser degree – by a factor of 2.4 and 3.8, respectively, compared to the control (p = 0.004 in both cases), and by 1.4-fold and 2.2-fold, respectively, compared to irradiation (p = 0.04 and p = 0.006, respectively).
A single exposure to DB(5) and DB(7) also yielded a 7.5-fold and a 5.2-fold decrease of the absolute numbers of CD44+CD24−/low CSCs compared to control (p = 0.001 and p = 0.003, respectively). The absolute quantity of non-CSCs decreased only by a factor of 1.8 and 3.3 (p = 0.001 and p = 0.004), respectively.
The data on the clonogenic activity (reproductive survival) of SP and non-SP cells after combined exposure to ionizing radiation and DB(5) or DB(7) are given in Figure 10. The single exposure to radiation was shown to decrease the clonogenic activity of SP cells merely by 18% vs control (p = 0.02), while the clonogenic activity of non-SP cells decreased to a greater degree, by 33% (p = 0.003 vs control). These findings corroborate the published reports on higher radioresistance of CSCs compared to other cells. We established that DB(5) and DB(7) combined with irradiation significantly decreased the clonogenic activity of SP cells compared to the single irradiation (p = 0.0003 and p = 0.005, respectively). The effects of combined exposure to DB(n) and radiation on SP cells were synergistic: the synergism coefficient was 1.3 in case of DB(5) and 1.2 in case of DB(7). The combined exposure of non-SP cells to DB(n) and radiation reduced the colony count to a lesser degree and in additive or subadditive manner: the synergism coefficient was equal to 1.0 for DB(5) and 0.7 for DB(7).
The preliminary experiments with MCF-7 cells confirmed an interrelation between EMT and stemness of cancer cells, which was demonstrated earlier by other authors [23]–[25]. As shown by confocal laser scanning microscopy, mean intensity of fluorescence with vimentin antibodies was significantly higher in sorted CSCs (SP) than that in non-CSCs, both in control unexposed and in irradiated samples (Figure 11). In the unexposed samples, weak expression of vimentin was found only in SP cells while fluorescence of non-SP cells corresponded to background fluorescence. It should be noted that the mean proportion (± SE) of sorted SP cells among the whole population of cancer cells was 1.8 ± 0.5% in the control and 7.5 ± 1.0% after radiation exposure at a dose of 4 Gy.
As shown by flow cytometry on the whole (unsorted) MCF-7 culture, the fluorescence intensity of unirradiated cells after incubation with vimentin antibodies did not differ from that after incubation with control antibodies of the same isotype (Figure 12). However, specific binding of vimentin antibodies was discovered 48–96 hours after irradiation at 4 Gy. These data suggest induction of expression of this protein, an EMT marker, under the influence of γ-radiation. Thus, we established that not only the above-mentioned elevation in CSC proportion detectable by two independent methods, but also a radiation-induced EMT can be registered 48 hours after irradiation. Therefore this time point was chosen for further investigation of combined effects of DB(5), DB(7) and ionizing radiation on EMT based on the criterion of protein expression of vimentin. Notably, vimentin expression is not yet detected in the cell culture 24 hours after irradiation, and similarly, no increase in the CSC number can be found at this time point (the data are not shown).
Incubation of cells with DB(5) or DB(7) blocked radiation EMT (Table 3, Figure 13), and simultaneously caused a decrease in CSC fraction below the baseline (control level).
Mean ± SE | |
Control | 14.0 ± 0.6 |
DB(5) | 10.8 ± 0.9 |
DB(7) | 13.3 ± 1.2 |
4 Gy | 17.2 ± 1.0 a,b |
DB(5) + 4 Gy | 12.8 ± 1.0c |
DB(7) + 4 Gy | 12.8 ± 0.4d |
Background fluorescence (nonspecific binding of control antibodies) | 12.3 ± 1.0 |
ap = 0.03 in comparison with control; bp = 0.003 in comparison with background fluorescence; cp = 0.01 in comparison with 4Gy; dp = 0.003 in comparison with 4Gy.
The studied compounds are dimer derivatives of bisbenzimidazoles, which are capable of rendering both radioprotective and radiosensitizing effect as it was shown in different model systems by the example of Hoechst33258 and Hoechst33342. In particular, on the one hand, early investigations revealed an ability of the latter to quench radiation-induced oxidative radicals on DNA and decrease the number of DNA breaks after radiation exposure, which resulted in elevated radioresistance of healthy tissues and higher survival rate of animals exposed [26]–[30]. On the other hand, bisbenzimidazoles and their derivatives have been shown to possess the ability of inhibiting a number of enzymes (topoisomerases I and II, DNA-methyltransferases, poly (ADP-ribose) polymerase I – PARP), which play an important role in chromatin remodeling and repair of damaged DNA [18],[19],[31]–[33]. As the cancer cell, unlike the normal one, is characterized by higher contents of DNA-topoisomerases [34],[35], inhibition of the latter's activity under the influence of bisbenzimidazoles might have prevalent significance specifically for cancer cells and finally result in the increase of their radiosensitivity, as reported by a number of articles [36],[37]. Besides, inhibition of PARP leads also to increase in radiation-induced death of cancer cells while radiosensitivity of normal cells might remain unmodified. This effect is especially pronounced in tumors displaying a deficit in the repair of double-stranded DNA breaks due to BRCA1 and BRCA2 mutations [38],[39].
Considering literature data, we supposed that dimer derivatives of bisbenzimidazoles might be quite attractive for radiation therapy as radiomodifiers that are capable of raising cancer cells' sensitivity to ionizing radiation concurrently protecting normal tissues. In this paper, the ability of chosen DB(n) to affect the quantity and clonogenic activity of the resistant fraction of cancer cells (CSCs) was studied for single and combined usage of the compounds and ionizing radiation.
The first stage of the study showed that the ability of DB(n) to accumulate in MCF-7 cells and bind to cell nuclei depended on the length of methylene linker, where n = 1, 3, 5, 7, 9, 11. DB(3), DB(5), and DB(7) were found to accumulate intensively in the nuclei, mostly in the area of nucleoli. Such distribution might be explained by interaction of dimer bisbenzimidazoles with AT-rich regions of heterochromatin surrounding the nucleolus. It is not implausible that these compounds interact with negatively charged proteins of the nucleolus and ribonucleproteide complexes. The highest fluorescence of cells was demonstrated for DB(5) and DB(7), which were chosen to study further their influence on CSCs. At this stage DB(5) and DB(7) were also found to suppress growth of the cell culture. This effect seems to be related to the ability of compounds chosen to inhibit the activity of topoisomerases I. This hypothesis is based on the findings of Susova et al. who showed that DB (3, 5, 7) inhibited binding of topoisomerases I to isolated eukaryotic DNA, wherein, for instance, DB(7) did this dozen times more effectively than camptothecin that is the known inhibitor of this enzyme and successfully used as an anticancer drug [19].
One of the most important properties of CSCs is high rate of efflux of many chemical compounds, including anticancer drugs, due to high expression of respective ATP–binding transporters on the cellular membrane [40]. We have shown that the intracellular content of DB(n), where n = 5 or 7, is approximately equal in CSCs and the other cells in contrast to many known chemotherapy agents. These basic facts warrant selection of these compounds to study their anticancer and radiomodifying effects on CSCs.
The present study demonstrated that single irradiation resulted in elevation of CSC fraction identified by CD44+CD24−/low immunophenotype. These findings supporting higher resistance of CSCs to low-LET ionizing radiation (compared to the bulk of cancer cells) are consistent with the results of numerous studies that used various approaches to identification of breast CSCs both in experimental and clinical conditions [3],[5],[23],[24],[41]–[43].
Within recent years, one more mechanism of increase in the CSC quantity after an exposure to low-LET ionizing radiation is discussed. This is reprogramming of differentiated cancer cells surviving after irradiation into CSCs [24],[42],[44]. The process of dedifferentiation might explain the increase in not only relative but also absolute number of CSCs after an exposure to radiation – the effect we observed in case of CSC identification by immunophenotype in this study and by intensive efflux of fluorescent dye Ho342 earlier [45]. Such dedifferentiation is evidently a rather rare event in intact cancers or cell cultures, but may occur more frequently under the influence of various factors including ionizing radiation. Studying the mechanisms of this phenomenon was recently performed in many laboratories worldwide and resulted in clarification of the role of EMT in the maintenance and even increase in the CSC pool after irradiation by dedifferentiation of non-stem cells [39],[41],[45]. It is well known that an exposure to radiation can induce EMT of cancer cells of epithelial origin, which has been proven in this paper by the example of MCF-7 breast cancer. An increased rate of vimentin expression (one of the main markers of EMT) was shown 48 hours after irradiation and at later time points tested in the study (up to 96 hours).
There is a growing body of data on a possibility of inhibiting EMT with different agents, among which various modifiers of chromatin structure turned out rather effective [46]–[48]. Cell culture experiments have shown that EMT blockers reduce the quantity of CSCs, hence, their use is considered very promising in a combination with conventional anticancer impacts [49],[50].
It should be noted that the reprogramming process involves epigenetic mechanisms such as modification of DNA methylation [51]. DNA methylation has been shown to participate directly in cell migration, invasion, metastasizing and EMT of cancer cells and is also an important regulatory mechanism for maintaining the key features of CSCs [14],[15],[52]. It would be logical to assume that DB(n) which possess DNA-methyltransferase inhibiting activity are capable of rendering influence on amount and properties of CSCs by blocking EMT. In fact, an absence of vimentin expression was demonstrated after combined exposure to DB(n) (where n = 5 or 7) and ionizing radiation; that is evidence for blocking the radiation-induced EMT. Importantly, this was predictably accompanied with decrease in the relative and absolute numbers of CSCs. Interestingly, cell incubation with DB(5) or DB(7) itself resulted in decreased CSC count as compared with not only irradiation but also control. In the latter case, the effects of the compounds were hypothetically attributed to inhibition of spontaneous EMT that occurs rarely and involves few cancer cells causing their dediffentiation into CD44+CD24−/low CSCs accounting for mere 0.29% in the control. It is quite explicable that EMT was impossible to be registered in the whole cell mass of intact MCF-7 culture because this process occurs only in a minor fraction of control cells as we have shown for sorted SP and non-SP cells with laser scanning microscopy.
Importantly, DB(5) and DB(7) not only reduced the CSC number shortly after exposure but also decreased clonogenic activity of cancer cells. In combination with irradiation, a synergistic decline of the clonogenic activity of CSCs (SP) occurred whereas the effects of decreasing the clonogenic activity of non-CSCs (non-SP) were of an additive and subadditive nature. Thus, we demonstrated that DB(n), where n = 5 or 7, rendered a radiosensitizing effect specifically on CSCs.
On the whole, our findings are consistent with a number of experimental studies showing that DNA-methylation inhibitors raise the efficacy of radiation impacts on cancer cells both in vitro and in vivo [51],[53]. In this instance, the expression of genes controlling maintenance of CSCs in the status of dedifferentiation is down-regulated significantly, as has been shown by the example of pancreatic cancer [53].
Thus, the intracellular content of synthetic DB(n), where n = 5 or 7, has proven to be similar in cancer stem and non-stem cells. Both compounds were capable of abolishing the stimulating effect of γ-radiation on CSC population, revealed as an increase in the relative and absolute numbers of these cells. Inhibition of radiation-induced EMT and significant reduction of CSCs pool were found after combined action of the compounds and radiation. The combined effects on clonogenic activity were synergistic for CSCs and additive or subadditive for non-CSCs. These effects may be due to inhibitory action of the compounds on DNA-methylation of a number of genes controlling dedifferentiation of cancer cells and CSC properties. However, further research is needed to test this assumption. The findings suggest a promising outlook of further investigation of DB(5) and DB(7) in vivo as potential agents capable of reducing the pool of CSCs in the course of radiation therapy of malignant neoplasms.
[1] | Peitzch C, Kurth I, Ebert N, et al. (2019) Cancer stem cells in radiation response: current views and future perspectives in radiation oncology. Int J Radiat Biol 95: 900-911. |
[2] | Zhu P, Fan Z (2018) Cancer stem cells and tumorigenesis. Biophys Rep 4: 178-188. |
[3] | Lytle NK, Barber AG, Reya T (2018) Stem cells fate in cancer growth, progression and therapy resistance. Nat Rev Cancer 18: 669-680. |
[4] | Battle E, Clevers H (2017) Cancer stem cells revisited. Nat Med 23: 1124-1134. |
[5] | Matchuk ON, Zamulaeva IA, Selivanova EI, et al. (2012) Sensitivity of melanoma B16 side population to low- and high-LET radiation. Radiats Biol Radioecol 52: 261-267. |
[6] | Kaiser J (2015) The cancer stem cell gamble. Science 347: 226-229. |
[7] | Ahmed M, Chaudhari K, Babaeli-Jadidi R, et al. (2017) Concise review: Emerging drugs targeting epithelial cancer stem-like cells. Stem Celsl 35: 839-850. |
[8] | Desai A, Yan Y, Gerson SL (2019) Concise reviews: Cancer stem cell targeted therapies: toward clinical success. Stem Cells Transl Med 8: 75-81. |
[9] | Kim YJ, Siegler EL, Siriwon N, et al. (2016) Therapeutic strategies for targeting cancer stem cells. J Cancer Metastasis Treat 2: 233-242. |
[10] | Pan Y, Ma S, Cao K, et al. (2018) Therapeutic approaches targeting cancer stem cells. J Cancer Res Ther 14: 1469-1475. |
[11] | Dominik Kuhlmann J, Hein L, Kurth I, et al. (2016) Targeting cancer stem cells: Promises and challenges. Anticancer Agents Med Chem 16: 38-58. |
[12] | Nunes T, Hamdan D, Leboeuf C, et al. (2018) Targeting cancer stem cells to overcome chemoresistance. Int J Mol Sci 19: e4036. |
[13] | Phi LTH, Sari IN, Yang YG, et al. (2018) Cancer stem cells (CSCs) in drug resistance and their therapeutic implications in cancer treatment. Stem Cells Int 2018: 5416923. |
[14] | Wainwright EN, Scaffidi P (2017) Epigenetics and cancer stem cells: Unleashing hijacking, and restricting cellular plasticity. Trends Cancer 3: 372-386. |
[15] | Toh TB, Lim JJ, Chow EK (2017) Epigenetics in cancer stem cells. Mol Cancer 16: 29. |
[16] | Ivanov AA, Salyanov VI, Strel'tsov SA, et al. (2011) DNA sequence-specific ligands: XIV. Synthesis of fluorescent biologicaly active dimeric bisbenzimidazoles-DB (3, 4, 5, 7, 11). Russ J Bioorgan Chem 37: 472-482. |
[17] | Ivanov AA, Salyanov VI, Zhuze AL (2016) DNA sequence specific ligands: XV. synthesis and spectral characteristics of a new series of dimeric bisbenzimidazoles DB(1, 2, 6, 8, 9, 10, 12). Russ J Bioorgan Chem 42: 183-190. |
[18] | Cherepanova NA, Ivanov AA, Maltseva DV, et al. (2011) Dimeric bisbenzimidazoles inhibit the DNA methylation catalyzed by the Dnmt3a catalytic domain. J Ensyme Inhib Med Chem 26: 295-300. |
[19] | Susova OY, Ivanov AA, Ruiz SSM, et al. (2010) Minor groove dimeric bisbenzimidazoles inhibit in vitro DNA binding to eukaryotic DNA topoisomerase I. Biochemistry (Mosc) 75: 695-701. |
[20] | Loewe H, Urbanietz J (1974) Basic-substituted 2,6-bisbenzimidazole derivates, a novel class of substances with chemotherapeutic activity. Arzneimittel-forschung 24: 1927-1933. |
[21] | Popov KV, Egorova EI, Ivanov AA, et al. (2008) Dimeric bisbenzimidazole Hoechst 33258-related dyes as novel AT-specific DNA-binding fluorochromes for human and plant cytogenetics. Biochem (Mosc) Suppl Ser A: Membr Cell Biol 2: 203-209. |
[22] | Petin VG, Kim JK (2016) Synergistic Interaction and Cell Responses to Environmental Factors New York: Nova Science Publishers, 100-101. |
[23] | Lee SY, Jeong EK, Ju MK, et al. (2017) Induction of metastasis, cancer stem cell phenotype, and oncogenic metabolism in cancer cells by ionizing radiation. Mol Cancer 16: 10. |
[24] | Li F, Zhou K, Gao L, et al. (2016) Radiation induced the generation of cancer stem cells: A novel mechanism for cancer radioresistance. Oncol Lett 12: 3059-3065. |
[25] | Chi HC, Tsai CY, Tsai MM, et al. (2017) Roles of long noncoding RNAs in recurrence and metastasis of radiotherapy-resistant cancer stem cells. Int J Mol Sci 18: e1903. |
[26] | Lyubimova NV, Coultas PG, Yuen K, et al. (2001) In vivo radioprotection of mouse brain endothelial cells by Hoechst 33342. Br J Radiol 74: 77-82. |
[27] | Lobachevsky P, Ivashkevich A, Martin OA, et al. (2011) DNA-binding radioprotectors. Selected Topics in DNA Repair London: IntechOpen, 497-519. |
[28] | Martin RF, Broadhurst S, Reum ME, et al. (2004) In vitro studies with methylproamine: A potent new radioprotector. Cancer Res 64: 1067-1070. |
[29] | Tawar U, Jain AK, Dwarakanath BS, et al. (2003) Influence of phenyl ring disubstitution on bisbenzimidazole and terbenzimidazole cytotoxicity: synthesis and biological evaluation as radioprotectors. J Med Chem 46: 3785-3792. |
[30] | Martin RF, Denison L (1992) DNA ligands as radiomodifiers: studies with minor-groove binding bibenzimidazoles. Int J Rad Oncol Biol Phys 23: 579-584. |
[31] | Kim JS, Sun Q, Yu C, et al. (1998) Quantitative structure-activity relationships on 5-substituted terbenzimidazoles as topoisomerase I poisons and antitumor agents. Bioorg Med Chem 6: 163-172. |
[32] | Shunkwiler L, Ferris G, Kunos C (2013) Inhibition of poly(ADP-Ribose) polymerase enhances radiochemosensitivity in cancers proficient in DNA double-strand break repair. Int J Mol Sci 14: 3773-3785. |
[33] | Sun Q, Gatto B, Yu C, et al. (1994) Structure activity of topoisomerase I poisons related to Hoechst 333342. Bioorg Med Chem Lett 4: 2871-2876. |
[34] | Parchment RE, Pessina A (1998) Topoisomerase I inhibitors and drug resistance. Cytotechnology 27: 149-164. |
[35] | Berney DM, Shamash J, Gaffney J, et al. (2002) DNA topoisomerase I and II expression in drug resistant germ cell tumours. Br J Cancer 87: 624-629. |
[36] | Dwarakanath BS, Singh S, Jain V (1999) Optimization of tumor radiotherapy: Part V-radiosensitization by 2-deoxy-D-glucose and DNA ligand hoechest-33342 in a murine tumor. Indian J Exp Biol 37: 865-870. |
[37] | Adhikari JS, Khaitan D, Arya MB, et al. (2005) Heterogeneity in the radiosensitizing effects of the DNA ligands hoechst-33342 in human tumor cell lines. J Cancer Res Ther 1: 151-161. |
[38] | Wera AC, Lobbens A, Stoyanov M, et al. (2019) Radiation-induced synthetic lethality: combination of poly(ADP-ribose) polymerase and RAD51 inhibitors to sensitize cells to proton irradiation. Cell Cycle 18: 1770-1783. |
[39] | Ashworth A (2008) A synthetic lethal therapeutic approach: Poly(ADP) ribose polymerase inhibitors for the treatment of cancers deficient in DNA double-strand break repair. J Clin Oncol 26: 3785-3790. |
[40] | Begicevic RR, Falasca M (2017) ABC transporters in cancer stem cells: beyond chemoresistance. Int J Mol Sci 18: e2362. |
[41] | Krause M, Dubrovska A, Linge A, et al. (2017) Cancer stem cells: Radioresistance, prediction of radiotherapy outcome and specific targets for combined treatments. Adv Drug Deliv Rev 109: 63-73. |
[42] | Lagadec C, Vlashi E, Donna LD, et al. (2012) Radiation-induced reprogramming of breast cancer cells. Stem Cells 30: 833-844. |
[43] | Zamulaeva IA, Matchuk ON, Selivanova EI, et al. (2014) Increase in the number of cancer stem cells after exposure to low-LET radiation. Radiats Biol Radioecol 54: 256-264. |
[44] | Murata K, Saga R, Monzen S, et al. (2019) Understanding the mechanism underlying the acquisition of radioresistance in human prostate cancer cells. Oncol Lett 17: 5830-5838. |
[45] | Matchuk ON, Zamulaeva IA (2019) Quantitative changes in the stem cell population of cervical cancer cell line HeLa under the influence of fractionated γ-irradiation in vitro. Radiation and Risk 28: 112-123. |
[46] | Boulding T, McCuaig RD, Tan A, et al. (2018) LSD1 activation promotes inducible EMT programs and modulates the tumour microenvironment in breast cancer. Sci Rep 8: 73. |
[47] | Ambrosio S, Sacca CD, Majello B (2017) Epigenetic regulation of epithelial to mesenchymal transition by the Lysine-specific demethylase LSD1/KDM1A. Biochim Biophys Acta Gene Regul Mech 1860: 905-910. |
[48] | Kanamoto A, Ninomiya I, Harada S, et al. (2016) Valproic acid inhibits irradiation-induced epithelial-mesenchymal transition and stem cell-like characteristics in esophageal squamous cell carcinoma. Int J Oncol 49: 1859-1869. |
[49] | Garg M (2017) Epithelial plasticity and cancer stem cells: Major mechanisms of cancer pathogenesis and therapy resistance. World J Stem Cells 9: 118-126. |
[50] | Kotiyal S, Bhattacharya S (2014) Breast cancer stem cells, EMT and therapeutic targets. Biochem Biophys Res Commun 453: 112-116. |
[51] | Miousse IR, Kutanzi KR, Koturbash I (2017) Effects of ionizing radiation on DNA methylation: from experimental biology to clinical applications. Int J Radiat Biol 93: 457-469. |
[52] | Luczak MW, Jagodzinski PP (2006) The role of DNA methylation in cancer development. Folia Histochem Cytobiol 44: 143-154. |
[53] | Kwon HM, Kang EJ, Kang K, et al. (2017) Combinatorial effects of an epigenetic inhibitor and ionizing radiation contribute to targeted elimination of pancreatic cancer stem cell. Oncotarget 8: 89005-89020. |
1. | K. A Churiukina, O. N Matchuk, A. D Kaprin, S. A Ivanov, V. S Koval, A. F Arutyunyan, A. L Zhuze, I. A Zamulaeva, Effects of DNA-binding ligands from dimeric bisbenzimidazoles series DBA(n) and DBPA( n) in combination with γ-radiation on epithelial-mesenchymal transition and pool size of MCF-7 breast cancer stem cells, 2023, 68, 0006-3029, 529, 10.31857/S0006302923030158 | |
2. | K. A. Churiukina, O. N. Matchuk, A. D. Kaprin, S. A. Ivanov, V. S. Koval, A. F. Arutyunyan, A. L. Zhuze, I. A. Zamulaeva, The Effect of DNA-Binding Ligands from Dimeric Bisbenzimidazoles of the DBA(n) and DBPA(n) Series in Combination with γ-Radiation on Epithelial–Mesenchymal Transition and Pool Size of MCF-7 Breast Cancer Stem Cells, 2023, 68, 0006-3509, 422, 10.1134/S0006350923030065 |
Сriteria for effect assessment | DB(5) |
DB(7) |
||
IC30, µM | IC50, µM | IC30, µM | IC50, µM | |
Total number of cells | 15.0 ± 2.2 | 29.6 ± 2.6 | 13.5 ± 1.6 | 27.5 ± 1.3 |
Cell viability (МТТ-assay) | 20.2 ± 2.3 | 35.7 ± 3.1 | 23.2 ± 1.8 | 39.5 ± 3.2 |
Mean fluorescence intensity ± SE, relative units |
||
CSCs | Non-CSCs | |
Control autofluorescence | 20.0 ± 3.6 | 10.2 ± 1.4 |
DB(5) | 95.3 ± 14.4*^ | 92.3 ± 4.9* |
DB(7) | 102.8 ± 10.9*^ | 109.2 ± 10.5* |
*p < 0.001 in comparison with corresponding control autofluorescence; ^p > 0.90 in comparison with non-CSCs incubated with the corresponding compound
Mean ± SE | |
Control | 14.0 ± 0.6 |
DB(5) | 10.8 ± 0.9 |
DB(7) | 13.3 ± 1.2 |
4 Gy | 17.2 ± 1.0 a,b |
DB(5) + 4 Gy | 12.8 ± 1.0c |
DB(7) + 4 Gy | 12.8 ± 0.4d |
Background fluorescence (nonspecific binding of control antibodies) | 12.3 ± 1.0 |
ap = 0.03 in comparison with control; bp = 0.003 in comparison with background fluorescence; cp = 0.01 in comparison with 4Gy; dp = 0.003 in comparison with 4Gy.
Сriteria for effect assessment | DB(5) |
DB(7) |
||
IC30, µM | IC50, µM | IC30, µM | IC50, µM | |
Total number of cells | 15.0 ± 2.2 | 29.6 ± 2.6 | 13.5 ± 1.6 | 27.5 ± 1.3 |
Cell viability (МТТ-assay) | 20.2 ± 2.3 | 35.7 ± 3.1 | 23.2 ± 1.8 | 39.5 ± 3.2 |
Mean fluorescence intensity ± SE, relative units |
||
CSCs | Non-CSCs | |
Control autofluorescence | 20.0 ± 3.6 | 10.2 ± 1.4 |
DB(5) | 95.3 ± 14.4*^ | 92.3 ± 4.9* |
DB(7) | 102.8 ± 10.9*^ | 109.2 ± 10.5* |
Mean ± SE | |
Control | 14.0 ± 0.6 |
DB(5) | 10.8 ± 0.9 |
DB(7) | 13.3 ± 1.2 |
4 Gy | 17.2 ± 1.0 a,b |
DB(5) + 4 Gy | 12.8 ± 1.0c |
DB(7) + 4 Gy | 12.8 ± 0.4d |
Background fluorescence (nonspecific binding of control antibodies) | 12.3 ± 1.0 |