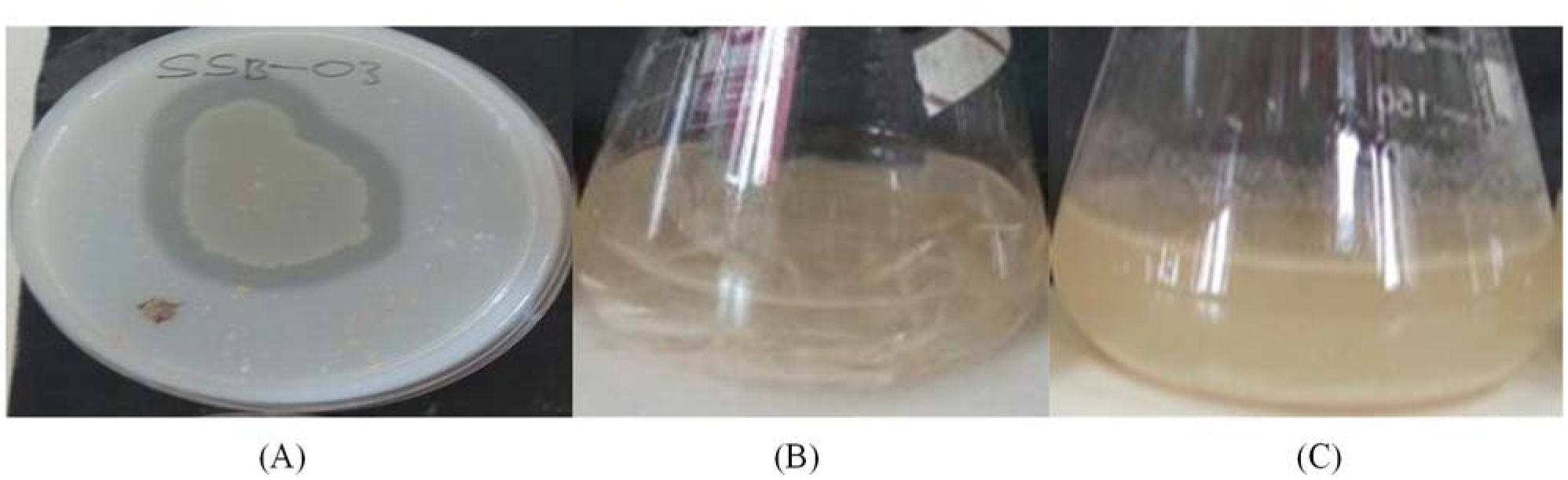
The transition from a traditional linear economy to a circular model aims to create a more sustainable future by reducing the adverse effects of agro-waste on the environment. The present study evaluated the metabolic diversity of bacterial isolates from municipal dumpsites for keratinase production and poultry feather valorization. The bacterium with the highest keratinolytic activity was identified through 16S ribosomal ribonucleic acid (rRNA) gene sequencing. The exo-keratinase production by the bacterium was optimized, and the feather hydrolysate obtained from the fermentation process was analyzed for amino acids. Among the twelve bacteria isolated from the dumpsite's sample, three showed significant feather degradation and keratinase production of 89% (744.5 ± 9.19 U/mL), 58% (269 ± 15.55 U/mL), and 46% (195 ± 7.07 U/mL) for SSB-03, SSB-02, and HSB-02, respectively. Analysis of the 16S rRNA gene sequence revealed that SSB-03 has high sequence homology with Exiguobacterium acetylicum, and thus, it was identified as Exiguobacterium acetylicum FHBD (accession number MW165834). Strain FHBD fermentation medium exhibited the maximum keratinase activity (2934.54 ± 38.56 U/mL) and sulfhydryl group content (3.09 ± 0.02 mM) at 72 h under optimal process conditions of pH 5.0, temperature (35 °C), inoculum size (2% v/v), and feather (15 g/L). Amino acid analysis of the feather hydrolysate showed significant levels of glutamic acid, aspartic acid, glycine, arginine, serine, and proline, with respective concentrations of 1.58, 1.34, 1.29, 1.20, 1.12, and 0.93 (g/100 g of sample). The study's findings emphasize the potential of E. acetylicum FHBD in poultry feather valorization and keratinase production.
Citation: Tutuka Dlume, Nonso E. Nnolim, Uchechukwu U. Nwodo. Exiguobacterium acetylicum transformed poultry feathers into amino acids through an extracellular secretion of keratinolytic enzymes[J]. AIMS Bioengineering, 2024, 11(4): 489-505. doi: 10.3934/bioeng.2024022
[1] | Zongyuan Zhu, Rachael Simister, Susannah Bird, Simon J. McQueen-Mason, Leonardo D. Gomez, Duncan J. Macquarrie . Microwave assisted acid and alkali pretreatment of Miscanthus biomass for biorefineries. AIMS Bioengineering, 2015, 2(4): 449-468. doi: 10.3934/bioeng.2015.4.449 |
[2] | Nikolaos Giormezis, Konstantinos Papakonstantinou, Fevronia Kolonitsiou, Eleanna Drougka, Antigoni Foka, Styliani Sarrou, Evangelos D. Anastassiou, Efthimia Petinaki, Iris Spiliopoulou . Biofilm synthesis and its relationship with genetic characteristics in clinical methicillin-resistant staphylococci. AIMS Bioengineering, 2015, 2(4): 375-386. doi: 10.3934/bioeng.2015.4.375 |
[3] | Urooj Ainuddin, Maria Waqas . Finite state machine and Markovian equivalents of the lac Operon in E. coli bacterium. AIMS Bioengineering, 2022, 9(4): 400-419. doi: 10.3934/bioeng.2022029 |
[4] | Khalil Essani, Anton Glieder, Martina Geier . Combinatorial pathway assembly in yeast. AIMS Bioengineering, 2015, 2(4): 423-436. doi: 10.3934/bioeng.2015.4.423 |
[5] | Flávia da Silva Fernandes, Luan Reis Honorato da Silva, Érica Simplício de Souza, Lívia Melo Carneiro, João Paulo Alves Silva, Steven Zelski, João Vicente Braga de Souza, Jacqueline da Silva Batista . Isolation, genetic identification of Amazonian yeasts and analysis of thermotolerance and alcohol tolerance of Saccharomyces cerevisiae from Theobroma grandiflorum and Eugenia stipitata. AIMS Bioengineering, 2024, 11(1): 24-43. doi: 10.3934/bioeng.2024003 |
[6] | Wagner C. A. Carvalho, Rayane A. S. Freitas, Milson S. Barbosa, Ariela V. Paula, Ernandes B. Pereira, Adriano A. Mendes, Elton Franceschi, Cleide M. F. Soares . Preparation and characterization of new silica-based heterofunctional biocatalysts utilizing low-cost lipase Eversa® Transform 2.0 and evaluation of their catalytic performance in isoamyl esters production from Moringa oleifera Lam oil. AIMS Bioengineering, 2024, 11(2): 185-211. doi: 10.3934/bioeng.2024011 |
[7] | Clara Navarrete, José L. Martínez . Non-conventional yeasts as superior production platforms for sustainable fermentation based bio-manufacturing processes. AIMS Bioengineering, 2020, 7(4): 289-305. doi: 10.3934/bioeng.2020024 |
[8] | Olga M. Tsivileva, Inna M. Uchaeva, Nikolay A. Yurasov . Biotesting of technologically important carboxy containing acridones with solid-state fungal culture. AIMS Bioengineering, 2021, 8(1): 1-13. doi: 10.3934/bioeng.2021001 |
[9] | Frank R. Bengelsdorf, Christina Gabris, Lisa Michel, Manuel Zak, Marian Kazda . Syntrophic microbial communities on straw as biofilm carrier increase the methane yield of a biowaste-digesting biogas reactor. AIMS Bioengineering, 2015, 2(3): 264-276. doi: 10.3934/bioeng.2015.3.264 |
[10] | Ana Paula Guedes Pinheiro, Augusto Bücker, Ana Cláudia Cortez, John Edward Hallsworth, João Vicente Braga de Souza, Érica Simplício de Souza . Vinegar production from Theobroma grandiflorum SCHUM (cupuassu). AIMS Bioengineering, 2021, 8(4): 257-266. doi: 10.3934/bioeng.2021022 |
The transition from a traditional linear economy to a circular model aims to create a more sustainable future by reducing the adverse effects of agro-waste on the environment. The present study evaluated the metabolic diversity of bacterial isolates from municipal dumpsites for keratinase production and poultry feather valorization. The bacterium with the highest keratinolytic activity was identified through 16S ribosomal ribonucleic acid (rRNA) gene sequencing. The exo-keratinase production by the bacterium was optimized, and the feather hydrolysate obtained from the fermentation process was analyzed for amino acids. Among the twelve bacteria isolated from the dumpsite's sample, three showed significant feather degradation and keratinase production of 89% (744.5 ± 9.19 U/mL), 58% (269 ± 15.55 U/mL), and 46% (195 ± 7.07 U/mL) for SSB-03, SSB-02, and HSB-02, respectively. Analysis of the 16S rRNA gene sequence revealed that SSB-03 has high sequence homology with Exiguobacterium acetylicum, and thus, it was identified as Exiguobacterium acetylicum FHBD (accession number MW165834). Strain FHBD fermentation medium exhibited the maximum keratinase activity (2934.54 ± 38.56 U/mL) and sulfhydryl group content (3.09 ± 0.02 mM) at 72 h under optimal process conditions of pH 5.0, temperature (35 °C), inoculum size (2% v/v), and feather (15 g/L). Amino acid analysis of the feather hydrolysate showed significant levels of glutamic acid, aspartic acid, glycine, arginine, serine, and proline, with respective concentrations of 1.58, 1.34, 1.29, 1.20, 1.12, and 0.93 (g/100 g of sample). The study's findings emphasize the potential of E. acetylicum FHBD in poultry feather valorization and keratinase production.
Minimal salt media;
Ground chicken feather;
Polymerase chain reaction;
National Centre for Biotechnology Information;
Basic local alignment search tool
Advocating for the transition from a linear economy to a circular one, which prioritizes the use of renewable bioresources for green energy and biochemicals, can help address the global dependency on finite fossil-based resources. This shift can contribute to a more sustainable future by reducing the negative impact of waste handling [1]. However, the increasing waste stream from agro-industrial sectors could potentially overwhelm the Earth's biophysical capacity due to population growth. Therefore, it is important to explore alternatives to incorporating renewable waste biomass into the economy to support the concept of circularity and promote sustainable development [2].
The poultry industry plays a significant role in global food security by providing nutritious meats and meat products [3]. However, the processing of poultry meat generates variable by-products, including keratinous feathers, which make up a significant portion of the waste stream. In order to stimulate a sustainable and healthy agroecosystem, it is crucial to integrate efficient poultry feather recycling into the poultry production value chain. Avian feathers display high mechanical and structural stability due to their unique architectural disposition of interactive linkages, which hold the biomass together [4]. This results in super-durability and high resistance to mechanical damage or biological attack [5]. Researchers have explored various processing methods, such as pressure cooking, mechanical crushing, and chemical hydrolysis, to salvage the high crude protein content of keratinous feathers [6]. The limitations associated with existing technologies for processing keratinous poultry feathers have prompted the search for more sustainable alternatives. Microbial technology, which is both eco-friendly and cost-effective, has been identified as a promising approach for the valorization of keratin-rich waste [7].
Environmental stressors can influence prokaryotes' ability to use a variety of substrates in their environment. Stress conditions cause changes in gene expression, including the upregulation of genes in biosynthetic pathways as part of the bacterial stress response mechanism [8]. In addition to keratinophilic fungi that inhabit keratin-rich areas and secrete keratinase as a means of causing disease [9], bacteria have been widely reported to break down keratinous biomass through the expression of a robust keratinolytic system [10]. This green technological approach has gained significant traction since the isolation and characterization of Bacillus licheniformis PWD-1 from a poultry facility [11].
To date, various strains of Gram-positive and Gram-negative bacteria with keratinolytic potential have been isolated from different environments. However, there is a lack of uniformity in the ability of these bacterial strains to degrade keratinous biomass. For example, Bacillus cereus HD1 [12], Stenotrophomonas maltophilia BBE11-1 [13], Pseudomonas geniculata H10 [14], Chryseobacterium sp. RBT [15], Kocuria rhizophila p3-3 [16], and Streptomyces netropsis A-ICA [17] were able to degrade feathers by 57 ± 2%, 22.8%, 82.3 ± 3.5%, 83%, 52%, and 84 ± 2%, after 168, 96, 36, 48, 96, and 120 h, respectively.
Therefore, several bacterial strains are being studied for their ability to effectively convert keratinous biomass into valuable products. The efficient decomposition of poultry feathers by wild bacterial species is promising for the sustainable production of protein hydrolysates, which contain significant amounts of bioactive peptides, amino acids, and non-protein nitrogen [18],[19]. Protein hydrolysates generated through microbial fermentation of poultry feathers have been utilized as soil fertility enhancers due to their slow nitrogen-releasing power, plant growth hormone content, and microbial soil-nutrient recycling potential [20].
Despite the potential of municipal dumpsites as a source of novel bacterial strains with diverse metabolic activity, they remain underexplored. The complex interactions among microbial populations in this ecological niche and their involvement in nutrient recycling highlight the importance of microbial technology in achieving a regenerative and circular economy [21]. Production of hydrolytic enzymes ensures that bacteria extract relevant nutrients from both soluble and insoluble substrates for homeostasis. Therefore, this study explored the bacterial diversity of local municipal dumpsites for feather-degrading bacteria. A potent isolate, identified as E. acetylicum FHBD, was evaluated for its ability to secrete keratinase in a cheaply formulated medium, and the fermentation process conditions were optimized. The protein hydrolysate generated through bacterial digestion of chicken feathers was analyzed for various amino acids.
Soil samples were collected from the municipal dumpsites in Alice environment (32.7901° S, 26.8330° E) where variable biomaterials have decomposed. The samples were taken to the laboratory for further analysis. Approximately 1 g of soil samples was inoculated into a 250 mL Erlenmeyer flask containing 99 mL of minimal salt medium (MSM) with 10 g/L of ground chicken feathers (GCF) as the only carbon source and incubated with a constant agitation (130 rpm) for 7 days at 30 °C. The MSM composition was as follows (g/L): K2HPO4, 0.3; KH2PO4, 0.4; MgCl2, 0.2; CaCl2, 0.22; NH4Cl, 0.5 [22]. The isolation medium (100 µL) was spread on minimal salt agar after appropriate dilution of the medium. The agar plate contained similar compositions as the MSM, with additions of 15 g/L bacteriological agar and 50 µg/L of nystatin. The agar plates were incubated for 72 h and monitored for bacterial growth. Different colonies were picked from the bacterial culture based on their morphological characteristics and subcultured on freshly prepared plates. Pure bacterial isolates were preserved on GCF agar slant at 4 °C.
From the slant, a loopful of pure isolates was streaked on the GCF agar plates and incubated for 24 h at 30 °C. Bacterial cultures were further standardized on 0.5 McFarland bases, reading 0.1 absorbance at 600 nm. These inocula were used for subsequent evaluation and fermentation processes.
The isolates were subjected to skimmed milk hydrolysis evaluation as reported previously [23]. Skimmed milk agar plates were prepared using MSM, skimmed milk (10 g/L), and bacteriological agar (15 g/L). Freshly standardized inoculum (10 µL) of each bacterial culture was placed onto the skimmed milk plates and incubated at 30 °C for 24 h. Subsequently, the plates were observed for clearance zones around the isolate, and the plates showing halo zones were considered protease-producing bacterial isolates.
The proteolytic bacteria were evaluated for chicken feather degradation capability in MSM (without NH4Cl) supplemented with intact chicken feathers (10 g/L) as the only carbon and nitrogen source. The Erlenmeyer flasks (250 mL) containing 100 mL of working volume were incubated for 72 h in a rotary shaker (130 rpm) at 30 °C. The degree of chicken feathers' hydrolysis was assessed in the flasks showing feathers degradation by visual inspection and determination of percentage feather hydrolysis [24]. Extracellular keratinase production was determined using cell-free filtrates extracted by centrifugation. The isolates that showed significant degradation of chicken feathers were considered potent keratin degraders and were selected for further studies.
Keratinase activity was determined using the method described by Nnolim et al. [25]. Keratin-azure (Sigma-Aldrich, St. Louis, MO, USA) was used as an assay substrate. The keratin-azure powder (10 g/L) was suspended in 0.1 M Tris-HCl buffer, pH 7.5. The assay vial contained 0.5 mL of keratin-azure solution and 0.5 mL of crude enzyme. The tube was incubated for 30 min at 37 °C. After incubation, the unutilized substrates were removed by centrifugation at 15,000 rpm for 10 min. Azo dye released from the keratin-azure was determined at 595 nm using a 96-well plate reader (SYNERGYMx, BioTek, USA). One unit of keratinase activity was defined as the amount of enzyme causing an increase of 0.01 in absorbance at 595 nm per minute under the assay protocol described.
Bovine serum albumin was used as a reference protein to quantify the crude extract's protein concentration as reported by the method of Bradford [26]. Sulfhydryl groups' concentration in the extract was determined according to Ellman's method [27].
The colonies of the potent keratinolytic isolates were harvested after 18 h of incubation and the deoxyribonucleic acid (DNA) extraction was carried out using the Zymo Research DNA isolation kit (ZR Fungal/Bacterial DNA Kit™). The DNA template extracted was used to amplify the target 16S rRNA gene sequence using polymerase chain reaction (PCR) under standard thermal cycling conditions and primer set 27f (5′-AGAGTTTGATCMTGGCTCAG-3′) and 1492r (5′-CGGTTACCTTGTTACGACTT-3′) as forward and reverse primers, respectively [28]. PCR was performed using Ready 2× Master Mix containing DreamTaq™ DNA polymerase (Thermo Fisher Scientific). Prior to visualizing the amplified genes, the PCR products were electrophoresed using agarose gel (1.5%) appropriately stained with ethidium bromide. The position of the gel showing the bands was excised and extracted using Gel DNA Recovery Kit (Zymo Research). Forward and reverse directions sequencing was carried out using BrilliantDye™ Terminator Cycle Sequencing Kit v3.1 (NimaGen, The Netherlands). ABI 3500xl Genetic Analyzer (Applied Biosystems, Thermo Fisher Scientific) was employed to profile the purified fragments. The resulting .ab1 files generated with Genetic Analyzer were further explored using CLC Bio Main Workbench v7.6 through a basic local alignment search tool (BLAST) in the National Centre for Biotechnology Information (NCBI) database [29].
Extracellular keratinase production in MSM was optimized by varying the following variables: initial pH (3–10), incubation temperature (25–30 °C), inoculum size (1–6%, v/v), and feather concentration (5–30 g/L). In each case, the fermentation flasks were incubated with constant agitation (130 rpm) for 72 h. The optimal process variables (temperature 35 °C, pH 5.0, inoculum size 2%, feathers 15 g/L) were used to determine the time course of keratinolytic activity by the bacterial isolate. A portion of the fermentation was taken at intervals of 24 h to determine the kinetics of keratinase titer, bacterial growth, pH change, and protein and sulfhydryl groups' concentrations.
Whole chicken feathers were hydrolyzed using keratinolytic isolate in a submerged state fermentation under optimized process conditions. The hydrolysate recovered from the fermentation medium was freeze-dried using vacuum concentrator systems (Martin Christ Gefriertrocknungsanlagen GmbH, Germany) and then prepared for amino acid analysis. Digestibility assessment of the feather hydrolysate for amino acids was carried out using 9-fluorenylmethyl chloroformate quantitative derivatization and reversed-phase high-performance liquid chromatography under mild conditions following previously established methods [30],[31].
SigmaPlot version 11.0 was used to analyze the data obtained from the replicate experiments. One-way analysis of variance was used to compare the mean differences at p < 0.05 with Duncan's multiple range post-hoc test.
Twelve distinct bacteria were isolated from the soil samples collected from the municipal dumpsite, and six of the isolates showed variable proteolytic activity with halo zone diameters of 35.0 ± 7.07 mm (SSB-03), 30.5 ± 3.53 mm (HSB-02), 29.0 ± 1.41 mm (SSB-02), 17.0 ± 1.41 mm (HSB-04), and 16.0 ± 1.41 mm (SSB-04 and SSB-05). Figure 1a represents skimmed milk hydrolysis by the proteolytic isolate SSB-03. The six proteolytic isolates were further used to initiate chicken feather degradation in MSM (Figure 1b,c). The results show that three of the six isolates (SSB-03, SSB-02, and HSB-02) differentially degraded chicken feathers with a respective percentage feather hydrolysis of 89%, 58%, and 46%.
The extracellular enzyme production by SSB-03, SSB-02 and HSB-02 was evaluated using the cell-free extract of the fermentation (Figure 1c), and the results show respective keratinase activity of 744.5 ± 9.19 U/mL, 269 ± 15.55 U/mL, and 195 ± 7.07 U/mL (Figure 2). Based on the keratinolytic potential of the isolates, SSB-03 was selected for investigations. The 16S rRNA gene sequencing, analysis, and nucleotide blast against the NCBI showed that SSB-03 has 100% and 99.88% sequence similarity with Exiguobacterium acetylicum VITNS (NCBI: JX307688) and Exiguobacterium acetylicum KIS22 (NCBI: MH017384), respectively. Therefore, SSB-03 was assigned Exiguobacterium acetylicum FHBD, and the nucleotide sequence was submitted to GenBank and designated the accession number MW165834.
Keratinase production by E. acetylicum FHBD was enhanced by varying some physico-nutritional variables. Incubation temperature optimization showed an incremental enzyme production from 333.64 ± 1.29 U/mL and 497.27 ± 1.28 U/mL to 701.82 ± 17.9 U/mL when the temperature was adjusted from 25 and 30 to 35 °C, respectively (Figure 3). However, keratinase production by strain FHBD declined to 297.27 ± 11.57 U/mL when the fermentation was carried out at 40 °C, indicating that 35 °C was the optimal temperature for bacterial keratinase production. E. acetylicum FHBD extracellularly secreted keratinase at all pH tested, with optimal enzyme activity of 1492.73 ± 125.99 U/mL at pH 5.0 (Figure 3). Results also show that strain FHBD had more keratinase titer at stronger acidic (pH 3.0) conditions than stronger alkaline conditions (pH 10.0). Changing the size of the starter culture for the fermentation process from 1% to 2% (v/v) resulted in an enzyme production increase from 1415.45 ± 116.99 U/mL to 1699.98 ± 105.42 U/mL. The inoculum was further added to the production medium at concentrations of 3%, 4%, 5%, and 6% (v/v), but there was a corresponding decrease in keratinase production with enzyme activities of 1290.91 ± 41.14 U/mL, 1210.90 ± 71.99 U/mL, 1186.36 ± 96.42 U/mL, and 1029.98 ± 80.95 U/mL compared to the optimum 2% (Figure 3). Fermentation was carried out using different concentrations of chicken feathers to study their effect on extracellular keratinase production by strain FHBD. The results showed that keratinase production increased from 1795.45 ± 47.56 and 1842.73 ± 26.99 to 1997.27 ± 78.43 (U/mL) with increasing feather concentration from 5 and 10 to 15 g/L, although there was no significant difference (p = 0.471) between enzyme yield at 5 and 10 g/L (Figure 3). Above 15 g/L of chicken feathers, keratinase production decreased drastically with the least enzyme yield (729.09 ± 92.57 U/mL) obtained at 30 g/L (Figure 3).
The time-course study showed that keratinase production by E. acetylicum FHBD increased with an increasing fermentation period, with 608.18 ± 1.29 U/mL, 1180 ± 17.99 U/mL, and 2934.54 ± 38.56 U/mL after 24, 48, and 72 h, respectively (Figure 4). Beyond the optimum production time (72 h), keratinase titer decreased drastically to 1865.45 ± 53.97 U/mL, 896.36 ± 5.14 U/mL, 790.09 ± 2.57 U/mL, and 643.63 ± 38.56 U/mL at 96, 120, 144, and 168 h, respectively. The sulfhydryl groups' content in the fermentation medium showed a similar pattern to the enzyme titer, with a maximum concentration of 3.09 ± 0.02 mM at 72 h (Figure 4). The production medium maintained a constant increment in soluble protein content with increasing incubation period, recording 1.25 ± 0.05 mg/mL at 168 h (Figure 4). The pH of the fermentation medium was observed to change with increasing incubation time. The initial pH 5.0 was recorded at the start of the fermentation, while 168 h post-incubation the medium pH drifted until with a final value of 8.02 ± 0.01(Figure 4).
The protein hydrolysate generated through the bioconversion of chicken feathers was analyzed for the presence of amino acids. The results indicated that the hydrolysate contained a total protein of 45.04 g/100 g of sample. Among the amino acids detected, glutamic acid, aspartic acid, glycine, arginine, serine, and proline were significantly present with the respective concentrations of 1.58, 1.34, 1.29, 1.20, 1.12, and 0.93 (g/100 g of sample) (Figure 5). In addition, per 100 g of sample, leucine (0.78 g), valine (0.69 g), histidine (0.69 g), lysine (0.68 g), alanine (0.61 g), threonine (0.57 g), and phenylalanine (0.51 g) were also recorded in appreciable concentrations. However, methionine and tryptophan showed the lowest concentrations of 0.05 g/100 g and 0.2 g/100 g, respectively.
The ability of microbes to thrive in extreme and constantly changing environments is a testament to their power in creating sustainable ecosystems. A wide range of microbes play a crucial role in implementing clean technology, which promotes a circular bioeconomy. In recent times, poultry feather biodegradation has gained traction due to its numerous benefits in the bioeconomy sector. For this study, feather-degrading bacteria were collected from municipal dumpsite samples, which receive variable waste materials from households and local enterprises. Microbial exposure to different substrates potentially influences the diversity of their metabolic capabilities [32]. The metabolic diversity of the bacterial isolates was evident from their differential proteolytic and keratinolytic activities against the keratinous poultry feather. Among the bacterial strains evaluated, E. acetylicum FHBD showed the highest keratinolytic potential, producing extracellular keratinase and degrading feathers effectively. There is limited information on keratinous biomass degradation and keratinase secretion by Exiguobacterium spp. [33],[34]. Therefore, this study provides valuable insights into the diversity and ecological distribution of keratinolytic bacteria.
At optimized conditions, strain FHBD exhibited thermo-sensitivity within the mesophilic end of the temperature spectrum. The production of enzymes by soil microbes is known to be influenced by temperature, and the degree of sensitivity can vary among strains [35]. For example, Bacillus velezensis ZBE1 showed optimal keratinase production at 35 °C [36], while Exiguobacterium indicum secreted maximum keratinase in fermentation medium at 30 °C [34]. The growth of FHBD and its extracellular keratinase production at moderate temperatures highlight its competitive advantage in promoting sustainable development. A previous study found that weakly acidic conditions (pH 5.0) were favorable for keratinase production by E. acetylicum FHBD. pH is an important environmental factor that affects microbial growth and metabolism [37], and it also modulates the transport of metabolites from the intracellular compartments to the extracellular milieu by regulating the integrity and transport channels of cellular membranes. The pattern of keratinase production at various pH values tested suggests that strain FHBD's keratinase biosynthetic machinery functions well at acidic pH, as the same microorganism may optimally produce different metabolites at different pH conditions [38]. Other bacteria from environmental samples have shown similar acidic pH optima for exo-keratinase production [39],[40].
A suitable starter culture for enzyme production should be in an active growth phase and have a certain size. The viable biomass of strain FHBD showed optimal keratinase production at 2%, indicating that the inoculum concentration was sufficient for rapid cell growth and enzyme production. However, the decremental pattern of keratinase production with increasing inoculum size might be due to the quick depletion of essential nutrients and dissolved oxygen in the production medium caused by an overpopulated culture biomass [41]. A similar inoculum optimum was reported for keratinase production by B. velezensis ZBE1 [36] and Bacillus subtilis KD-N2 [42]. However, Bacillus oleronius [43] and Arthrobacter sp. NFH5 [44] produced maximum keratinase at 5% inoculum size, which suggests that strain peculiarity and fermentation medium composition could also influence the required starter culture. The optimization of chicken feather concentration used for keratinase production demonstrated incremental enzyme yield with increasing medium feather concentration until the optimum. This finding highlights the inducibility of the synthesis and secretion of higher keratinase titers for the degradation of increasing concentrations of keratinous biomass for bacterial nutrient acquisition [45]. However, extracellular keratinase production declined above the optimum chicken feather concentration, which might be attributed to the viscous fermentation medium resulting from excessive insoluble substrates that also affect the medium's dissolved oxygen necessary for bacterial growth and respiration [17]. Other bacterial strains reported for their preference for similar or variable concentrations of chicken feathers for maximum keratinase production include Bacillus tropicus Gxun-17 (15 g/L) [46], Thermoactinomyces sp. YT06 (10 g/L) [47], and S. maltophilia DHHJ (25 g/L) [48]. This variation in optimal feather concentrations also alludes to microbial strain differences and genetic diversity.
The highest keratinase activity was observed during the stationary phase of E. acetylicum FHBD growth. The molecular changes that occur during this phase, such as increased protein synthesis, are crucial for maintaining the bacterial cells' viability in a nutrient-depleted medium [49]. However, the accumulation of toxic products in the production medium is a common occurrence during the stationary phase [50], which could explain why the enzyme titer declined significantly after 72 h. The optimal timeline for keratinase production may also depend on the bacterial strain and medium composition. Consequently, under submerged state fermentation, P. geniculata H10 [14], Chryseobacterium sp. RBT [15], B. velezensis ZBE1 [36], B. cereus HD1 [12], and S. netropsis A-ICA [17] achieved the maximum keratinase production of 36.3 ± 2.7 U/mL, 89.12 U/mL, 11.65 U/mL, 41 U/mL, and 113.6 ± 5.1 U/mL, respectively, at different time intervals of 24, 48, 96, 120, and 120 h, respectively. The highest enzyme activity coincided with the highest medium sulfhydryl group concentration, suggesting that optimal disulfide bond reduction might be facilitated by the increased keratinase titer.
The cleavage of densely populated disulfide bonds in keratinous biomass enhances its biodegradation by exposing the peptide bonds to peptidase attacks [51], making strain FHBD a potential candidate for keratinous biomass valorization. The accumulation of sulfhydryl groups in the fermentation medium during E. acetylicum FHBD growth on poultry feathers supports the proposed synergistic mechanism of keratin biodegradation by sulfolytic and proteolytic systems [52]. Efficient dismemberment of keratin-rich biomass into soluble proteins by microbial/enzymatic action causes a medium pH drift toward the alkaline end of the spectrum by causing the deamination of degradation products releasing ammonia in the medium [15]. Similar pH changes have been reported for Bacillus sp. CN2 [19] and Ectobacillus sp. JY-23 [53] growing on poultry feathers. Additionally, the consistent rise in protein concentration in the fermentation medium following increased incubation time is expected during keratin bioconversion by a competent isolate [14],[19].
The amino acid profiling of the feather hydrolysate indicates that E. acetylicum FHBD could be a relevant candidate for sustainable development in the agri–food–environmental venues. The total amino acid content was significantly lower than the total protein content of the hydrolysate, and this difference might be attributed to the other peptide constituents that were not completely dismembered into amino acids [5]. The use of cheap protein supplements from agro-residues for livestock feed formulations has been proposed to reduce the cost of poultry production by decreasing food-feed competition and promoting resource use efficiency, thereby bolstering global food security [54]. Different types of bacterial keratinases exhibit distinct patterns of amino acid release from poultry feathers, which depend on their ability to effectively reduce disulfide linkages, recognize peptide bonds, and hydrolyze polypeptide chains [55],[56]. Hydrolysates derived from feathers through microbial or keratinase degradation offer significant value in the production of animal feed, as they provide essential amino acids for optimal growth and development of livestock. This assertion was supported by the recent report where feather meals prepared through microbial fermentation offered great nutritional requirements to broilers compared to feather meals prepared by other forms of treatment [57]. Microbial degradation of keratinous feathers requires less energy investment and is environmentally friendly. Contrariwise, feather hydrolysates were prepared using high concentrations of NaOH and HCl [58], and this unsustainable method of managing feather biomass could have adverse consequences on the environment through chemical toxicity.
The byproducts of extraction of keratinases, amino acids, and peptides from feather hydrolysates have shown great relevance, including their use as organic fertilizers in the amendment of soil fertility and as feedstock in biogas production, among others. The waste materials generated from keratinous feather recycling have been reported to possess not only high nitrogen content but also important plant growth hormones, while microbial biomass can aid in nutrient recycling and availability in the soil [59]. In bioenergy production, the microbial-generated feather biomass serves as the pretreated substrate by providing the methanogens with important metabolites and nutrients that enhance their activity during methanogenesis [60]. The metabolic capabilities of E. acetylicum FHBD make it a promising candidate for the biodegradation of sturdy feather biomass into a digestible protein source of industrial importance.
In conclusion, a bacterial isolate obtained from a municipal dumpsite sample was able to disintegrate keratinous feathers by 89% in a minimal salt medium through biosynthesis and extracellular keratinase production. The isolate was identified as E. acetylicum FHBD using a molecular identification system. The isolate demonstrated optimal performance at a temperature of 35 °C, a pH 5, an inoculum size of 2%, and a feather concentration of 15 g/L. The maximum keratinase titer (2934.54 ± 38.56 U/mL) was achieved at 72 h, which corresponded to the time of highest concentration (3.09 ± 0.02 mM) of sulfhydryl groups in the production medium. This suggests that the extensive secretion of keratinolytic enzymes is critical for efficient keratin dismemberment, highlighting the potential of strain FHBD in the circular bioeconomy. The abundance of various amino acids in the feather hydrolysates indicates the relevance of FHBD in sustainable agro-waste valorization and keratinase production. Therefore, further studies on molecular optimization and genetic engineering are imperative to create an industrially competent and robust strain for scale-up applications.
The authors declare they have not used Artificial Intelligence (AI) tools in the creation of this article.
[1] |
Muscat A, de Olde EM, Ripoll-Bosch R, et al. (2021) Principles, drivers and opportunities of a circular bioeconomy. Nat Food 2: 561-566. https://doi.org/10.1038/s43016-021-00340-7 ![]() |
[2] |
Velenturf AP, Purnell P (2021) Principles for a sustainable circular economy. Sustain Prod Consum 27: 1437-1457. https://doi.org/10.1016/j.spc.2021.02.018 ![]() |
[3] |
Choi J, Kong B, Bowker BC, et al. (2023) Nutritional strategies to improve meat quality and composition in the challenging conditions of broiler production: a review. Animals 13: 1386. https://doi.org/10.3390/ani13081386 ![]() |
[4] |
Zhang F, Jiang L, Wang S (2018) Repairable cascaded slide-lock system endows bird feathers with tear-resistance and superdurability. Proc Natl Acad Sci 115: 10046-10051. https://doi.org/10.1073/pnas.1808293115 ![]() |
[5] |
Li ZW, Liang S, Ke Y, et al. (2020) The feather degradation mechanisms of a new Streptomyces sp. isolate SCUT-3. Commun Biol 3: 191. https://doi.org/10.1038/s42003-020-0918-0 ![]() |
[6] |
Chilakamarry CR, Mahmood S, Saffe SNBM, et al. (2021) Extraction and application of keratin from natural resources: a review. 3 Biotech 11: 1-12. https://doi.org/10.1007/s13205-021-02734-7 ![]() |
[7] |
Srivastava B, Khatri M, Singh G, et al. (2020) Microbial keratinases: an overview of biochemical characterization and its eco-friendly approach for industrial applications. J Clean Prod 252: 119847. https://doi.org/10.1016/j.jclepro.2019.119847 ![]() |
[8] |
Dawan J, Ahn J (2022) Bacterial stress responses as potential targets in overcoming antibiotic resistance. Microorganisms 10: 1385. https://doi.org/10.3390/microorganisms10071385 ![]() |
[9] |
Alwakeel SS, Ameen F, Al Gwaiz H, et al. (2021) Keratinases produced by Aspergillus stelliformis, Aspergillus sydowii, and Fusarium brachygibbosum isolated from human hair: yield and activity. J Fungi 7: 471. https://doi.org/10.3390/jof7060471 ![]() |
[10] | Derhab N, Mabrouk ME, El-Metwally MM, et al. (2023) Thermostable keratinase from Bacillus cereus L10: optimization and some potential biotechnological applications. Biomass Convers Bioref 2023: 1-17. https://doi.org/10.1007/s13399-023-04887-2 |
[11] |
Williams CM, Richter CS, MacKenzie Jr JM, et al. (1990) Isolation, identification, and characterization of a feather-degrading bacterium. Appl Environ Microbiol 56: 1509-1515. https://doi.org/10.1128/aem.56.6.1509-1515.1990 ![]() |
[12] |
Yahaya RSR, Phang LY, Normi YM, et al. (2022) Feather-degrading Bacillus cereus HD1: genomic analysis and its optimization for keratinase production and feather degradation. Curr Microbiol 79: 166. https://doi.org/10.1007/s00284-022-02861-1 ![]() |
[13] |
Peng Z, Mao X, Zhang J, et al. (2019) Effective biodegradation of chicken feather waste by co-cultivation of keratinase producing strains. Microb Cell Fact 18: 1-11. https://doi.org/10.1186/s12934-019-1134-9 ![]() |
[14] |
Park G, Lee KM, Lee YS, et al. (2023) Biodegradation and valorization of feather waste using the keratinase-producing bacteria and their application in environmentally hazardous industrial processes. J Environ Manag 346: 118986. https://doi.org/10.1016/j.jenvman.2023.118986 ![]() |
[15] |
Gurav RG, Tang J, Jadhav JP (2016) Sulfitolytic and keratinolytic potential of Chryseobacterium sp. RBT revealed hydrolysis of melanin containing feathers. 3 Biotech 6: 145. https://doi.org/10.1007/s13205-016-0464-0 ![]() |
[16] |
Łaba W, Żarowska B, Chorążyk D, et al. (2018) New keratinolytic bacteria in valorization of chicken feather waste. AMB Express 8: 9. https://doi.org/10.1186/s13568-018-0538-y ![]() |
[17] |
Abdelmoteleb A, Gonzalez-Mendoza D, Tzintzun-Camacho O, et al. (2023) Keratinases from Streptomyces netropsis and Bacillus subtilis and their potential use in the chicken feather degrading. Fermentation 9: 96. https://doi.org/10.3390/fermentation9020096 ![]() |
[18] |
Kshetri P, Singh PL, Chanu SB, et al. (2022) Biological activity of peptides isolated from feather keratin waste through microbial and enzymatic hydrolysis. Electron J Biotechnol 60: 11-18. https://doi.org/10.1016/j.ejbt.2022.08.001 ![]() |
[19] | Lai Y, Wu X, Zheng X, et al. (2023) Insights into the keratin efficient degradation mechanism mediated by Bacillus sp. CN2 based on integrating functional degradomics. Biotechnol Biof Biop 16: 59. https://doi.org/10.1186/s13068-023-02308-0 |
[20] |
El-Gindy AA, Ibrahim ZM, Aziz HM, et al. (2023) Chicken feather waste degradation by Malbranchea cinnamomea and its application on plant growth and metabolites of Vicia faba plant. Biocatal Agric Biotechnol 53: 102883. https://doi.org/10.1016/j.bcab.2023.102883 ![]() |
[21] |
Zengler K, Zaramela LS (2018) The social network of microorganisms—how auxotrophies shape complex communities. Nat Rev Microbiol 16: 383-390. https://doi.org/10.1038/s41579-018-0004-5 ![]() |
[22] |
Letourneau F, Soussotte V, Bressollier P, et al. (1998) Keratinolytic activity of Streptomyces sp. S. K1–02: a new isolated strain. Lett Appl Microbiol 26: 77-80. https://doi.org/10.1046/j.1472-765X.1998.00281.x ![]() |
[23] |
Riffel A, Brandelli A (2006) Keratinolytic bacteria isolated from feather waste. Braz J Microbiol 37: 395-399. https://doi.org/10.1590/S1517-83822006000300036 ![]() |
[24] |
Bokveld A, Nnolim NE, Digban TO, et al. (2023) Chryseobacterium aquifrigidense keratinase liberated essential and nonessential amino acids from chicken feather degradation. Environ Technol 44: 293-303. https://doi.org/10.1080/09593330.2021.1969597 ![]() |
[25] |
Nnolim NE, Okoh AI, Nwodo UU (2020) Proteolytic bacteria isolated from agro-waste dumpsites produced keratinolytic enzymes. Biotechnol Rep 27: e00483. https://doi.org/10.1016/j.btre.2020.e00483 ![]() |
[26] |
Bradford MM (1976) A rapid and sensitive method for the quantitation of microgram quantities of protein utilizing the principle of protein-dye binding. Anal Biochem 72: 248-254. https://doi.org/10.1016/0003-2697(76)90527-3 ![]() |
[27] |
Ellman GL (1959) Tissue sulfhydryl groups. Arch Biochem Biophys 82: 70-77. https://doi.org/10.1016/0003-9861(59)90090-6 ![]() |
[28] |
Turner S, Pryer KM, Miao VP, et al. (1999) Investigating deep phylogenetic relationships among cyanobacteria and plastids by small subunit rRNA sequence analysis1. J Eukaryot Microbiol 46: 327-338. https://doi.org/10.1111/j.1550-7408.1999.tb04612.x ![]() |
[29] |
Altschul SF, Madden TL, Schäffer AA, et al. (1997) Gapped BLAST and PSI-BLAST: a new generation of protein database search programs. Nucleic Acids Res 25: 3389-3402. https://doi.org/10.1093/nar/25.17.3389 ![]() |
[30] |
Einarsson S, Josefsson B, Lagerkvist S (1983) Determination of amino acids with 9-fluorenylmethyl chloroformate and reversed-phase high-performance liquid chromatography. J Chromatogr A 282: 609-618. https://doi.org/10.1016/S0021-9673(00)91638-8 ![]() |
[31] |
Bank RA, Jansen EJ, Beekman B, et al. (1996) Amino acid analysis by reverse-phase high-performance liquid chromatography: improved derivatization and detection conditions with 9-fluorenylmethyl chloroformate. Anal Biochem 240: 167-176. https://doi.org/10.1006/abio.1996.0346 ![]() |
[32] |
Ke H, Li J, Zhang X, et al. (2022) Bacterial community structure and predicted metabolic function of landfilled municipal solid waste in China. Sustainability 14: 3144. https://doi.org/10.3390/su14063144 ![]() |
[33] | Gumilar J, Triatmojo S, Yusiati LM, et al. (2015) Isolation, identification and dehairing activity of Indonesian native keratinolytic bacteria Exiguobacterium sp. DG1. Pak J Biotechnol 12: 41-48. https://pjbt.org/index.php/pjbt/article/view/128 |
[34] |
Emon TH, Hakim A, Chakraborthy D, et al. (2020) Kinetics, detergent compatibility and feather-degrading capability of alkaline protease from Bacillus subtilis AKAL7 and Exiguobacterium indicum AKAL11 produced with fermentation of organic municipal solid wastes. J Environ Sci Health Part A 55: 1339-1348. https://doi.org/10.1080/10934529.2020.1794207 ![]() |
[35] |
Alster CJ, Baas P, Wallenstein MD, et al. (2016) Temperature sensitivity as a microbial trait using parameters from macromolecular rate theory. Front Microbiol 7: 1821. https://doi.org/10.3389/fmicb.2016.01821 ![]() |
[36] |
Revankar AG, Bagewadi ZK, Bochageri NP, et al. (2023) Response surface methodology based optimization of keratinase from Bacillus velezensis strain ZBE1 and nanoparticle synthesis, biological and molecular characterization. Saudi J Biol Sci 30: 103787. https://doi.org/10.1016/j.sjbs.2023.103787 ![]() |
[37] |
Jin Q, Kirk MF (2018) pH as a primary control in environmental microbiology: 1. thermodynamic perspective. Front Environ Sci 6: 21. https://doi.org/10.3389/fenvs.2018.00021 ![]() |
[38] |
Jiang J, Sun YF, Tang X, et al. (2018) Alkaline pH shock enhanced production of validamycin a in fermentation of Streptomyces hygroscopicus. Bioresour Technol 249: 234-240. https://doi.org/10.1016/j.biortech.2017.10.012 ![]() |
[39] |
Kim JM, Lim WJ, Suh HJ (2001) Feather-degrading bacillus species from poultry waste. Process Biochem 37: 287-291. https://doi.org/10.1016/S0032-9592(01)00206-0 ![]() |
[40] |
Nnolim NE, Okoh AI, Nwodo UU (2020b) Bacillus sp. FPF-1 produced keratinase with high potential for chicken feather degradation. Molecules 25: 1505. https://doi.org/10.3390/molecules25071505 ![]() |
[41] |
Abusham RA, Rahman RNZR, Salleh AB, et al. (2009) Optimization of physical factors affecting the production of thermo-stable organic solvent-tolerant protease from a newly isolated halo tolerant Bacillus subtilis strain rand. Microb Cell Factories 8: 20. https://doi.org/10.1186/1475-2859-8-20 ![]() |
[42] |
Cai CG, Lou BG, Zheng XD (2008) Keratinase production and keratin degradation by a mutant strain of Bacillus subtilis. J Zhejiang Univ Sci B 9: 60-67. https://doi.org/10.1631/jzus.B061620 ![]() |
[43] |
Parashar D, Bhatia D, Malik DK (2017) Optimization of keratinase production by Bacillus olironius isolated from poultry farm soil. J Pure Appl Microbiol 11: 1129-1134. https://doi.org/10.22207/JPAM.11.2.58 ![]() |
[44] |
Barman NC, Zohora FT, Das KC, et al. (2017) Production, partial optimization and characterization of keratinase enzyme by Arthrobacter sp. NFH5 isolated from soil samples. AMB Express 7: 181. https://doi.org/10.1186/s13568-017-0462-6 ![]() |
[45] | Zhang YZ, Zhang WX, Chen XL (2020) Mechanisms for induction of microbial extracellular proteases in response to exterior proteins. Appl Environ Microbiol 86: e01036-20. https://doi.org/10.1128/AEM.01036-20 |
[46] |
Shen N, Yang M, Xie C, et al. (2022) Isolation and identification of a feather degrading Bacillus tropicus strain gxun-17 from marine environment and its enzyme characteristics. BMC Biotechnol 22: 11. https://doi.org/10.1186/s12896-022-00742-w ![]() |
[47] |
Wang L, Qian Y, Cao Y, et al. (2017) Production and characterization of keratinolytic proteases by a chicken feather-degrading thermophilic strain, Thermoactinomyces sp. YT06. J Microbiol Biotechnol 27: 2190-2198. https://doi.org/10.4014/jmb.1705.05082 ![]() |
[48] | Wu XQ, Chen L, Cao ZJ, et al. (2012) Feather degradation and keratinase production by Stenotrophomonas maltophilia DHHJ. Adv Mat Res 550: 1400-1403. https://doi.org/10.4028/www.scientific.net/AMR.550-553.1400 |
[49] |
Jaishankar J, Srivastava P (2017) Molecular basis of stationary phase survival and applications. Front Microbiol 8: 2000. https://doi.org/10.3389/fmicb.2017.02000 ![]() |
[50] |
Pletnev P, Osterman I, Sergiev P, et al. (2015) Survival guide: Escherichia coli in the stationary phase. Acta Nature 7: 22-33. https://doi.org/10.32607/20758251-2015-7-4-22-33 ![]() |
[51] |
Yamamura S, Morita Y, Hasan Q, et al. (2002) Keratin degradation: a cooperative action of two enzymes from Stenotrophomonas sp. Biochem Biophys Res Commun 294: 1138-1143. https://doi.org/10.1016/S0006-291X(02)00580-6 ![]() |
[52] |
Jeong JH, Park KH, Oh DJ, et al. (2010) Keratinolytic enzyme-mediated biodegradation of recalcitrant feather by a newly isolated Xanthomonas sp. P5. Polym Degrad Stab 95: 1969-1977. https://doi.org/10.1016/j.polymdegradstab.2010.07.020 ![]() |
[53] |
Peng S, Li H, Zhang S, et al. (2023) Isolation of a novel feather-degrading Ectobacillus sp. JY-23 strain and characterization of a new keratinase in the M4 metalloprotease family. Microbiol Res 274: 127439. https://doi.org/10.1016/j.micres.2023.127439 ![]() |
[54] |
Sandström V, Chrysafi A, Lamminen M, et al. (2022) Food system by-products upcycled in livestock and aquaculture feeds can increase global food supply. Nat Food 3: 729-740. https://doi.org/10.1038/s43016-022-00589-6 ![]() |
[55] |
Callegaro K, Brandelli A, Daroit DJ (2019) Beyond plucking: feathers bioprocessing into valuable protein hydrolysates. Waste Manag 95: 399-415. https://doi.org/10.1016/j.wasman.2019.06.040 ![]() |
[56] |
Zhang J, Su C, Kong XL, et al. (2022) Directed evolution driving the generation of an efficient keratinase variant to facilitate the feather degradation. Bioresour Bioprocess 9: 38. https://doi.org/10.1186/s40643-022-00524-4 ![]() |
[57] |
Safari H, Mohit A, Mohiti-Asli M (2024) Feather meal processing methods impact the production parameters, blood biochemical indices, gut function, and hepatic enzyme activity in broilers. J Anim Sci 102: skae068. https://doi.org/10.1093/jas/skae068 ![]() |
[58] | Fitriyanto NA, Ramadhanti Y, Rusyadi I, et al. (2022) Production of poultry feather hydrolysate using HCl and NaOH as a growth medium substrate for indigenous strains. Earth Environ Sci 951: 012064. https://doi.org/10.1088/1755-1315/951/1/012064 |
[59] |
Tamreihao K, Devi LJ, Khunjamayum R, et al. (2017) Biofertilizing potential of feather hydrolysate produced by indigenous keratinolytic Amycolatopsis sp. MBRL 40 for rice cultivation under field conditions. Biocatal Agri Biotechnol 10: 317-320. https://doi.org/10.1016/j.bcab.2017.04.010 ![]() |
[60] |
El Salamony DH, Hassouna MSE, Zaghloul TI, et al. (2024) Bioenergy production from chicken feather waste by anaerobic digestion and bioelectrochemical systems. Microb Cell Fact 23: 102. https://doi.org/10.1186/s12934-024-02374-5 ![]() |