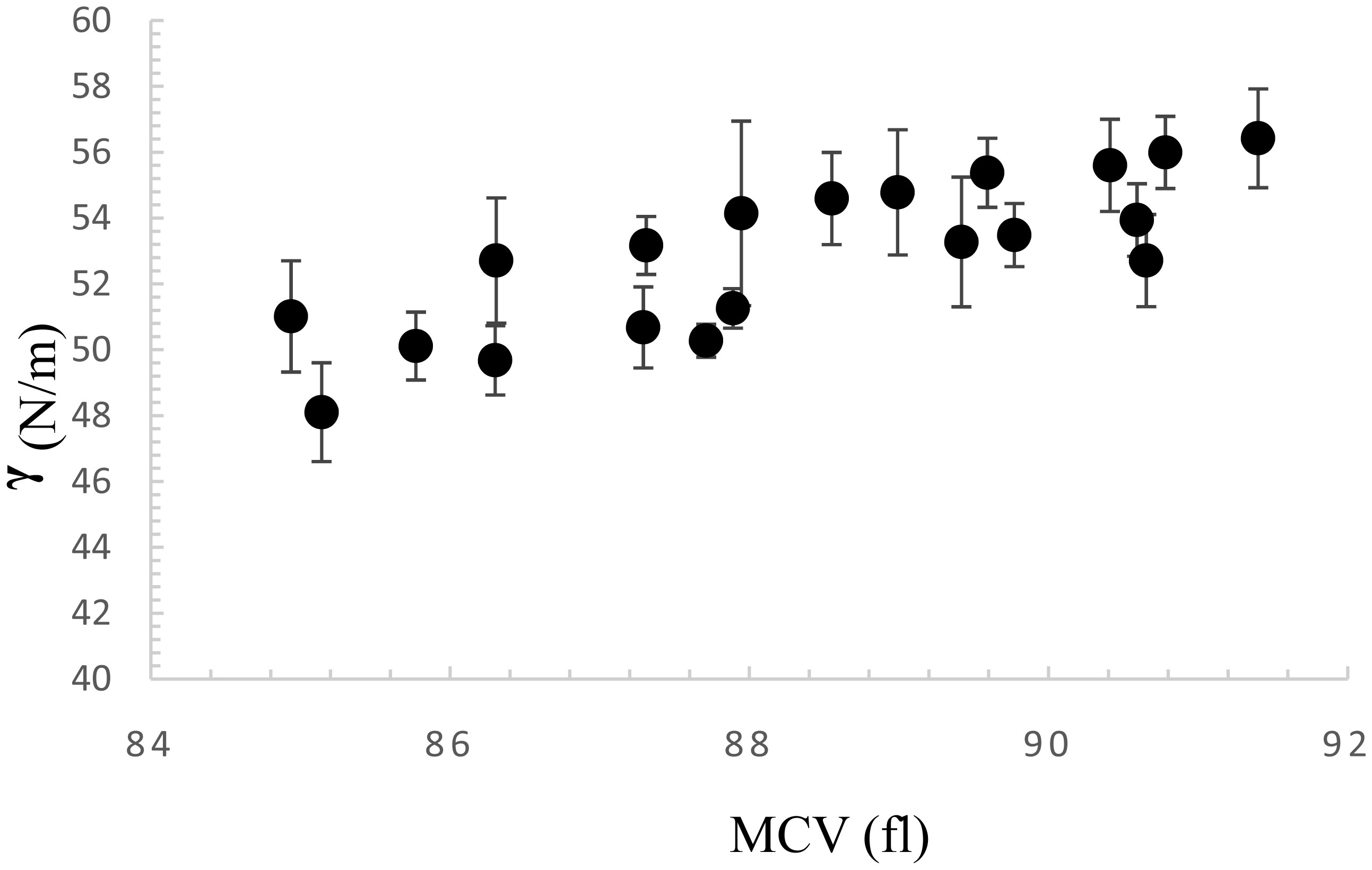
Citation: Mohamed A. Elblbesy. Impact of erythrocytes properties on blood surface tension[J]. AIMS Biophysics, 2019, 6(2): 39-46. doi: 10.3934/biophy.2019.2.39
[1] | Nikolay Kalaydzhiev, Elena Zlatareva, Dessislava Bogdanova, Svetozar Stoichev, Avgustina Danailova . Changes in biophysical properties and behavior of aging human erythrocytes treated with natural polyelectrolytes. AIMS Biophysics, 2025, 12(1): 14-28. doi: 10.3934/biophy.2025002 |
[2] | Lajevardipour Alireza, W. M. Chon James, H. A. Clayton Andrew . Determining complex aggregate distributions of macromolecules using photobleaching image correlation microscopy. AIMS Biophysics, 2015, 2(1): 1-7. doi: 10.3934/biophy.2015.1.1 |
[3] | Claudia Tanja Mierke . Physical role of nuclear and cytoskeletal confinements in cell migration mode selection and switching. AIMS Biophysics, 2017, 4(4): 615-658. doi: 10.3934/biophy.2017.4.615 |
[4] | Nily Dan . Membrane-induced interactions between curvature-generating protein domains: the role of area perturbation. AIMS Biophysics, 2017, 4(1): 107-120. doi: 10.3934/biophy.2017.1.107 |
[5] | Klemen Bohinc, Leo Lue . On the electrostatics of DNA in chromatin. AIMS Biophysics, 2016, 3(1): 75-87. doi: 10.3934/biophy.2016.1.75 |
[6] | Piotr H. Pawłowski . Charged amino acids may promote coronavirus SARS-CoV-2 fusion with the host cell. AIMS Biophysics, 2021, 8(1): 111-120. doi: 10.3934/biophy.2021008 |
[7] | Andrew H.A. Clayton . Cell Surface Receptors in the 21st Century. AIMS Biophysics, 2014, 1(1): 51-52. doi: 10.3934/biophy.2014.1.51 |
[8] | István P. Sugár . Density of electric field energy around two surface-charged spheres surrounded by electrolyte I. The spheres are separated from each other. AIMS Biophysics, 2022, 9(2): 86-95. doi: 10.3934/biophy.2022008 |
[9] | Michelle de Medeiros Aires, Janine Treter, Antônio Nunes Filho, Igor Oliveira Nascimento, Alexandre José Macedo, Clodomiro Alves Júnior . Minimizing Pseudomonas aeruginosa adhesion to titanium surfaces by a plasma nitriding process. AIMS Biophysics, 2017, 4(1): 19-32. doi: 10.3934/biophy.2017.1.19 |
[10] | Arjun Acharya, Madan Khanal, Rajesh Maharjan, Kalpana Gyawali, Bhoj Raj Luitel, Rameshwar Adhikari, Deependra Das Mulmi, Tika Ram Lamichhane, Hari Prasad Lamichhane . Quantum chemical calculations on calcium oxalate and dolichin A and their binding efficacy to lactoferrin: An in silico study using DFT, molecular docking, and molecular dynamics simulations. AIMS Biophysics, 2024, 11(2): 142-165. doi: 10.3934/biophy.2024010 |
Biological processes within the human body depend on biofluids such as blood and urine. The physical and chemical properties of biofluids are of great importance and are used in determining the health status of the human and the diagnosis of diseases [1]. One of the most important parameters of liquid is surface tension. Surface tension is a phenomenon that can be observed during daily life in many processes in nature. The surface tension of biofluids is of great importance as most biochemical reactions occur at the surface and interface [2].
Blood surface tension is one of the physical properties of blood that is of great importance in many biological functions. The area of study of blood surface tension is not considered an area of preliminary studies, but it is of practical importance in the field of diagnosis and treatment of diseases [3]–[5].
Surface tension, as an intricate property of a fluid, depends on many factors such as temperature and viscosity [6]. Human blood contains numerous low-and high molecular weight surfactants, proteins, and lipids that adsorb at fluid interface. The composition of these materials varies according to gender and age, which makes the surface tension of the blood can be changed by changing the age and gender of the human [7],[8]. In biofluids, interfacial forces are involved in cell-to-cell adhesion and maintenance of cell morphology [2].
Recently, the powerful techniques of the dynamic surface tension measurements have been developed and successfully applied to various biological liquids [8]. The recent ‘adjustments’ of such a general method of blood diagnostics are of particular importance for productive and domestic animals, for diagnostics in veterinary medicine and some biomedical applications [7].
Previous studies have focused heavily on the effect of biochemical parameters on blood surface tension, as well as study the effect of gender and age on it [2],[7],[9]. The effect of suspended blood cells on whole blood surface tension is still under investigation. Since erythrocytes represent the bulk of blood cells, this study aims to study the effect of the physical properties of erythrocytes on the surface tension of whole blood.
Human blood samples (n = 20) were collected from adult males aged 35–45 years. Blood was collected by venipuncture in evacuated tube contains EDTA as an anticoagulant. 2 mL from each sample was sent to the clinical laboratory to measure the blood indices by hematology autoanalyzer and to ensure that the samples were free of any diseases affecting the measurements. Mean cell volume (MCV), hematocrit (Hct), and erythrocytes count (RBCs) were indicated form blood indices. All samples were divided in several volumes and stored in the refrigerator at 4 °C until measurements were made. All samples were used within a maximum of 24 hours from the date of collection. When the measurements were done, the samples were incubated at 37 °C for 5 min in water path.
Bubble Pressure Tensiometer (KRUSS BP 50) was used to determine the dynamic surface tension (γ) of the blood [8],[10]. This tensiometer allowed to use small sample volume (4 mL) with fully automated measurement. The surface tension of erythrocytes suspended in phosphate buffer saline (PBS) was measured in order to evaluate the effect of the presence of plasma on whole blood surface tension.
Zeta potential measurement was done by Zeta meter System 4.0. (Zeta-Meter, Inc Staunton VA 24402 USA). This system measure Zeta potential(ξ) using a technique called microelectrophoresis. 2 mL of blood was placed in a viewing chamber called an electrophoresis cell. Then an electric field was activated. This causes the cells to move with a velocity that is proportional to their zeta potential.
Erythrocytes agammaegation and deformability were determined by a Laser-assisted Optical Rotational Cell Analyzer (LORCA, RR Mechatronics, Hoorn, The Netherlands). Erythrocytes agammaegation was also determined by the LORCA system. For determination of erythrocytes agammaegation and deformation, the blood was centrifuged at 3000 to 4000 rpm for 5 min. The plasma and buffy coat were then removed. Erythrocytes were washed three times in phosphate buffered saline (PBS) solution (pH 7.4, 290 mOsmol/kg). The washed erythrocytes were resuspended in autologous plasma with the hematocrit being fixed at 30%. The samples were sheared at a high shear rate in order to disperse all pre-existing agammaegates, following by reducing shear rate gradually to zero. The extent of erythrocytes agammaegation was determined by measuring the agammaegation index (AI). Erythrocytes deformability was measured at 37 °C based on laser diffraction patterns obtained at defined shear stress. The LORCA system determines RBC elongation under shear and reports an elongation index (EI) which is directly proportional to erythrocyte deformability.
Surface tension measurement was done in triplicate, and the result was represented as mean ± SD. Correlation analysis (Pearson's correlation) was done for the relationships between surface tension and other parameters. SPSS 23 (IBM Corp. Released 2015. IBM SPSS Statistics for Windows, Version 23.0. Armonk, NY: IBM Corp.) was used in data analysis.
The measured γ was 52.8 ± 2.3 N/m for normal blood samples. E. Hrnčíř and J. Rosina measured blood surface tension by the drop method. Their results showed that The relative blood surface tension in the examined group (n = 71) was 55.89 ± 3.57 × 10−3 (SD) and no significant differences between men and women were obtained [11]. Values of blood surface tension obtained in this study were in agreement with the previous studies which used different measurement methods of blood surface tension.
Figure 1 illustrates the relationship between surface tension and the mean volume of erythrocytes. The results showed a change in the blood surface tension with the size of the erythrocytes. A strong positive correlation (R2 = 0.65) between γ and MCV was indicated. Figure 2 shows positive relationship between blood surface tension and packed erythrocytes volume (R2 = 0.65). In contrary, erythrocytes counting was inversely proportional to surface tension (R2 = 0.78) as indicated in Figure 3. Hadidimasouleh et al. established a new model to take in a count the presence of suspended particles in colloidal suspensions when measuring surface tension by maximum bubble pressure method. They demonstrated that surface tension could be altered by the concentration and size of colloidal particles [12]. Assuming that blood is a suspension, the results obtained in this study are consistent with the results of previous studies in terms of the effect of the volume of suspended particles (erythrocytes) on the surface tension of suspension (blood).
Figure 4 shows the association of blood surface tension with the agammaegation index (AI) of the erythrocytes. Strong direct correlation (R2 = 0.6) was indicated for the relationship between γ and AI. L. Dong, and D. Johnson, studied the surface tension of charge-stabilized Titania suspensions. They found that the surface tension of this suspension strongly depends on the particle concentration. To stages of the variation in surface tension with particle concentration were indicated: i) decrease of surface tension at low concentration ii) increase in surface tension with a further increase in the particle concentration. They explained that as capillary forces may acting between suspended particles causing the increase in the surface tension at the higher concentrations [13]. Erythrocytes could be considered as negatively charged particles suspended in plasma. Erythrocytes form agammaegates at low shear rate or stasis. These agammaegates take network like shape. The association between surface tension and erythrocytes agammaegation given in this study may be explained as the size of agammaegates increase the capillary forces increase causing influence the surface tension.
Figure 5 illustrates the relationship between blood surface tension and Zeta potential. A weak correlation (R2 = 0.2) between γ and ζ was found. Figure 6 shows the relationship between the blood surface tension and erythrocytes deformability. Also, a weak correlation was found (R2 = 0.4) for the relationship between γ and EI. This can be explained as the fact that surface tension is affected by the bulk properties of the suspended particles and not by the internal properties of the particles. Plasma includes many chemicals components which have direct effect on whole blood properties. Figure 7 illustrates the effect of the presence of plasma on surface tension. The value of γ for erythrocytes suspended in plasma was 52.8 ± 2.3 N/m while for erythrocytes suspended in PBS was 50.5 ± 0.25 N/m. there was no significant deference between both values. Indeed, this confirms the hypothesis that the physical properties of blood cells can be one of the important factors in the change of surface tension of blood beside the other biochemical factors.
Particles suspended in colloidal suspension affect the surface tension of the suspension. This fact draws our attention to the need to take into account the physical properties of blood cells when studying the surface tension of blood and its derivatives. From this study it can be concluded that not only biochemical agents are affecting surface tension, but also the physical properties of erythrocytes can have an effect on it.
[1] | Schaller J, Gerber S, Kampfer U, et al. (2008) Human blood plasma proteins: structure and function. John Wiley & Sons. |
[2] | Fathi-Azarbayjani A, Jouyban A (2015) Surface tension in human pathophysiology and its application as a medical diagnostic tool. Bioimpacts 5: 29–44. |
[3] |
Kimmel E, Seri M, Fredberg JJ (1995) Lung tissue resistance and hysteretic moduli of lung parenchyma. J Appl Physiol 79: 461–466. doi: 10.1152/jappl.1995.79.2.461
![]() |
[4] |
Nieman GF, Clark WR, Paskanik AM, et al. (1994) Unilateral smoke inhalation increases pulmonary blood flow to the injured lung. J trauma 36: 617–623. doi: 10.1097/00005373-199405000-00003
![]() |
[5] |
Zaitsev S (2016) Dynamic surface tension measurements as general approach to the analysis of animal blood plasma and serum. Adv colloid interface sci 235: 201–213. doi: 10.1016/j.cis.2016.06.007
![]() |
[6] | Drelich J, Fang C, White CL (2002) Measurement of interfacial tension in fluid-fluid systems. In: Encyclopedia of surface and colloid science, Marcel Dekker Inc, 3152–3166. |
[7] |
Zaitsev S (2018) Dynamic surface tension measurements for animal blood analysis and correlations with related biochemical parameters. Colloids Interfaces 2: 5. doi: 10.3390/colloids2010005
![]() |
[8] | Kazakov VN, Sinyachenko OV, Fainerman VB, et al. (2000) Dynamic surface tensiometry in medicine. Elsevier. |
[9] |
Zaitsev SY, Milaeva IV, Zarudnaya EN, et al. (2011) Investigation of dynamic surface tension of biological liquids for animal blood diagnostics. Colloids Surf A: Physicochem Eng Aspects 383: 109–113. doi: 10.1016/j.colsurfa.2011.02.009
![]() |
[10] |
Aksenenko EV, Kovalchuk VI, Fainerman VB, et al. (2006) Surface dilational rheology of mixed adsorption layers at liquid interfaces. Adv colloid interface sci, 122: 57–66. doi: 10.1016/j.cis.2006.06.012
![]() |
[11] | Hrnčíř E, Rosina J (1997) Surface tension of blood. Physiol Res 46: 319–321. |
[12] |
Hadidimasouleh R, Yaghmaee MS, Riahifar R, et al. (2017) Modeling of the surface tension of colloidal suspensions. Surf Rev Lett 24: 1750050. doi: 10.1142/S0218625X17500500
![]() |
[13] |
Dong L, Johnson D (2003) Surface tension of charge-stabilized colloidal suspensions at the water-air interface. Langmuir 19: 10205–10209. doi: 10.1021/la035128j
![]() |