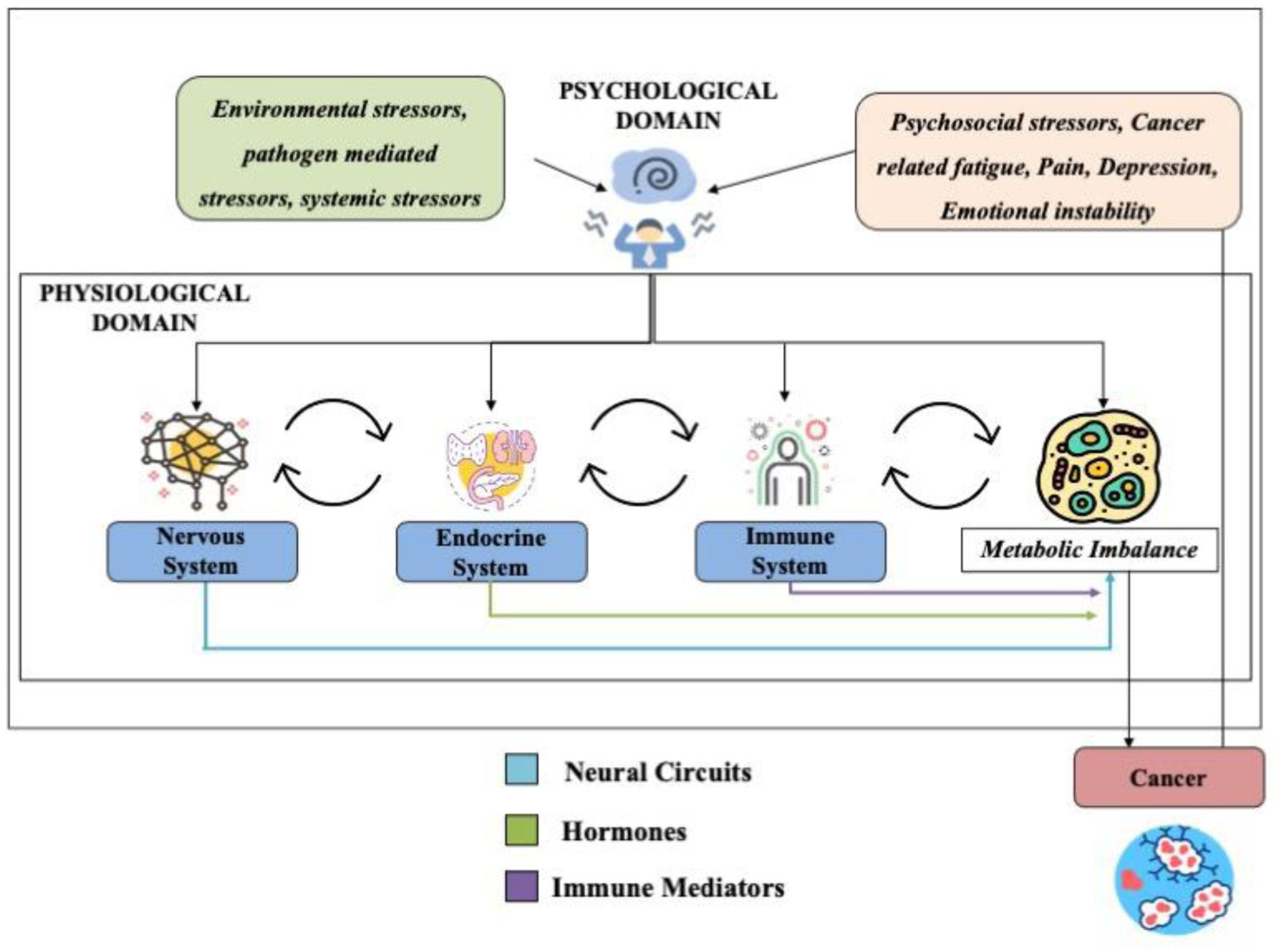
Neuroendocrine-immune homeostasis in health and disease is a tightly regulated bidirectional network that influences predisposition, onset and progression of age-associated disorders. The complexity of interactions among the nervous, endocrine and immune systems necessitates a complete review of all the likely mechanisms by which each individual system can alter neuroendocrine-immune homeostasis and influence the outcome in age and disease. Dysfunctions in this network with age or external/internal stimuli are implicated in the development of several disorders including autoimmunity and cancer. The existence of sympathetic noradrenergic innervations on lymphoid organs in synaptic association with immune cells that express receptors for endocrine mediators such as hormones, neural mediators such as neurotransmitters and immune effector molecules such as cytokines explains the complicated nature of the regulatory pathways that must always maintain homeostatic equilibrium within and among the nervous, endocrine and immune systems. The incidence, development and progression of cancer, affects each of the three systems by disrupting regulatory pathways and tipping the scales away from homeostasis to favour pathways that enable it to evade, override and thrive by using the network to its advantage. In this review, we have explained how the neuroendocrine-immune network is altered in female reproductive aging and cancer, and how these modulations contribute to incidence and progression of cancer and hence prove to be valuable targets from a therapeutic standpoint. Reproductive aging, stress-associated central pathways, sympathetic immunomodulation in the periphery, inflammatory and immunomodulatory changes in central, peripheral and tumor-microenvironment, and neuro-neoplastic associations are all likely candidates that influence the onset, incidence and progression of cancer.
Citation: Hannah P. Priyanka, Rahul S. Nair, Sanjana Kumaraguru, Kirtikesav Saravanaraj, Vasantharekha Ramasamy. Insights on neuroendocrine regulation of immune mediators in female reproductive aging and cancer[J]. AIMS Molecular Science, 2021, 8(2): 127-148. doi: 10.3934/molsci.2021010
[1] | Stephen Schultz, Dario Siniscalco . Endocannabinoid system involvement in autism spectrum disorder: An overview with potential therapeutic applications. AIMS Molecular Science, 2019, 6(1): 27-37. doi: 10.3934/molsci.2019.1.27 |
[2] | Bilal Ahmad Mir, P. F. Rahaman, Arif Ahmad . Viral load and interaction of HPV oncoprotein E6 and E7 with host cellular markers in the progression of cervical cancer. AIMS Molecular Science, 2021, 8(3): 184-192. doi: 10.3934/molsci.2021014 |
[3] | Zhaoping Qin, Patrick Robichaud, Taihao Quan . Oxidative stress and CCN1 protein in human skin connective tissue aging. AIMS Molecular Science, 2016, 3(2): 269-279. doi: 10.3934/molsci.2016.2.269 |
[4] | Xin Nian, Yasuhiro Nagai, Cameron Jeffers, Kara N. Maxwell, Hongtao Zhang . Dietary influence on estrogens and cytokines in breast cancer. AIMS Molecular Science, 2017, 4(3): 252-270. doi: 10.3934/molsci.2017.3.252 |
[5] | Patrizia A d'Alessio, Marie C Béné, Chantal Menut . d-Limonene challenging anti-inflammatory strategies. AIMS Molecular Science, 2022, 9(2): 46-65. doi: 10.3934/molsci.2022003 |
[6] | Fatima Al-Hashimi, Salvador J. Diaz-Cano . Multi-target analysis of neoplasms for the evaluation of tumor progression: stochastic approach of biologic processes. AIMS Molecular Science, 2018, 5(1): 14-62. doi: 10.3934/molsci.2018.1.14 |
[7] | Andrew V. Nguyen, Yuan-Yuan Wu, Elaine Y. Lin . The regulatory function of sphingosine-1-phosphate signaling axis on regulatory T cells in colorectal cancer. AIMS Molecular Science, 2015, 1(1): 34-47. doi: 10.3934/molsci.2015.1.34 |
[8] | Sayeeda Ahsanuddin, Minh Lam, Elma D. Baron . Skin aging and oxidative stress. AIMS Molecular Science, 2016, 3(2): 187-195. doi: 10.3934/molsci.2016.2.187 |
[9] | Fumiaki Uchiumi, Akira Sato, Masashi Asai, Sei-ichi Tanuma . An NAD+ dependent/sensitive transcription system: Toward a novel anti-cancer therapy. AIMS Molecular Science, 2020, 7(1): 12-28. doi: 10.3934/molsci.2020002 |
[10] | Kannan Mayuri, Sundaram Vickram, Thirunavukarasou Anand, Konda Mani Saravanan . MicroRNA-mediated regulation of BCL-2 in breast cancer. AIMS Molecular Science, 2025, 12(1): 32-48. doi: 10.3934/molsci.2025003 |
Neuroendocrine-immune homeostasis in health and disease is a tightly regulated bidirectional network that influences predisposition, onset and progression of age-associated disorders. The complexity of interactions among the nervous, endocrine and immune systems necessitates a complete review of all the likely mechanisms by which each individual system can alter neuroendocrine-immune homeostasis and influence the outcome in age and disease. Dysfunctions in this network with age or external/internal stimuli are implicated in the development of several disorders including autoimmunity and cancer. The existence of sympathetic noradrenergic innervations on lymphoid organs in synaptic association with immune cells that express receptors for endocrine mediators such as hormones, neural mediators such as neurotransmitters and immune effector molecules such as cytokines explains the complicated nature of the regulatory pathways that must always maintain homeostatic equilibrium within and among the nervous, endocrine and immune systems. The incidence, development and progression of cancer, affects each of the three systems by disrupting regulatory pathways and tipping the scales away from homeostasis to favour pathways that enable it to evade, override and thrive by using the network to its advantage. In this review, we have explained how the neuroendocrine-immune network is altered in female reproductive aging and cancer, and how these modulations contribute to incidence and progression of cancer and hence prove to be valuable targets from a therapeutic standpoint. Reproductive aging, stress-associated central pathways, sympathetic immunomodulation in the periphery, inflammatory and immunomodulatory changes in central, peripheral and tumor-microenvironment, and neuro-neoplastic associations are all likely candidates that influence the onset, incidence and progression of cancer.
The crosstalk among the nervous, endocrine and immune systems is responsible for the maintenance of homeostasis; and dysfunctions in this effective crosstalk pave way for the onset and progression of diseases such as cancer. These complex systems interact at multiple levels. Cancer, irrespective of the site of origin, tissue type or aggressiveness, is a disease that affects the body at the systemic level, thereby modulating the neuroendocrine-immune network on the whole to alter homeostasis and fuel its own growth [1]. Although recent studies have shifted their focus to study the dysregulation of the neuroendocrine-immune network in disease conditions such as cancer, rather than individual systems, there are a lot of unanswered questions. Are cancer-associated dysfunctions of the neuroendocrine-immune network causal or contributory to the development and progression of the disease? Most tumors are irregularly innervated by nerve fibers that directly interact with cancer cells through neuro-neoplastic synapses [2]–[5]. Although the existence of such innervations have been shown by several studies the exact mechanism by which these nerves influence or may be potential therapeutic targets have not been explored yet. Studies have shown that neural mediators can bind to receptors on cancer cells and modulate signaling pathways that may alter proliferation, angiogenesis, progression, invasion, migration and metastasis. Although a few of these pathways have been studied, how these signals may influence tumors of different hormone sensitivities is yet to be explored.
Neurotransmitters released into tumor microenvironment can also suppress immune functions, modulate inflammatory pathways and aid in cancer progression and metastasis [1],[6]. Hormone responsive tumors express receptors for endocrine mediators that bind to stress-associated outputs of the hypothalamo-pituitary-adrenal axis, thereby influencing cancer cell metabolism, growth and migration [7]–[9]. Tumor microenvironment attracts routine immune surveillance during stages of cancer development beginning from the initial cellular level anomalies, and stimulation of angiogenesis; to migration, invasion and colonisation [10]–[12]. In each stage, cancer cells evade immunosurveillance by modulating their interaction with immune mediators through various mechanisms including: shedding cancer antigens that may be used to target them, releasing immunosuppressive molecules into the tumor microenvironment, using innate immune machinery for promoting angiogenesis and through metastatic movement [13],[14]. The endocrine milieu at the cellular level is modulated by cancer-induced direct autocrine secretion of hormones that drive the growth of the tumor itself and suppress or alter immune-cell functions in the microenvironment [15]. It is still unclear how these inflammatory and immunosuppressive processes work in tandem to aid the progression of the disease and if these markers can be altered to influence prognosis.
Centrally, psychological and emotional responses to cancer at the systemic level may trigger the release of stress-associated signals through the HPA axis, ANS or SNS leading to immunosuppressive effects (Figure 1) [16]. Chronic stress can trigger inflammatory responses that may aid tumorigenesis by supporting the tumor microenvironment, enhance neuroinflammatory processes that can impair neural responses to stress and cause selective immunosuppression of type 1 helper T cells (Th1), cytotoxic T cells (CTL) and increase the risk of cancer invasion and metastasis [17]–[19]. In females and males, the age-associated alterations in gonadal hormone secretion can influence the incidence and progression of certain types of cancers such as cancers of the endometrium, breast and ovary in women and cancer of the prostate in men [20],[21]. Hence it is important to explore the role of female reproductive aging, central stress-associated pathways, peripheral immunomodulatory sympathetic innervations, tumor-associated neuro-neoplastic associations, tumor-associated/mediated endocrine secretions and tumor-associated alterations in immune effector cells and molecules in modulating neuroendocrine-immune homeostasis to fuel cancer.
Reproductive aging in female mammals such as humans is characterized by a progressive transition from regular reproductive cycles to irregular cycles, acyclicity and ultimately the loss of follicles and fertility [22]. The reproductive cycle involves two phases: the ovarian cycle (follicular and luteal) and the uterine cycle (menses, proliferative phase, and secretory phase), that prime the uterus for fertilization and is accompanied by a concomitant rise and fall in the levels of associated female sex hormones released during the 28-day period [23],[24]. This rhythmic process is tightly regulated by the hypothalamus-pituitary-gonadal (HPG) axis, hence indicating the importance of neuro-endocrine signalling in the maintenance of the reproductive cycles in females [22],[25]. Aging in women eventually leads to loss of fertility and is characterized by a slow transition from menarche, regular cycles, perimenopause, to finally menopause, accompanied by alterations in the levels of hormones secreted by the HPG axis [26]–[28]. Studies from our lab have shown that the bi-directional communication in the neuro-endocrine immune network is altered during reproductive aging [26].
Dysregulation of the HPG axis, following the loss in effective crosstalk between the nervous and endocrine systems, contributes to significant changes in the immune system [24]. This is brought about by alterations in the levels of hormones, such as estrogen and progesterone, released by the HPG axis during reproductive aging [29]. Estrogen exerts immunomodulatory effects by directly binding to estrogen receptors on immune cells and transduces subsequent signalling cascades that alter immune-mediated functions. In the lymphoid organs, estrogen is involved in the regulation of maturation, differentiation, activation and proliferation of lymphoid cells and hence may influence the outflow of cytokines and antibodies [30]. Estrogen also influences T cell maturation (Th2 type) and influences humoral responses to diseases [31]. Estrogen plays an immunomodulatory role indirectly by altering peripheral sympathetic noradrenergic (NA) innervations of the lymphoid organs and by reversing chronic stress-associated adrenergic immunosuppression [27],[32]–[34].
Progesterone shows immunomodulatory effects by binding predominantly to progesterone receptors and to a lesser extent to glucocorticoid and mineralocorticoid receptors on immune cells and mediating downstream signals [35]. Progesterone has broad anti-inflammatory effects including decreased production of TNF and IL-1b cytokines, inhibition of inducible nitric oxide synthase (iNOS) and nitric oxide (NO) pathways, and blockade of TLR and NF-kB pathways in macrophages and dendritic cells, decreased production of IFN-γ via caspase-dependent apoptosis in NK cells, and promotion of Th2 type CD4+ cell response through IL-4, IL-5, IL-10 production in helper T cells [36]–[41]. Thus, steroid hormones released by the HPG axis play a crucial role in positively modulating immune cell functions in aging women. Studies have shown that the susceptibility to diseases such as cancer, increases with progression of age due to dysregulation of the neuroendocrine-immune network [26],[37].
The development of female-specific cancers, such as breast, ovarian and endometrial cancer, can be attributed to the altered outflow of hormones released from the dysregulated HPG axis. The release of hormones estrogen and progesterone has been shown to favour the proliferation of cancer cells despite its immune-enhancive effects [42]. Therapeutic increase in the levels of estrogen and progestogen (synthetic analogue of progesterone) significantly increases the risk of breast cancer, endometrial cancer and ovarian cancer depending on the type of progestogen used [43]–[46]. However, treatment with only estrogen has shown to reduce the risk of breast cancer; and the absence of progesterone, backed by the “unopposed estrogen hypothesis”, promotes mitotic activity of endometrial cells [47],[48]. Despite the roles of female sex hormones produced by the HPG axis in promoting cancers such as breast, endometrial and ovarian cancer, studies have shown that females are less prone to malignancy that males [49]. The role of differences in cancer aetiology including sex differences in hormonal regulation, gene expression, immune function, oxidative damage, autophagy, or a combination of factors that confers protection to females against cancer needs to be explored [50],[51]. Additional studies also suggest that estrogen may play a role in upregulating the PD-1 and PD-L1 expression which may contribute to immunosuppression of T cells, B cells, Macrophages and NK cells [53]–[55]. Studies from our lab have shown that treatment of splenic lymphocytes with estrogen can enhance Con-A-induced proliferation and IFN-γ production dose-dependently and reverse immunosuppression by adrenergic agonists in Con-A-stimulated cells in vitro [56],[57]. Middle-aged female rats treated with 0.6mg slow-release pellets implanted at the scruff for 30 days showed significant increase in hippocampal, and splenic NGF expression, TH expression indicating central and peripheral benefits [58],[59]. Similarly, women in their follicular phase showed better cell-mediated immune responses compared with luteal and old women indicating that cyclic variations in estrogen and decline with reproductive age influence neuro-immunomodulatory responses and may hence be implicated in the development of female-specific cancers and autoimmunity [60]. Put together, these reports show that the sex hormones, such as estrogen and progesterone, released by the HPG axis in women may play a dual role in the onset and progression of cancers. The role of dose/duration of exposure to hormones and co-stimulatory molecules needs to be explored in animal models of cancer to better understand the synergistic milieu that may cause onset or disease progression.
The biological responses to stress are under tight regulation through the combined action of two systems, the hypothalamus-pituitary-adrenal axis (HPA) and sympathetic nervous system (SNS) which releases glucocorticoids and catecholamines, respectively, and both are activated by the central nervous system (CNS) [61]. The stress-related behavioural factors can activate (or inhibit) the HPA and SNS, and the mediators of these pathways can modulate pathological mechanisms in the tumor microenvironment [61]. The key role of the biological circuit is played by paraventricular nucleus of the hypothalamus that recognises any stressful situation, integrating human experiences with physiological signaling and releasing the corticotropin-releasing hormone (CRH) [62]. CRH triggers the pituitary gland to release adrenocorticotropic hormone (ACTH). ACTH acts on the adrenal cortex to release glucocorticoids, mainly cortisol, which is released in an inactive form, cortisone. The cortisone is then converted to the active form cortisol and back to cortisone by the enzymes 11-β hydroxysteroid dehydrogenase type 1 (11-β HSD-1) and 11-β hydroxysteroid dehydrogenase type 2 (11-β HSD-2), in the target organs [63]. The production of cortisol is regulated by a negative feedback system via hypothalamus and pituitary gland [64]. The sympathetic fibres play a significant role, by releasing norepinephrine and contributing to potentiation of cancer progression and development of metastases [21]. Both the HPA and SNS pathways maintain the stress response of the body. However, chronic stress negatively affects the body due to prolonged exposure to stressors, a phenomenon known as allostatic load where the demand exceeds the adaptive capacity of an individual, which contributes to development of the disease [65]. Chronic stress has shown to play key role in cancer progression, due to upregulated levels of glucocorticoids and catecholamines [21],[66]. Studies have shown increased levels of circulating catecholamines and glucocorticoids in response to stress, resulting in pro-tumorigenic activities [67],[68]. Enhanced expression of β-adrenergic receptors has also been reported in breast cancer [69]. Cancer initiation, progression and metastasis are mediated by sympathetic neural mediators via β-adrenergic signaling, which are also involved in mediating associated psychosocial, noetic and somatic signals [70]. The β2-adrenergic receptor expression was found to be higher in gastric cancer tissues than normal samples and activation of these receptors was associated with increased malignancy [71]. Adrenergic stimulation of splenic lymphocytes was significantly immunosuppressive, irrespective of receptor subtype [6],[56]. Catecholamine-induced β-adrenergic signals have been shown to enhance cancer cell proliferation, as well as tumour growth, through ERK1/2 and promote cancer cell survival, proliferation and angiogenesis through STAT [72],[73]. Lymph node metastasis was also associated with higher β2-AR receptor expression [74]. Unpublished data from our laboratory has shown that adrenergic stimulation of ER+ and ER-mammary tumor cell lines differentially altered survival signals in vitro, possibly implicating crosstalk between estrogen and adrenergic signaling pathways. It would be interesting to study how adrenergic immunosuppression would promote the progression of cancers in animal models of the disease.
Various experimental studies have found that chronic stress, as well as significant acute stress, promotes tumor incidence and progression through impairment of antigen presentation, T and NK cell suppression, and enhancement of T regulatory cells [75]–[78]. The health-related quality of life (HRQoL) of cancer patients is negatively affected by the psychological stressors associated with the diagnosis and treatment of cancer and affect disease prognosis [21],[79]. It has been observed that the stigma attached to disfigurement was significantly associated with functional impairments, leading to psychological distress and poor prognosis [80],[81]. Although it is clear that cancer affects several brain circuits, it is difficult to determine its effects on the human brain as it might be overlapped by the effect of psychological factors associated with the diagnosis of the disease. This necessitates animal experiments in order to delineate the specific effects of cancer on the brain [82],[83].
The sympathetic nervous system is a part of the autonomic nervous system that keeps the body in a heightened state of awareness for a “fight or flight” response to external and internal stimuli. Sympathetic nerves have been shown to densely innervate the primary (bone marrow and thymus) and secondary (spleen, lymph nodes, etc.) lymphoid organs and hence establish a conduit for possible neural-immune interactions. These nerves primarily release catecholamines, norepinephrine (NE), neuropeptide Y and endogenous opioids such as enkephalins and endorphins, all of which have been shown to affect immunity [84],[85]. Sympathetic noradrenergic (NA) circuits that innervate the primary and secondary lymphoid organs are the most widely studied due to the abundance of adrenergic receptors found in the cells of the innate and adaptive immune system [86]. Briefly, the noradrenergic input to the lymphoid organs is sent via small, unmyelinated post-ganglionic NA fibers along the vasculature. Tracing studies and ganglionectomy have shown that this communication is established through the innervations of axons of pre-ganglionic cell bodies located in the intermediolateral column of upper lumbar and thoracic spinal cord on the post-ganglionic cell bodies present in sympathetic chain or collateral plexuses in conjunction with the great vessels [87]. Thus, the presence of adrenergic receptors on immune cells and the dense sympathetic noradrenergic innervation of the lymphoid organs, provides a gateway for effective communication and signal transduction between the nervous and the immune system. Studies from our lab have shown that dysregulation of the effective bi-directional communication among the nervous, immune, and endocrine systems leads to aging and the onset and progression of age-associated diseases such as cancer [9]. Treatment of splenic lymphocytes with adrenergic agonists significantly decreased proliferation and cytokine expression irrespective of receptor subtype and dose indicating that adrenergic signals may play an immunosuppressive role and aid in the maintenance of naïve cells in the periphery [6],[56]. Age associated decline in peripheral sympathetic innervation in the lymphoid organs disrupts neuroendocrine-immune homeostasis, and regeneration of fibers helps to restore homeostasis and check the progression of mammary tumors in animal models [88]. Further, onset of age-associated decline in sympathetic fibers is much earlier in female rats compared to males, suggesting that altered neuroimmunomodulation in the periphery may play a role in female-specific cancers and autoimmunity [34]. Sex-based differences have been shown by our lab on age-associated alterations in cell- and humoral-mediated immune functions, neural-immune mediators, inflammatory markers, trophic factors, and compensatory mechanisms in healthy aging and in rheumatoid arthritis [89],[90]. Differences in cellular events that facilitate the crosstalk among hormone receptors and neural mediators and their implications in determining the predisposition to or incidence and progression of age-associated diseases and cancer in males and females is still unclear.
One way to differentiate a cancer cell from a normal cell is by its potential to evade immune destruction [14]. The immune system strictly prevents cell anomalies that lead to cancer cell formation through the cancer-immunity cycle. This process involves the priming and activation of immune cells upon cancer antigen release, presentation by antigen presenting cells (APC's) and the subsequent trafficking of immune-effector cells such as T cells to perform the required actions to eliminate the anomalies that lead to cancer cell formation [91]. Additionally, the state of cancer is monitored by the process of immunoediting which utilises the functions of cells of the innate and adaptive immune system. This process categorizes that state of cancer into elimination (cancer immunosurveillance), equilibrium (cancer persistence/dormancy) and escape (cancer progression) [92]. From this, it is evident that the cells of the immune system play a vital role in determining the onset and progression of age-associated diseases such as cancer.
Evidence has shown that norepinephrine (released by sympathetic noradrenergic nerves) plays an important role in modulating immune cell response via the adrenergic receptors. These adrenergic receptors bind to norepinephrine and lead to downstream signalling cascades that regulate the activity of immune cells such as T cells and B cells [85],[93]–[95]. A major portion of immune modulation is brought upon by the stimulation of the β-2 adrenergic receptors (β-2 AR) on immune cells. For instance, NE has been shown to impact T cell development and upregulate the magnitude of Th1 cell-mediated response respectively through the β-2 adrenergic receptor. However, the role that NE plays during Th2 development and/or progression of Th2 cell-mediated response is still unclear [96]–[98]. Similarly, norepinephrine has also been shown to modulate B-cell mediated release of IgG1 and IgE antibodies through the stimulation of the β-2 adrenergic receptor. Studies shows that β-2 AR stimulation in a B cell that is activated in the presence of IL-4 may induce the activation of two distinct signalling pathways in a B cell to regulate the level of IgG1 and IgE produced and may also upregulate CD86 expression on a B cell to participate in mediating the antibody increase [84],[86],[99]. In summary, the nervous system might directly affect immune modulation of processes related to cancer through sympathetic noradrenergic innervations which is crucial for maintaining homeostasis at cellular levels. In support of this, it was shown that in the absence of noradrenergic input there is increased anti-inflammatory effects and lytic activity of CD8+ cells through sympathetic activation [10].
Apart from the abovementioned systemic alterations in neuroendocrine-immune functions, crucial changes occur within the tumor microenvironment that warrant close study. Specific changes in the nature and functions of the immune cells in the tumor vicinity are mediated by the tumor cells themselves to facilitate neoplastic growth. Of special interest are the neuro-neoplastic interactions in the tumor microenvironment and their role in prognosis which has been less widely studied [70]. Finally, the role of tumor associated endocrine secretions and their autocrine and paracrine functions and implications in disease prognosis which also contribute to the tumor microenvironment needs to be studied.
The tumor microenvironment (TME) encompasses the extra cellular matrix, cellular subtypes such as immune cells, fibroblasts, lymphocytes, neuroendocrine cells, adipose cells, and blood and lymphatic vasculature [100]–[103]. T cells destruct tumor cells by recognizing and reacting to tumor-associated antigens through their T cell receptors (TCRs). Recent studies have shown that the exhaustion and functional impairment of T cells in the TME is a defining feature of many cancers [104]. The various components of the TME exert adverse effects on T cells limiting their differentiation and promoting cellular dysfunction. The tumor cells within the TME also exhibit immunosuppressive properties through production of toxic metabolites that inhibit T cell responses [105]. Myeloid-derived suppressor cells (MDSCs) play pivotal roles in promoting tumor progression and contribute to immunosuppression through the release of high levels of arginase (ARG)-1, inducible nitric oxide synthase (iNOS), reactive oxygen species (ROS) and cyclooxygenase-2 (COX2) all of which contribute to T cell apoptosis [106].
Tumor-associated macrophages (TAMs) are a class of immune cells present in high numbers in the microenvironment of solid tumors. As crucial drivers of chronic cancer-associated inflammation, their involvement has been described in every step of cancer progression, from early neoplastic transformation throughout metastatic progression to therapy resistance [107],[108]. Macrophages can be either pro-inflammatory (M1 type due to IFN-γ) or anti-inflammatory (M2 type due to IL-4 and IL-13). In the initial stages of carcinogenesis, macrophages show a M1 like polarization that works to eliminate immunogenic cancer cells. With progression of the tumor, the TME draws out the M2 polarization hence making it pro-tumorigenic [108]. Polarization of TAMs is regulated by multiple microenvironmental cytokines, chemokines, growth factors, and other signals derived from tumor and stromal cells [109]. Vascular endothelial growth factor-A (VEGF-A) exhibits pro-angiogenic effects and is also shown to foster cancer metastasis by inducing M2 polarization of TAMs in the presence of cytokines IL-4 and IL-10 [110]. Furthermore, other chemokines such as IL-4, IL-6, IL-13, CCL7, CCL8, CCL9, CCL18, and CXCL12 are also highly expressed in tumors and entail TAM recruitment and polarization [111]–[114]. Hypoxia, one of the main features of solid tumors, develops due to unprecedented cell proliferation, altered metabolism in cancer cells and tumor angiogenesis, resulting in reduced oxygen and nutrient content for normal cells [115],[116]. Various chemokines such as CCL2, CCL5, VEGF, SEM3A, oncostatin M and CSF-1 are invariably induced in hypoxic conditions and are accountable for the activation of TAMs and evading immune destruction in the TME [117]. Due to hypoxic conditions, lactate is predominantly produced in the TME due to anaerobic glycolysis which is shown to be a key inducer of M2 phenotype in macrophages. Enhanced expression of G protein-coupled receptor 132 (Gpr132) has been shown to activate downstream signals and modulate the expression of polarization-related genes in breast cancer patients. Studies have also shown a positive correlation between Gpr132 level and M2 macrophage infiltration and metastasis in mice with breast cancer [118]. Although hypoxia fine tunes activation and M2 polarization, it may not be solely responsible for TAM infiltration and hence warrants further study.
The initiation and progression of cancer is regulated by nerve fibres in the tumor microenvironment, although the sympathetic, parasympathetic, and sensory innervations do not play equal roles in all cancers [119]. Evidences of increased nerve density in many cancers have shown the significance of tumor-associated nerve plasticity [120]–[122]. The emerging pathological feature of many cancers is the process of perineural invasion (PNI), a distant source of metastatic spread well beyond the extent of local tumors. It is one of the important causes of tumor recurrence, mainly due to synergistic neural-epithelial interaction [123],[124]. The PNI have been shown to be a prognostic indicator and an independent predictor of outcome in colorectal cancer [125]. The sympathetic neurotransmitters and their cognate receptors have been shown to modulate several hallmarks of cancer, such as proliferation, immune escape, angiogenesis, and extracellular matrix invasion [21],[126]. The expression of neural progenitor doublecortin (DCX+) from the central nervous system has been found to drive neurogenesis in cancer in mouse models [127]. The neuropeptide Y (NPY) family of peptides, in addition to its many physiological actions, has also been shown to be involved in the modulation of tumor progression in endocrine-related cancers, through NPY receptor expression or NPY-related peptide secretion or both [128]. The association of NPY with tumor progression is found to be due to the activation of G-protein coupled receptors, Y-Rs such as Y1-R, which modulates the proliferation of tumor cells, and Y2-R which enhances angiogenesis [129]. Cholinergic stimulation has been shown to induce nerve growth (NGF), and the over-expression of this paracrine mediator NGF, promotes carcinogenesis [121],[129]. Synthetic monoamine oxidase inhibitors such as deprenyl and desmethyldeprenyl have been shown to reverse age-associated loss of sympathetic fibers, expression of neuropeptides, nerve growth factors and neurotransmitters in the periphery and prevent the progression of tumors [88],[130]. It would be interesting to see how these molecules modulate neuro-neoplastic associations within the tumors themselves.
The mitogenic effects of the hormones have been implicated in cancer progression [131]. Studies have shown estradiol (E2) as a contributing factor towards carcinogenesis, and that administration of E2 causes breast cancer [132],[133]. An autocrine and paracrine β-catenin independent ligand, Wnt5a, has been found to induce carcinogenic signals, and trigger the migration and invasion of tumor cells [134]. The activation of canonical Wnt pathway and transactivation of EFGR in autocrine Wnt signaling also contributes to tumor proliferation in breast cancer [135]. Transforming growth factor beta-1 (TGFβ-1) regulates cancer progression through endocrine, paracrine and autocrine mechanisms, and enhanced expression is shown to be associated with increased breast cancer risk [136]. It has been found that autocrine and paracrine signaling of TGFβ-1 is also mediated through neuropeptide receptor [137]. The neuropeptides such as bombesin-like peptides, gastrin-releasing peptide (GRP) and neuromedin B (NMB) have been shown to function as autocrine growth factors in lung cancer cells [138]–[140]. Dispersed sub-population of neuroendocrine cells in cancers have been shown to express variety of bio-active neuropeptides including GRP [141],[142]. The autocrine actions of peptides, gastrin and cholecystokinin (CCK) are reported in gastrointestinal carcinomas [143]. The co-expression of gastrin and CCK-B receptors is observed in cultured pancreatic carcinoma cell lines and the growth of these cell lines is inhibited by CCK-B receptor antagonists and gastrin neutralizing antibodies [144],[145]. The neuropeptide, neurotensin (NT), provides yet another example of a peptide that serves dual functions as a neurotransmitter or neuromodulator in the nervous system, and a hormone in the periphery. Evidence indicates that NT also serves as an autocrine growth factor in prostate cancer. NT receptors were detected in an androgen-independent prostatic carcinoma cell line and NT stimulated the growth of these cells, indicating that NT could contribute to the growth or survival of prostate tumor cells [146].
Inflammation plays a crucial role in the immune system to defend against several pathogens, including viruses and bacteria, and has been shown to mediate the initiation, progression, angiogenesis and metastasis of tumors (Figure 2). Several cytokines and mediators of inflammation are attracted by oncogenic changes, hypoxia, chemokines, and other signalling molecules. Immune cells infiltrate the tumor microenvironment and secrete cytokines, chemokines and growth factors which contribute to the progression of tumor [147]. There is an increased production of pro-inflammatory cytokines in the TME, however, they lack specific functioning and cannot elicit an immune response [148]. One of the distinct physiological difference among tumor and normal cells is hypoxia which can in turn induce an array of cytokines and chemokines. Tumor necrosis factor (TNF) is a versatile cytokine that exerts its role in cell differentiation, proliferation, survival and death [149]. TNF-α in particular plays a double-edged role; being both proinflammatory and tumorigenic. Studies have shown that the effect of TNF-α depends on both concentration and expression site within the tumor. In non-small cell lung cancer, patients with increased TNF-α production in the macrophages and mast cells show higher survival rates than patients expressing stromal TNF-α [150]. TNF-α exerts its function by activating nuclear factor-κB (NF-κB) and c-Jun N-terminal kinase (JNK) signalling pathways [150]. One of the mechanisms for the pro-tumorigenic effect of TNF-α is through increased production of reactive oxygen and reactive nitrogen species, which can induce DNA damage and facilitate the progression of tumors [151],[152]. In breast cancer, TNF-α activates the NF-κB pathway through TNFR1, leading to the upregulation of the oncoprotein HBXIP, which in turn upregulates TNFR1, thereby creating positive feedback loop [153].
Interleukin-10 (IL-10) was initially shown to be produced by T-helper cell type 2 (Th2) cells which inhibits Th1 cell type activation and cytokine production [154]. IL-10 has been shown to exert pro-tumorigenic effects by promoting tumor growth and metastasis. The TAMs in microenvironment produce high levels of IL-10 that could be associated with tumor-mediated immunosuppression, thereby setting the stage for tumor progression [155]. Increased expression of IL-10 by Th2 cells has also been implicated in suppressing immune surveillance against cancer growth, despite no direct evidence [156]. IL-6 supports progression of tumors by promoting proliferation and consecutively inhibiting apoptosis, thereby facilitating two major hallmarks of cancer [14],[157]. IL-6 mediates its effects through IL-6 receptor (IL-6R) and coreceptor gp130, which subsequently activate the JAK/STAT pathway [158]. Similar to TNF-α, IL-6 also facilitates tumor development by conversion of normal cells to tumor cells by upregulating Oct4 oncogene through the IL-6R/JAK/STAT signalling pathway [158]. Interestingly, there are reported evidences which show that the cytokines synthesized in the tumor microenvironment might mediate cancer-associated effects in the central nervous system. Cancer-induced hypothalamic inflammation may in-turn play a role in the development of cancer anorexia and cachexia [159].
Increasing evidence shows pro-tumorigenic effects of anti-inflammatory cytokine transforming growth factor β (TGF-β). The role of TGF-β in cancer varies according to the cell type and stage of cancer. In the initial stages, TGF-β is anti-tumorigenic, by suppressing oncogenes and promoting apoptosis. But in later stages it is seen that TGF-β promotes invasion and metastasis by inducing epithelial-mesenchymal transition (EMT) [160]. During cancer induction, TGF-β inhibits cyclin-dependent kinase p21 and upregulates c-myc expression [161]. Studies have shown that the expression of TGF-β mRNA in gastric, colorectal, prostate, breast and bladder cancers positively corelated with the degree of invasion and metastasis [162]. Thus proinflammatory milieu in the tumor microenvironment can aid in the initiation and progression of disease.
The dysfunctions in the neuro-endocrine immune networks have been shown to set the stage for the onset and progression of various age-associated diseases including cancer. These complex systems interact at multiple levels. Both neuroendocrine (the primary hormonal pathway is hypothalamic–pituitary–adrenal axis) and neuronal (direct sympathetic innervation of the lymphoid organs) pathways are involved in the control of the humoral and cellular immune responses. Chronic activation of sympathetic fibers adversely affects anti-cancer immunity and directly potentiates cancer progression. Stress plays a major role by modulating the HPA axis which sets a series of reactions in motion, and further contributes to the dysfunctional bi-directional communication among the systems. Catecholamines are a major class of neurotransmitters released by the sympathetic nerves in response to stress that modulate immune functions important for tumor immunity, including Th and B cell functions, activation of tumor-associated macrophages, and NK cell activity. The immune system, in turn, may communicate with the CNS through immune products, primarily cytokines, leading to the direct CNS activation. Production of cytokines and growth factors enhance tumor initiation through inflammation. Initially, tumor growth depends on increased cellular proliferation and decreased cell death, both of which are stimulated by inflammation-driven mechanisms. Peripheral nerve invasion provides another pathway for the spread of cancer cells in the absence of blood and lymphatic metastases, by facilitating tumor-promoting interactions between the tumor microenvironment and the nerve fibres. The nervous system modulates angiogenesis, influences the tumor microenvironment, bone marrow outputs, immune functions and inflammatory pathways to influence metastasis. In addition to these factors, the TME also responds to peripheral signals by producing growth factors, stimulating angiogenesis, evading apoptosis, inducing cytokine storms and expressing other transcription factors that promote invasion and metastasis leading to the progression of cancer (Figure 2).
Thus, the incidence and progression of cancer involves: immunomodulation by neural output, endocrine factors and tumor-associated mediators; neuromodulation by immune effector molecules, tumor microenvironment and associated hormones; and endocrine dysfunctions mediated by stress-associated neural signals, cytokines and autocrine tumor hormones. The loss of regulatory control over the neuroendocrine-immune network and its role in mediating the incidence and progression (associated physiological and psychological manifestations) of age-associated cancers needs further exploration in order to identify possible targets for therapeutic intervention.
[1] | Ader R, Felten DL, Cohen N (2001) Psychoneuroimmunology New York: Academic Press. |
[2] |
Seifert P, Spitznas M (2001) Tumours may be innervated. Virchows Arch 438: 228-231. doi: 10.1007/s004280000306
![]() |
[3] |
Seifert P, Benedic M, Effert P (2002) Nerve fibers in tumors of the human urinary bladder. Virchows Arch 440: 291-297. doi: 10.1007/s004280100496
![]() |
[4] |
Lu SH, Zhou Y, Que HP, et al. (2003) Peptidergic innervation of human esophageal and cardiac carcinoma. World J Gastroenterol 9: 399-403. doi: 10.3748/wjg.v9.i3.399
![]() |
[5] | Liang YJ, Zhou P, Wongba W, et al. (2010) Pulmonary innervation, inflammation and carcinogenesis. Sheng Li Xue Bao 62: 191-195. |
[6] |
Priyanka HP, ThyagaRajan S (2013) Selective modulation of lymphoproliferation and cytokine production via intracellular signaling targets by α1- and α2-adrenoceptors and estrogen in splenocytes. Int Immunopharmacol 17: 774-784. doi: 10.1016/j.intimp.2013.08.020
![]() |
[7] | Meites J, Quadri SK (1987) Neuroendocrine theories of aging. The Encyclopedia of Aging New York: Springer, 474-478. |
[8] |
ThyagaRajan S, MohanKumar PS, Quadri SK (1995) Cyclic changes in the release of norepinephrine and dopamine in the medial basal hypothalamus: effects of aging. Brain Res 689: 122-128. doi: 10.1016/0006-8993(95)00551-Z
![]() |
[9] | ThyagaRajan S, Priyanka HP (2012) Bidirectional communication between the neuroendocrine system and the immune system: relevance to health and diseases. Ann Neurosci 19: 40-46. |
[10] |
Mravec B, Gidron Y, Hulin I (2008) Neurobiology of cancer: interactions between nervous, endocrine and immune systems as a base for monitoring and modulating the tumorigenesis by the brain. Semin Cancer Biol 18: 150-163. doi: 10.1016/j.semcancer.2007.12.002
![]() |
[11] |
Mukhtar RA, Nseyo O, Campbell MJ, et al. (2011) Tumor-associated mac-rophages in breast cancer as potential biomarkers for new treatments and diagnostics. Expert Rev Mol Diagn 11: 91-100. doi: 10.1586/erm.10.97
![]() |
[12] |
Müller A, Homey B, Soto H, et al. (2001) Involvement of chemokine receptors in breast cancer metastasis. Nature 410: 50-56. doi: 10.1038/35065016
![]() |
[13] |
Hanahan D, Coussens LM (2012) Accessories to the crime: functions of cells recruited to the tumor microenvironment. Cancer Cell 21: 309-322. doi: 10.1016/j.ccr.2012.02.022
![]() |
[14] |
Hanahan D, Weinberg RA (2011) Hallmarks of cancer: the next generation. Cell 144: 646-674. doi: 10.1016/j.cell.2011.02.013
![]() |
[15] |
Cole SW, Nagaraja AS, Lutgendorf SK, et al. (2015) Sympathetic nervous system regulation of the tumour microenvironment. Nat Rev Cancer 15: 563-572. doi: 10.1038/nrc3978
![]() |
[16] |
Segerstrom SC, Miller GE (2004) Psychological stress and the human immune system: a meta-analytic study of 30 years of inquiry. Psychol Bull 130: 601-630. doi: 10.1037/0033-2909.130.4.601
![]() |
[17] |
Greten FR, Grivennikov SI (2019) Inflammation and cancer: triggers, mechanisms, and consequences. Immunity 51: 27-41. doi: 10.1016/j.immuni.2019.06.025
![]() |
[18] |
Yang H, Xia L, Chen J, et al. (2019) Stress-glucocorticoid-TSC22D3 axis compromises therapy-induced antitumor immunity. Nat Med 25: 1428-1441. doi: 10.1038/s41591-019-0566-4
![]() |
[19] |
Curtin NM, Boyle NT, Mills KH, et al. (2009) Psychological stress suppresses innate IFN-gamma production via glucocorticoid receptor activation: reversal by the anxiolytic chlordiazepoxide. Brain Behav Immun 23: 535-547. doi: 10.1016/j.bbi.2009.02.003
![]() |
[20] |
Key TJ (1995) Hormones and cancer in humans. Mutat Res 333: 59-67. doi: 10.1016/0027-5107(95)00132-8
![]() |
[21] |
Mravec B, Tibensky M, Horvathova L (2020) Stress and cancer. Part I: Mechanisms mediating the effect of stressors on cancer. J Neuroimmunol 346: 577311. doi: 10.1016/j.jneuroim.2020.577311
![]() |
[22] |
Yin W, Gore AC (2006) Neuroendocrine control of reproductive aging: roles of GnRH neurons. Reproduction 131: 403-414. doi: 10.1530/rep.1.00617
![]() |
[23] | Reed BG, Carr BR (2000) The Normal Menstrual Cycle and the Control of Ovulation. Endotext South Dartmouth (MA): MDText.com, Inc. |
[24] |
Del Río JP, Alliende MI, Molina N, et al. (2018) Steroid Hormones and Their Action in Women's Brains: The Importance of Hormonal Balance. Front Public Health 6: 141. doi: 10.3389/fpubh.2018.00141
![]() |
[25] |
Djahanbakhch O, Ezzati M, Zosmer A (2007) Reproductive ageing in women. J Pathol 211: 219-231. doi: 10.1002/path.2108
![]() |
[26] | Braunwald E, Isselbacher KJ, Petersdorf RG, et al. (1987) Harrison's Principles of Internal Medicine New York: McGraw-Hill, 1818-1837. |
[27] |
Priyanka HP, Nair RS (2020) Neuroimmunomodulation by estrogen in health and disease. AIMS Neurosci 7: 401-417. doi: 10.3934/Neuroscience.2020025
![]() |
[28] |
Sherman BM, West JH, Korenman SG (1976) The menopausal transition: analysis of LH, FSH, estradiol, and progesterone concentrations during menstrual cycles of older women. J Clin Endocrinol Metab 42: 629-636. doi: 10.1210/jcem-42-4-629
![]() |
[29] |
Hall JE, Gill S (2001) Neuroendocrine aspects of aging of aging in women. Endocrinol Metab Clin North Am 30: 631-646. doi: 10.1016/S0889-8529(05)70205-X
![]() |
[30] |
Lang TJ (2004) Estrogen as an immunomodulator. Clin Immunol 113: 224-230. doi: 10.1016/j.clim.2004.05.011
![]() |
[31] |
Salem ML (2004) Estrogen, a double-edged sword: modulation of TH1- and TH2-mediated inflammations by differential regulation of TH1/TH2 cytokine production. Curr Drug Targets Inflamm Allergy 3: 97-104. doi: 10.2174/1568010043483944
![]() |
[32] |
Wise PM, Scarbrough K, Lloyd J, et al. (1994) Neuroendocrine concomitants of reproductive aging. Exp Gerontol 29: 275-283. doi: 10.1016/0531-5565(94)90007-8
![]() |
[33] |
Chakrabarti M, Haque A, Banik NL, et al. (2014) Estrogen receptor agonists for attenuation of neuroinflammation and neurodegeneration. Brain Res Bull 109: 22. doi: 10.1016/j.brainresbull.2014.09.004
![]() |
[34] |
ThyagaRajan S, Madden KS, Teruya B, et al. (2011) Age-associated alterations in sympathetic noradrenergic innervation of primary and secondary lymphoid organs in female Fischer 344 rats. J Neuroimmunol 233: 54-64. doi: 10.1016/j.jneuroim.2010.11.012
![]() |
[35] |
Teilmann SC, Clement CA, Thorup J, et al. (2006) Expression and localization of the progesterone receptor in mouse and human reproductive organs. J Endocrinol 191: 525-535. doi: 10.1677/joe.1.06565
![]() |
[36] |
Butts CL, Shukair SA, Duncan KM, et al. (2007) Progesterone inhibits mature rat dendritic cells in a receptor-mediated fashion. Int Immunol 19: 287-296. doi: 10.1093/intimm/dxl145
![]() |
[37] |
Jones LA, Kreem S, Shweash M, et al. (2010) Differential modulation of TLR3- and TLR4-mediated dendritic cell maturation and function by progesterone. J Immunol 185: 4525-4534. doi: 10.4049/jimmunol.0901155
![]() |
[38] |
Menzies FM, Henriquez FL, Alexander J, et al. (2011) Selective inhibition and augmentation of alternative macrophage activation by progesterone. Immunology 134: 281-291. doi: 10.1111/j.1365-2567.2011.03488.x
![]() |
[39] |
Hardy DB, Janowski BA, Corey DR, et al. (2006) Progesterone receptor plays a major antiinflammatory role in human myometrial cells by antagonism of nuclear factor-κB activation of cyclooxygenase 2 expression. Mol Endocrinol 20: 2724-2733. doi: 10.1210/me.2006-0112
![]() |
[40] |
Arruvito L, Giulianelli S, Flores AC, et al. (2008) NK cells expressing a progesterone receptor are susceptible to progesterone-induced apoptosis. J Immunol 180: 5746-5753. doi: 10.4049/jimmunol.180.8.5746
![]() |
[41] | Piccinni MP, Giudizi MG, Biagiotti R, et al. (1995) Progesterone favors the development of human T helper cells producing TH2-type cytokines and promotes both IL-4 production and membrane CD30 expression in established TH1 cell clones. J Immunol 155: 128-133. |
[42] |
Özdemir BC, Dotto GP (2019) Sex hormones an anticancer immunity. Clin Cancer Res 25: 4603-4610. doi: 10.1158/1078-0432.CCR-19-0137
![]() |
[43] |
Beral V (2003) Breast cancer and hormone-replacement therapy in the Million Women Study. Lancet 362: 419-427. doi: 10.1016/S0140-6736(03)14596-5
![]() |
[44] |
Grady D, Gebretsadik T, Kerlikowske K, et al. (1995) Hormone replacement therapy and endometrial cancer risk: a meta-analysis. Obstet Gynecol 85: 304-313. doi: 10.1016/0029-7844(94)00383-O
![]() |
[45] |
Lacey JV, Mink PJ, Lubin JH, et al. (2002) Menopausal hormone replacement therapy and risk of ovarian cancer. JAMA 288: 334-341. doi: 10.1001/jama.288.3.334
![]() |
[46] |
Fournier A, Berrino F, Clavel-Chapelon F (2008) Unequal risks for breast cancer associated with different hormone replacement therapies results from the E3N cohort study. Breast Cancer Res Treat 107: 103-111. doi: 10.1007/s10549-007-9523-x
![]() |
[47] |
Anderson GL, Limacher M, Assaf AR, et al. (2004) Effects of conjugated equine estrogen in postmenopausal women with hysterectomy: the Women's Health Initiative randomized controlled trial. JAMA 291: 1701-1712. doi: 10.1001/jama.291.14.1701
![]() |
[48] |
Key TJ, Pike MC (1988) The dose-effect relationship between ‘unopposed’ oestrogens and endometrial mitotic rate: its central role in explaining and predicting endometrial cancer risk. Br J Cancer 57: 205-212. doi: 10.1038/bjc.1988.44
![]() |
[49] |
Cook MB, Dawsey SM, Freedman ND, et al. (2009) Sex disparities in cancer incidence by period and age. Cancer Epidemiol Biomarkers Prev 18: 1174-1182. doi: 10.1158/1055-9965.EPI-08-1118
![]() |
[50] |
Cook MB, McGlynn KA, Devesa SS, et al. (2011) Sex disparities in cancer mortality and survival. Cancer Epidemiol. Biomarkers Prev 20: 1629-1637. doi: 10.1158/1055-9965.EPI-11-0246
![]() |
[51] |
Lista P, Straface E, Brunelleschi S, et al. (2011) On the role of autophagy in human diseases: a gender perspective. J Cell Mol Med 15: 1443-1457. doi: 10.1111/j.1582-4934.2011.01293.x
![]() |
[52] |
Lin PY, Sun L, Thibodeaux SR, et al. (2010) B7-H1-dependent sex-related differences in tumor immunity and immunotherapy responses. J Immunol 185: 2747-2753. doi: 10.4049/jimmunol.1000496
![]() |
[53] |
Polanczyk MJ, Hopke C, Vandenbark AA, et al. (2006) Estrogen-mediated immunomodulation involves reduced activation of effector T cells, potentiation of Treg cells, and enhanced expression of the PD-1 costimulatory pathway. J Neurosci Res 84: 370-378. doi: 10.1002/jnr.20881
![]() |
[54] |
Klein SL, Flanagan KL (2016) Sex differences in immune responses. Nature Rev Immunol 16: 626-638. doi: 10.1038/nri.2016.90
![]() |
[55] |
Nance DM, Sanders VM (2007) Autonomic innervation and regulation of the immune system. Brain Behav Immun 21: 736-745. doi: 10.1016/j.bbi.2007.03.008
![]() |
[56] |
Priyanka HP, Pratap UP, Singh RV, et al. (2014) Estrogen modulates β2-adrenoceptor-induced cell-mediated and inflammatory immune responses through ER-α involving distinct intracellular signaling pathways, antioxidant enzymes, and nitric oxide. Cell Immunol 292: 1-8. doi: 10.1016/j.cellimm.2014.08.001
![]() |
[57] |
Priyanka HP, Krishnan HC, Singh RV, et al. (2013) Estrogen modulates in vitro T cell responses in a concentration- and receptor dependent manner: effects on intracellular molecular targets and antioxidant enzymes. Mol Immunol 56: 328-339. doi: 10.1016/j.molimm.2013.05.226
![]() |
[58] |
Pratap UP, Patil A, Sharma HR, et al. (2016) Estrogen-induced neuroprotective and anti-inflammatory effects are dependent on the brain areas of middle-aged female rats. Brain Res Bull 124: 238-253. doi: 10.1016/j.brainresbull.2016.05.015
![]() |
[59] |
Kale P, Mohanty A, Mishra M, et al. (2014) Estrogen modulates neural–immune interactions through intracellular signaling pathways and antioxidant enzyme activity in the spleen of middle-aged ovariectomized female rats. J Neuroimmunol 267: 7-15. doi: 10.1016/j.jneuroim.2013.11.003
![]() |
[60] |
Priyanka HP, Sharma U, Gopinath S, et al. (2013) Menstrual cycle and reproductive aging alters immune reactivity, NGF expression, antioxidant enzyme activities, and intracellular signaling pathways in the peripheral blood mononuclear cells of healthy women. Brain Behav Immun 32: 131-143. doi: 10.1016/j.bbi.2013.03.008
![]() |
[61] |
Ulrich-Lai YM, Herman JP (2009) Neural regulation of endocrine and autoimmune stress responses. Nat Rev Neurosci 10: 397-409. doi: 10.1038/nrn2647
![]() |
[62] |
Herman JP, Flak J, Jankord R (2008) Chronic stress plasticity in the hypothalamic paraventricular nucleus. Prog Brain Res 170: 353-364. doi: 10.1016/S0079-6123(08)00429-9
![]() |
[63] |
Iftikhar A, Islam M, Shepherd S, et al. (2021) Cancer and Stress: Does It Make a Difference to the Patient When These Two Challenges Collide? Cancers (Basel) 13: 163. doi: 10.3390/cancers13020163
![]() |
[64] |
Lin KT, Wang LH (2016) New dimension of glucocorticoids in cancer treatment. Steroids 111: 84-88. doi: 10.1016/j.steroids.2016.02.019
![]() |
[65] |
Antoni MH, Lutgendorf SK, Cole SW, et al. (2006) The influence of bio-behavioural factors on tumour biology: pathways and mechanisms. Nat Rev Cancer 6: 240-248. doi: 10.1038/nrc1820
![]() |
[66] |
Lutgendorf SK, Costanzo E, Siegel S (2007) Psychosocial influences in oncology: An expanded model of biobehavioral mechanisms. Psychoneuroimmunology New York, NY, USA: Academic Press, 869-895. doi: 10.1016/B978-012088576-3/50048-4
![]() |
[67] |
Xie H, Li B, Li L, et al. (2014) Association of increased circulating catecholamine and glucocorticoid levels with risk of psychological problems in oral neoplasm patients. PLoS One 9: e99179. doi: 10.1371/journal.pone.0099179
![]() |
[68] |
Lutgendorf SK, DeGeest K, Dahmoush L, et al. (2011) Social isolation is associated with elevated tumor norepinephrine in ovarian carcinoma patients. Brain Behav Immun 25: 250-255. doi: 10.1016/j.bbi.2010.10.012
![]() |
[69] |
Obeid EI, Conzen SD (2013) The role of adrenergic signaling in breast cancer biology. Cancer Biomark 13: 161-169. doi: 10.3233/CBM-130347
![]() |
[70] |
Mravec B, Horvathova L, Hunakova L (2020) Neurobiology of cancer: the role of β-adrenergic receptor signaling in various tumor environments. Int J Mol Sci 21: 7958. doi: 10.3390/ijms21217958
![]() |
[71] |
Thaker PH, Han LY, Kamat AA, et al. (2006) Chronic stress promotes tumor growth and angiogenesis in a mouse model of ovarian carcinoma. Nat Med 12: 939-944. doi: 10.1038/nm1447
![]() |
[72] |
Lin Q, Wang F, Yang R, et al. (2013) Effect of chronic restraint stress on human colorectal carcinoma growth in mice. PLoS One 8: e61435. doi: 10.1371/journal.pone.0061435
![]() |
[73] |
Landen CN, Lin YG, Armaiz Pena GN, et al. (2007) Neuroendocrine modulation of signal transducer and activator of transcription-3 in ovarian cancer. Cancer Res 67: 10389-10396. doi: 10.1158/0008-5472.CAN-07-0858
![]() |
[74] |
Shang ZJ, Liu K, Liang DF (2009) Expression of beta2-adrenergic receptor in oral squamous cell carcinoma. J Oral Pathol Med 38: 371-376. doi: 10.1111/j.1600-0714.2008.00691.x
![]() |
[75] |
Saul AN, Oberyszyn TM, Daugherty C, et al. (2005) Chronic stress and susceptibility to skin cancer. J Natl Cancer Inst 97: 1760-1767. doi: 10.1093/jnci/dji401
![]() |
[76] |
Ben-Eliyahu S, Page GG, Yirmiya R, et al. (1999) Evidence that stress and surgical interventions promote tumor development by suppressing natural killer cell activity. Int J Cancer 80: 880-888. doi: 10.1002/(SICI)1097-0215(19990315)80:6<880::AID-IJC14>3.0.CO;2-Y
![]() |
[77] |
Ben-Eliyahu S, Yirmiya R, Liebeskind JC, et al. (1991) Stress increases metastatic spread of a mammary tumor in rats: evidence for mediation by the immune system. Brain Behav Immun 5: 193-205. doi: 10.1016/0889-1591(91)90016-4
![]() |
[78] |
Greenfeld K, Avraham R, Benish M, et al. (2007) Immune suppression while awaiting surgery and following it: dissociations between plasma cytokine levels, their induced production, and NK cell cytotoxicity. Brain Behav Immun 21: 503-513. doi: 10.1016/j.bbi.2006.12.006
![]() |
[79] |
Morris N, Moghaddam N, Tickle A, et al. (2018) The relationship between coping style and psychological distress in people with head and neck cancer: A systematic review. Psychooncology 27: 734-747. doi: 10.1002/pon.4509
![]() |
[80] | Nogueira TE, Adorno M, Mendonça E, et al. (2018) Factors associated with the quality of life of subjects with facial disfigurement due to surgical treatment of head and neck cancer. Med Oral Patol Oral Cir Bucal 23: e132-e137. |
[81] |
Hagedoorn M, Molleman E (2006) Facial disfigurement in patients with head and neck cancer: the role of social self-efficacy. Health Psychol 25: 643-647. doi: 10.1037/0278-6133.25.5.643
![]() |
[82] |
Lackovicova L, Gaykema RP, Banovska L, et al. (2013) The time-course of hindbrain neuronal activity varies according to location during either intraperitoneal or subcutaneous tumor growth in rats: Single Fos and dual Fos/dopamine beta-hydroxylase immunohistochemistry. J Neuroimmunol 260: 37-46. doi: 10.1016/j.jneuroim.2013.04.010
![]() |
[83] |
Horvathova L, Tillinger A, Padova A, et al. (2020) Changes in gene expression in brain structures related to visceral sensation, autonomic functions, food intake, and cognition in melanoma-bearing mice. Eur J Neurosci 51: 2376-2393. doi: 10.1111/ejn.14661
![]() |
[84] |
Kin NW, Sanders VM (2006) It takes nerve to tell T and B cells what to do. J Leukocyte Biol 79: 1093-1104. doi: 10.1189/jlb.1105625
![]() |
[85] |
Straub RH (2004) Complexity of the bi-directional neuroimmune junction in the spleen. Trends Pharmacol Sci 25: 640-646. doi: 10.1016/j.tips.2004.10.007
![]() |
[86] |
Nance DM, Sanders VM (2007) Autonomic innervation and regulation of the immune system (1987–2007). Brain Behav Immun 21: 736-745. doi: 10.1016/j.bbi.2007.03.008
![]() |
[87] |
Madden KS (2003) Catecholamines, sympathetic innervation, and immunity. Brain Behav Immun 17: S5-10. doi: 10.1016/S0889-1591(02)00059-4
![]() |
[88] |
ThyagaRajan S, Felten DL (2002) Modulation of neuroendocrine--immune signaling by L-deprenyl and L-desmethyldeprenyl in aging and mammary cancer. Mech Ageing Dev 123: 1065-1079. doi: 10.1016/S0047-6374(01)00390-6
![]() |
[89] |
Pratap U, Hima L, Kannan T, et al. (2020) Sex-Based Differences in the Cytokine Production and Intracellular Signaling Pathways in Patients With Rheumatoid Arthritis. Arch Rheumatol 35: 545-557. doi: 10.46497/ArchRheumatol.2020.7481
![]() |
[90] |
Hima L, Patel M, Kannan T, et al. (2020) Age-associated decline in neural, endocrine, and immune responses in men and women: Involvement of intracellular signaling pathways. J Neuroimmunol 345: 577290. doi: 10.1016/j.jneuroim.2020.577290
![]() |
[91] |
Chen DS, Mellman I (2013) Oncology meets immunology: the cancer-immunity cycle. Immunity 39: 1-10. doi: 10.1016/j.immuni.2013.07.012
![]() |
[92] |
Schreiber RD, Old LJ, Smyth MJ (2011) Cancer immunoediting: integrating immunity's roles in cancer suppression and promotion. Science 331: 1565-1570. doi: 10.1126/science.1203486
![]() |
[93] |
Sanders VM (1995) The role of adrenoceptor-mediated signals in the modulation of lymphocyte function. Adv Neuroimmunol 5: 283-298. doi: 10.1016/0960-5428(95)00019-X
![]() |
[94] |
Madden KS, Felten DL (1995) Experimental basis for neural-immune interactions. Physiol Rev 75: 77-106. doi: 10.1152/physrev.1995.75.1.77
![]() |
[95] |
Madden KS (2003) Catecholamines, sympathetic innervation, and immunity. Brain Behav Immun 17: S5-10. doi: 10.1016/S0889-1591(02)00059-4
![]() |
[96] |
Callahan TA, Moynihan JA (2002) The effects of chemical sympathectomy on T-cell cytokine responses are not mediated by altered peritoneal exudate cell function or an inflammatory response. Brain Behav Immun 16: 33-45. doi: 10.1006/brbi.2000.0618
![]() |
[97] |
Madden KS, Felten SY, Felten DL, et al. (1989) Sympathetic neural modulation of the immune system. I. Depression of T cell immunity in vivo and vitro following chemical sympathectomy. Brain Behav Immun 3: 72-89. doi: 10.1016/0889-1591(89)90007-X
![]() |
[98] |
Alaniz RC, Thomas SA, Perez-Melgosa M, et al. (1999) Dopamine beta-hydroxylase deficiency impairs cellular immunity. Proc Natl Acad Sci U S A 96: 2274-2278. doi: 10.1073/pnas.96.5.2274
![]() |
[99] |
Pongratz G, McAlees JW, Conrad DH, et al. (2006) The level of IgE produced by a B cell is regulated by norepinephrine in a p38 MAPK- and CD23-dependent manner. J Immunol 177: 2926-2938. doi: 10.4049/jimmunol.177.5.2926
![]() |
[100] |
Chen F, Zhuang X, Lin L, et al. (2015) New horizons in tumor microenvironment biology: challenges and opportunities. BMC Med 13: 45. doi: 10.1186/s12916-015-0278-7
![]() |
[101] |
Wang M, Zhao J, Zhang L, et al. (2017) Role of tumor microenvironment in tumorigenesis. J Cancer 8: 761-773. doi: 10.7150/jca.17648
![]() |
[102] |
Del Prete A, Schioppa T, Tiberio L, et al. (2017) Leukocyte trafficking in tumor microenvironment. Curr Opin Pharmacol 35: 40-47. doi: 10.1016/j.coph.2017.05.004
![]() |
[103] |
Jiang Y, Li Y, Zhu B (2015) T-cell exhaustion in the tumor microenvironment. Cell Death Dis 6: e1792. doi: 10.1038/cddis.2015.162
![]() |
[104] |
Maimela NR, Liu S, Zhang Y (2019) Fates of CD8+ T cells in tumor microenvironment. Comput Struct Biotechnol J 17: 1-13. doi: 10.1016/j.csbj.2018.11.004
![]() |
[105] |
Zhang Z, Liu S, Zhang B, et al. (2020) T Cell Dysfunction and Exhaustion in Cancer. Front Cell Dev Biol 8: 17. doi: 10.3389/fcell.2020.00017
![]() |
[106] |
Gonzalez H, Robles I, Werb Z (2018) Innate and acquired immune surveillance in the postdissemination phase of metastasis. FEBS J 285: 654-664. doi: 10.1111/febs.14325
![]() |
[107] |
Komohara Y, Fujiwara Y, Ohnishi K, et al. (2016) Tumor-associated macrophages: Potential therapeutic targets for anti-cancer therapy. Adv Drug Delivery Rev 99: 180-185. doi: 10.1016/j.addr.2015.11.009
![]() |
[108] |
Mantovani A, Marchesi F, Malesci A, et al. (2017) Tumour-associated macrophages as treatment targets in oncology. Nat Rev Clin Oncol 14: 399-416. doi: 10.1038/nrclinonc.2016.217
![]() |
[109] |
Linde N, Lederle W, Depner S, et al. (2012) Vascular endothelial growth factor-induced skin carcinogenesis depends on recruitment and alternative activation of macrophages. J Pathol 227: 17-28. doi: 10.1002/path.3989
![]() |
[110] |
Komohara Y, Jinushi M, Takeya M (2014) Clinical significance of macrophage heterogeneity in human malignant tumors. Cancer Sci 105: 1-8. doi: 10.1111/cas.12314
![]() |
[111] |
Ruffell B, Coussens LM (2015) Macrophages and therapeutic resistance in cancer. Cancer Cell 27: 462-472. doi: 10.1016/j.ccell.2015.02.015
![]() |
[112] |
Noy R, Pollard JW (2014) Tumor-associated macrophages: from mechanisms to therapy. Immunity 41: 49-61. doi: 10.1016/j.immuni.2014.06.010
![]() |
[113] |
Long KB, Gladney WL, Tooker GM, et al. (2016) IFN-γ and CCL2 Cooperate to Redirect Tumor-Infiltrating Monocytes to Degrade Fibrosis and Enhance Chemotherapy Efficacy in Pancreatic Carcinoma. Cancer Discov 6: 400-413. doi: 10.1158/2159-8290.CD-15-1032
![]() |
[114] |
Muz B, de la Puente P, Azab F, et al. (2015) The role of hypoxia in cancer progression, angiogenesis, metastasis, and resistance to therapy. Hypoxia (Auckl) 3: 83-92. doi: 10.2147/HP.S93413
![]() |
[115] |
Zhang CC, Sadek HA (2014) Hypoxia and metabolic properties of hematopoietic stem cells. Antioxid Redox Signal 20: 1891-1901. doi: 10.1089/ars.2012.5019
![]() |
[116] |
Henze AT, Mazzone M (2016) The impact of hypoxia on tumor-associated macrophages. J Clin Invest 126: 3672-3679. doi: 10.1172/JCI84427
![]() |
[117] |
Chen P, Zuo H, Xiong H, et al. (2017) Gpr132 sensing of lactate mediates tumor-macrophage interplay to promote breast cancer metastasis. Proc Natl Acad Sci 114: 580-585. doi: 10.1073/pnas.1614035114
![]() |
[118] |
Albo D, Akay CL, Marshall CL, et al. (2011) Neurogenesis in colorectal cancer is a marker of aggressive tumor behavior and poor outcomes. Cancer 117: 4834-4845. doi: 10.1002/cncr.26117
![]() |
[119] |
Kamiya A, Hiyama T, Fujimura A, et al. (2021) Sympathetic and parasympathetic innervation in cancer: therapeutic implications. Clin Auton Res 31: 165-178. doi: 10.1007/s10286-020-00724-y
![]() |
[120] |
Ceyhan GO, Schäfer KH, Kerscher AG, et al. (2010) Nerve growth factor and artemin are paracrine mediators of pancreatic neuropathy in pancreatic adenocarcinoma. Ann Surg 251: 923-931. doi: 10.1097/SLA.0b013e3181d974d4
![]() |
[121] |
Zhao CM, Hayakawa Y, Kodama Y, et al. (2014) Denervation suppresses gastric tumorigenesis. Sci Transl Med 6: 250ra115. doi: 10.1126/scitranslmed.3009569
![]() |
[122] |
Liebig C, Ayala G, Wilks JA, et al. (2009) Perineural invasion in cancer: a review of the literature. Cancer 115: 3379-3391. doi: 10.1002/cncr.24396
![]() |
[123] |
Dai H, Li R, Wheeler T, et al. (2007) Enhanced survival in perineural invasion of pancreatic cancer: an in vitro approach. Hum Pathol 38: 299-307. doi: 10.1016/j.humpath.2006.08.002
![]() |
[124] |
Liebig C, Ayala G, Wilks J, et al. (2009) Perineural invasion is an independent predictor of outcome in colorectal cancer. J Clin Oncol 27: 5131-5137. doi: 10.1200/JCO.2009.22.4949
![]() |
[125] |
Conceição F, Sousa DM, Paredes J, et al. (2021) Sympathetic activity in breast cancer and metastasis: partners in crime. Bone Res 9: 9. doi: 10.1038/s41413-021-00137-1
![]() |
[126] |
Mauffrey P, Tchitchek N, Barroca V, et al. (2019) Progenitors from the central nervous system drive neurogenesis in cancer. Nature 569: 672-678. doi: 10.1038/s41586-019-1219-y
![]() |
[127] |
Ruscica M, Dozio E, Motta M, et al. (2007) Role of neuropeptide Y and its receptors in the progression of endocrine-related cancer. Peptides 28: 426-434. doi: 10.1016/j.peptides.2006.08.045
![]() |
[128] |
Hayakawa Y, Sakitani K, Konishi M, et al. (2017) Nerve Growth Factor Promotes Gastric Tumorigenesis through Aberrant Cholinergic Signaling. Cancer Cell 31: 21-34. doi: 10.1016/j.ccell.2016.11.005
![]() |
[129] |
Barabutis N (2021) Growth Hormone Releasing Hormone in Endothelial Barrier Function. Trends Endocrinol Metab 32: 338-340. doi: 10.1016/j.tem.2021.03.001
![]() |
[130] |
ThyagaRajan S, Madden KS, Kalvass JC, et al. (1998) L-deprenyl-induced increase in IL-2 and NK cell activity accompanies restoration of noradrenergic nerve fibers in the spleens of old F344 rats. J Neuroimmunol 92: 9-21. doi: 10.1016/S0165-5728(98)00039-3
![]() |
[131] |
Zumoff B (1998) Does postmenopausal estrogen administration increase the risk of breast cancer? Contributions of animal, biochemical, and clinical investigative studies to a resolution of the controversy. Proc Soc Exp Biol Med 217: 30-37. doi: 10.3181/00379727-217-44202
![]() |
[132] |
Hollingsworth AB, Lerner MR, Lightfoot SA, et al. (1998) Prevention of DMBA-induced rat mammary carcinomas comparing leuprolide, oophorectomy, and tamoxifen. Breast Cancer Res Treat 47: 63-70. doi: 10.1023/A:1005872132373
![]() |
[133] |
Sun G, Wu L, Sun G, et al. (2021) WNT5a in Colorectal Cancer: Research Progress and Challenges. Cancer Manag Res 13: 2483-2498. doi: 10.2147/CMAR.S289819
![]() |
[134] |
Schlange T, Matsuda Y, Lienhard S, et al. (2007) Autocrine WNT signaling contributes to breast cancer cell proliferation via the canonical WNT pathway and EGFR transactivation. Breast Cancer Res 9: R63. doi: 10.1186/bcr1769
![]() |
[135] |
Sun X, Bernhardt SM, Glynn DJ, et al. (2021) Attenuated TGFB signalling in macrophages decreases susceptibility to DMBA-induced mammary cancer in mice. Breast Cancer Res 23: 39. doi: 10.1186/s13058-021-01417-8
![]() |
[136] |
Heasley L (2001) Autocrine and paracrine signaling through neuropeptide receptors in human cancer. Oncogene 20: 1563-1569. doi: 10.1038/sj.onc.1204183
![]() |
[137] |
Cuttitta F, Carney DN, Mulshine J, et al. (1985) Bombesin-like peptides can function as autocrine growth factors in human small-cell lung cancer. Nature 316: 823-826. doi: 10.1038/316823a0
![]() |
[138] |
Moody TW, Pert CB, Gazdar AF, et al. (1981) Neurotensin is produced by and secreted from classic small cell lung cancer cells. Science 214: 1246-1248. doi: 10.1126/science.6272398
![]() |
[139] | Cardona C, Rabbitts PH, Spindel ER, et al. (1991) Production of neuromedin B and neuromedin B gene expression in human lung tumor cell lines. Cancer Res 51: 5205-5211. |
[140] |
Sun B, Halmos G, Schally AV, et al. (2000) Presence of receptors for bombesin/gastrin-releasing peptide and mRNA for three receptor subtypes in human prostate cancers. Prostate 42: 295-303. doi: 10.1002/(SICI)1097-0045(20000301)42:4<295::AID-PROS7>3.0.CO;2-B
![]() |
[141] | Markwalder R, Reubi JC (1999) Gastrin-releasing peptide receptors in the human prostate: relation to neoplastic transformation. Cancer Res 59: 1152-1159. |
[142] |
Moody TW, Pert CB, Gazdar AF, et al. (1981) Neurotensin is produced by and secreted from classic small cell lung cancer cells. Science 214: 1246-1248. doi: 10.1126/science.6272398
![]() |
[143] |
Goetze JP, Nielsen FC, Burcharth F, et al. (2000) Closing the gastrin loop in pancreatic carcinoma: Co-expression of gastrin and its receptor in solid human pancreatic adenocarcinoma. Cancer 88: 2487-2494. doi: 10.1002/1097-0142(20000601)88:11<2487::AID-CNCR9>3.0.CO;2-E
![]() |
[144] |
Blackmore M, Hirst B (1992) Autocrine stimulation of growth of AR4-2J rat pancreatic tumour cells by gastrin. Br J Cancer 66: 32-38. doi: 10.1038/bjc.1992.212
![]() |
[145] | Smith JP, Liu G, Soundararajan V, et al. (1994) Identification and characterization of CCK-B/gastrin receptors in human pancreatic cancer cell lines. Am J Physiol 266: R277-283. |
[146] |
Seethalakshmi L, Mitra SP, Dobner PR, et al. (1997) Neurotensin receptor expression in prostate cancer cell line and growth effect of NT at physiological concentrations. Prostate 31: 183-192. doi: 10.1002/(SICI)1097-0045(19970515)31:3<183::AID-PROS7>3.0.CO;2-M
![]() |
[147] |
Kawaguchi K, Sakurai M, Yamamoto Y, et al. (2019) Alteration of specific cytokine expression patterns in patients with breast cancer. Sci Rep 9: 2924. doi: 10.1038/s41598-019-39476-9
![]() |
[148] |
Smyth MJ, Cretney E, Kershaw MH, et al. (2004) Cytokines in cancer immunity and immunotherapy. Immunol Rev 202: 275-293. doi: 10.1111/j.0105-2896.2004.00199.x
![]() |
[149] |
Wang X, Lin Y (2008) Tumor necrosis factor and cancer, buddies or foes? Acta Pharmacol Sin 29: 1275-1288. doi: 10.1111/j.1745-7254.2008.00889.x
![]() |
[150] |
Ohri CM, Shikotra A, Green RH, et al. (2010) Tumour necrosis factor-alpha expression in tumour islets confers a survival advantage in non-small cell lung cancer. BMC Cancer 10: 323. doi: 10.1186/1471-2407-10-323
![]() |
[151] |
Woo CH, Eom YW, Yoo MH, et al. (2000) Tumor necrosis factor-α generates reactive oxygen species via a cytosolic phospholipase A2-linked cascade. J Biol Chem 275: 32357-32362. doi: 10.1074/jbc.M005638200
![]() |
[152] |
Suganuma M, Watanabe T, Yamaguchi K, et al. (2012) Human gastric cancer development with TNF-α-inducing protein secreted from Helicobacter pylori. Cancer Lett 322: 133-138. doi: 10.1016/j.canlet.2012.03.027
![]() |
[153] |
Cai X, Cao C, Li J, et al. (2017) Inflammatory factor TNF-α promotes the growth of breast cancer via the positive feedback loop of TNFR1/NF-κB (and/or p38)/p-STAT3/HBXIP/TNFR1. Oncotarget 8: 58338-58352. doi: 10.18632/oncotarget.16873
![]() |
[154] |
Fiorentino DF, Bond MW, Mosmann TR (1989) Two types of mouse T helper cell. IV. Th2 clones secrete a factor that inhibits cytokine production by Th1 clones. J Exp Med 170: 2081-2095. doi: 10.1084/jem.170.6.2081
![]() |
[155] |
Ikeguchi M, Hatada T, Yamamoto M, et al. (2009) Serum interleukin-6 and -10 levels in patients with gastric cancer. Gastric Cancer 12: 95-100. doi: 10.1007/s10120-009-0509-8
![]() |
[156] |
Hassuneh MR, Nagarkatti M, Nagarkatti PS (2013) Role of interleukin-10 in the regulation of tumorigenicity of a T cell lymphoma. Leuk Lymphoma 54: 827-834. doi: 10.3109/10428194.2012.726721
![]() |
[157] |
Hodge DR, Hurt EM, Farrar WL (2005) The role of IL-6 and STAT3 in inflammation and cancer. Eur J Cancer 41: 2502-2512. doi: 10.1016/j.ejca.2005.08.016
![]() |
[158] |
Kim SY, Kang JW, Song X, et al. (2013) Role of the IL-6-JAK1-STAT3-Oct-4 pathway in the conversion of non-stem cancer cells into cancer stem-like cells. Cell Signaling 25: 961-969. doi: 10.1016/j.cellsig.2013.01.007
![]() |
[159] |
Mravec B, Horvathova L, Cernackova A (2019) Hypothalamic Inflammation at a Crossroad of Somatic Diseases. Cell Mol Neurobiol 39: 11-29. doi: 10.1007/s10571-018-0631-4
![]() |
[160] |
Morrison CD, Parvani JG, Schiemann WP (2013) The relevance of the TGF-β Paradox to EMT-MET programs. Cancer Lett 341: 30-40. doi: 10.1016/j.canlet.2013.02.048
![]() |
[161] |
Bierie B, Moses HL (2006) TGF-β and cancer. Cytokine Growth Factor Rev 17: 29-40. doi: 10.1016/j.cytogfr.2005.09.006
![]() |
[162] |
Levy L, Hill CS (2006) Alterations in components of the TGF-β superfamily signaling pathways in human cancer. Cytokine Growth Factor Rev 17: 41-58. doi: 10.1016/j.cytogfr.2005.09.009
![]() |