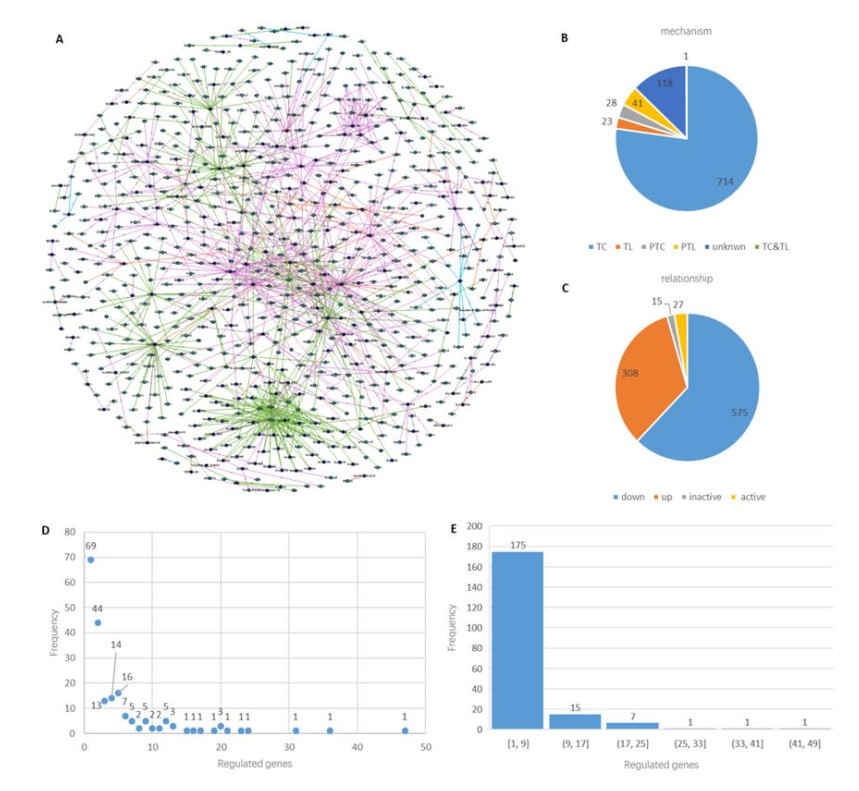
This paper presents some recent views on the aspects of vitamin D levels in relation to the COVID-19 infections and analyzes the relationship between the prevalence rates of vitamin D deficiency and COVID-19 death rates per million of various countries in Europe and Asia using the data from the PubMed database. The paper also discusses a new mathematical model of time-delay interactions between the body's immune healthy cells, infected cells, and virus particles with the effect of vitamin D levels. The model can be used to monitor the timely progression of healthy immune cells with the effects of the levels of vitamin D and probiotics supplement. It also can help to predict when the infected cells and virus particles free state can ever be reached as time progresses. The consideration of the time delay in the modeling due to effects of the infected cells or virus particles and the growth of healthy cells is also an important factor that can significantly change the outcomes of the body's immune cells as well as the infections.
Citation: Hoang Pham. Analyzing the relationship between the vitamin D deficiency and COVID-19 mortality rate and modeling the time-delay interactions between body's immune healthy cells, infected cells, and virus particles with the effect of vitamin D levels[J]. Mathematical Biosciences and Engineering, 2022, 19(9): 8975-9004. doi: 10.3934/mbe.2022417
[1] | Geovanni Alberto Ruiz-Romero, Carolina Álvarez-Delgado . Effects of estrogens in mitochondria: An approach to type 2 diabetes. AIMS Molecular Science, 2024, 11(1): 72-98. doi: 10.3934/molsci.2024006 |
[2] | Fumiaki Uchiumi, Makoto Fujikawa, Satoru Miyazaki, Sei-ichi Tanuma . Implication of bidirectional promoters containing duplicated GGAA motifs of mitochondrial function-associated genes. AIMS Molecular Science, 2014, 1(1): 1-26. doi: 10.3934/molsci.2013.1.1 |
[3] | Naba Hasan, Waleem Ahmad, Feroz Alam, Mahboob Hasan . Ferroptosis-molecular mechanisms and newer insights into some diseases. AIMS Molecular Science, 2023, 10(1): 22-36. doi: 10.3934/molsci.2023003 |
[4] | Jian Zou, Fulton T. Crews . Glutamate/NMDA excitotoxicity and HMGB1/TLR4 neuroimmune toxicity converge as components of neurodegeneration. AIMS Molecular Science, 2015, 2(2): 77-100. doi: 10.3934/molsci.2015.2.77 |
[5] | Fumiaki Uchiumi, Akira Sato, Masashi Asai, Sei-ichi Tanuma . An NAD+ dependent/sensitive transcription system: Toward a novel anti-cancer therapy. AIMS Molecular Science, 2020, 7(1): 12-28. doi: 10.3934/molsci.2020002 |
[6] | Yutaka Takihara, Ryuji Otani, Takuro Ishii, Shunsuke Takaoka, Yuki Nakano, Kaori Inoue, Steven Larsen, Yoko Ogino, Masashi Asai, Sei-ichi Tanuma, Fumiaki Uchiumi . Characterization of the human IDH1 gene promoter. AIMS Molecular Science, 2023, 10(3): 186-204. doi: 10.3934/molsci.2023013 |
[7] | Amena W. Smith, Swapan K. Ray, Arabinda Das, Kenkichi Nozaki, Baerbel Rohrer, Naren L. Banik . Calpain inhibition as a possible new therapeutic target in multiple sclerosis. AIMS Molecular Science, 2017, 4(4): 446-462. doi: 10.3934/molsci.2017.4.446 |
[8] | Dora Brites . Cell ageing: a flourishing field for neurodegenerative diseases. AIMS Molecular Science, 2015, 2(3): 225-258. doi: 10.3934/molsci.2015.3.225 |
[9] | Giulia Ambrosi, Pamela Milani . Endoplasmic reticulum, oxidative stress and their complex crosstalk in neurodegeneration: proteostasis, signaling pathways and molecular chaperones. AIMS Molecular Science, 2017, 4(4): 424-444. doi: 10.3934/molsci.2017.4.424 |
[10] | Tsuyoshi Inoshita, Yuzuru Imai . Regulation of vesicular trafficking by Parkinson's disease-associated genes. AIMS Molecular Science, 2015, 2(4): 461-475. doi: 10.3934/molsci.2015.4.461 |
This paper presents some recent views on the aspects of vitamin D levels in relation to the COVID-19 infections and analyzes the relationship between the prevalence rates of vitamin D deficiency and COVID-19 death rates per million of various countries in Europe and Asia using the data from the PubMed database. The paper also discusses a new mathematical model of time-delay interactions between the body's immune healthy cells, infected cells, and virus particles with the effect of vitamin D levels. The model can be used to monitor the timely progression of healthy immune cells with the effects of the levels of vitamin D and probiotics supplement. It also can help to predict when the infected cells and virus particles free state can ever be reached as time progresses. The consideration of the time delay in the modeling due to effects of the infected cells or virus particles and the growth of healthy cells is also an important factor that can significantly change the outcomes of the body's immune cells as well as the infections.
With the advent of technologies allowing for large-scale, high throughput data, a much clearer understanding of the genomic mechanisms behind gene regulation have been gained. The scientists found that there are unexpected far more noncoding RNAs comparing with protein-coding genes and, and these noncoding regions play important roles in determining the complexity observed in the human genome [1,2]. Within these noncoding regions, long noncoding RNAs (lncRNAs), which are functionally defined as noncoding regions of RNA that are at least 200 base-pairs in length, have attracted lots of attention. Certain lncRNAs appear to act locally, while others have more distal regulatory effects, even acting across multiple chromosomes [3]. Many studies have identified specific functions of particular lncRNAs, including embryonic mechanisms, cell cycle functions, innate immunity, and disease processes. However, there are still thousands of lncRNAs have no identified functions [1,3,4,5,6]. Some studies have been performed that produce relatively few numbers of lncRNA functions [7], and have shown that the function of lncRNAs is highly cell-type-specific: one lncRNA may inhibit particular genes in one type of cell while promoting the same gene in another. This phenomenon makes it even more difficult to identify lncRNA functions on a large scale. Due to this specificity, researchers propose that future lncRNA studies should be performed on specific cell types to identify particular regulatory mechanisms.
One of the most prominent and intriguing applications of lncRNA regulatory investigation comes from cancer studies [8,9]. It has been shown that lncRNAs appear to have high connectivity with numerous diseases, especially cancer. Because of the highly cell type-specific nature of lncRNA regulatory functions and the irregularity of cancer cell genetic information, studying lncRNA regulation in specific cancer types may provide promising insight into specific genomic regulations of common cancer cells. In a few documented cases, specific lncRNAs have been shown to be significantly differentially expressed in specific cancer types, such as prostate cancer and breast cancer [1]. For these reasons, it seems appropriate to further investigate lncRNA-gene interactions in particular cancer cells.
The wealth of gene expression datasets available provides an opportunity to computationally identify co-expressed gene modules(CEMs), each of which is defined as a highly structured expression pattern on a specific gene set [10,11]. These CEMs tend to be functionally related or co-regulated by the same transcriptional regulatory signals (e.g., transcription factors, lncRNA and so on) under a specific condition or in a particular disease cell type. Overall, successful derivation of the CEMs may grant a higher-level interpretation of large-scale gene expression data, improve functional annotation of condition-specific gene activities, facilitate inference of gene regulatory relationships, hence, provide a better mechanism level understanding of complex diseases.
The computational identification of CEMs can be solved by a biclustering approach [12], which is a two-dimensional data mining technique that simultaneously identifies co-expressed genes under a subset of conditions. a high proportion of enriched biclusters on real datasets. Within this study, we try to identify new lncRNA-gene interactions and transcription factor-lncRNA partnerships from cancer RNA-seq data using a biclustering approach. The biclustering method will allow for the identification of particular expression patterns across multiple datasets, indicating networks of lncRNA and gene interactions. This developed method will also provide a framework for future lncRNA interaction studies. We applied this method on two sets of TCGA breast cancer RNA-seq data to generated CEMs based on known lncRNA-gene interactions. Then, the predicted CEMs are linked to lncRNA by a statistic p-value and the new lncRNA-gene relationship are generated. The evaluation on the predicted results showed that the pipeline can find some target genes for given lncRNA, and meanwhile the performance still has some space to be improved. We further conducted a TF motif analysis on the predicted CEMs and provide potential regulation cooperation between TFs and lncRNAs. The related original data with codes, results and supplementary data can be downloaded on https://github.com/IvesG/sGavin.git.
Two sets of TCGA (The Cancer Genome Atlas) breast cancer RNA-seq data, one from the normal cell (referred as normal data) and the other from tumor cell (referred as tumor data) were downloaded from https://portal.gdc.cancer.gov/. The normal and tumor data consist of 113 and 1091 samples, respectively. And of the 113 normal samples, 112 of them are from the same patient among the tumors. Both datasets contain 60,483 genes, among which there are 19,824 protein-coding genes and 7,399 long intergenic noncoding RNAs (lincRNAs) genes. The RNA-seq data are all Upper Quartile normalized FPKM (UQ-FPKM) values.
A total of 1,081 experimentally validated lncRNA-associated regulatory entries were downloaded from LncReg [13], describing the comprehensive regulatory relationships among 258 lncRNAs and 571 genes. All these relationships were manually collected from PubMed with focus on the data generated by laboratory methods, and can be categorized into up/down/active/inactive based on regulatory relationships or transcription/post-transcription/translation/post-translation based on regulatory mechanisms.
As we focus on lncRNA-gene interactions, the relationships downloaded from LncReg were filtered to retain only relationships describing genes regulated by lncRNAs with specified species information (constrained to Homo sapiens and Mus musculus), resulting 925 relationships in total for the downstream analysis, covering interactions between 309 unique human genes and 103 human lncRNAs, as well as between 199 mouse genes and 100 mouse lncRNAs. It is noteworthy that these 925 relationships include 28 post-transcriptional regulations, 41 post-translational regulations, 714 transcriptional regulations, 23 translational regulations, 1 transcriptional & translational regulation and 118 unspecified relationships.
As the table from LncReg [13] only provides gene symbols, while the RNA-seq dataset uses Ensembl ID as gene's identifiers, we use Ensembl BioMart [14] to match gene symbols with Ensembl IDs for all the genes and lncRNAs. Then we got orthologous genes between mouse and human also using BioMart; we found orthologous human genes for all 199 mouse genes, and 38 overlapped with original human genes. For convenience, we recorded human genes, mouse genes that don't overlap with human genes, human lncRNAs and mouse lncRNAs that don't overlap with human lncRNAs as HG, MG, HL, and ML, respectively.
We combined the normal and tumor RNA-seq dataset together, then extracted expression values for all the HG, MG, HL, ML, protein-coding genes (PC, the remaining protein-coding genes except HG and MG) and lincRNAs (linc, the remaining lincRNA except HL and ML). Taking the genes as rows and the conditions as columns, we obtained the RNA-seq expression matrix on which biclustering will be performed to detect CEMs.
QUBIC is a biclustering analysis tool designed for co-expression analyses of genes based on their gene-expression patterns under multiple conditions. The software can generally identify all statistically significant groups, or biclusters, of genes with similar expression patterns under at least a specific number of experimental conditions, which tend to be more sensitive and more specific than other biclustering tools [15]. We use a quantile-based discretization method of QUBIC to generate a qualitative representing matrix for the RNA-seq expression matrix. Then we extracted the rows of known lncRNA regulated HG and MG from this representing matrix as seed 1 and HG, MG, HL, and ML rows as seed 2. Next bi-clustering analysis was performed on these two seeds to predict co-expressed gene modules (CEMs) in the qualitative representing matrix, respectively.
For an identified CEMs, we calculated the P-value of a bicluster enriched with genes regulated by a lncRNA using the hypergeometric function [16],
Pr(r|N,K,n)=(Kr)(N−Kn−r)(Nn) |
where r is the number of genes in a CEMs (with size n) that regulated by certain lncRNA, N is the total number of known lncRNA regulated genes in the whole genome, K is the number of genes regulated by that lncRNA in the whole genome.
We assumed that, if the known target genes of a given lncRNA are highly covered by a CEM with a significant p-value, the other genes in this CEM have high possibilities regulated by the given lncRNA. Thus, we used the smallest P-value for all possible lncRNAs as the p-value of the current bicluster and the relationships between lncRNA and genes in the bicluster are predicted.
To evaluate the performance of the new methods on the prediction of new relationships between lncRNA and genes, we randomly separate seed2 into two parts with equal size named seedpart1 and seedpart2, for multiple times. Then bi-clustering analysis will be performed on seedpart2 to predict co-expressed gene modules (CEMs). For seedpart1 we find its part which is covered by co-expressed gene modules (CEMs) from seedpart2. We calculate the cover ratios by the size of seedpart1 to be divided by the size of the covered part by CEMs generated from seedpart2. Also, we calculate the p-values for the coverage rates to present the statistical significance of them.
We choose several significant CEMs with sizes or conditions below 100, to conducted TF motif analysis. The promoter regions of the corresponding genes are inputted into the sub-routine findMotifs.pl of Homer [17], respectively. The script findMotifs.pl can firstly search for the upstream promoter sequences of a certain length automatically, and then perform motif finding on the promoters. For each run of findMotifs.pl on the datasets, we let the program output at most 5 top-ranking motifs, i.e. there will be up to 5 motifs discovered by findMotifs.pl for each CEMs. To evaluate the validity of the discovered motifs, findMotifs.pl automatically compares the similarity between the discovered motif profiles and the motif profiles archived in JASPAR [18] v2018 (http://jaspar.genereg.net/) under its default parameter setting. For each discovered motif having similarity with at least one motif archived in JASPAR, we present its motif logo as well as the information of its most similar motif in JASPAR.
All the known interactions between lncRNAs and genes are showcased in Figure 1A. The related data can be download from https://github.com/IvesG/sGavin.git data/LncReg0419 and more details are written in data/readme.txt. In figure 1A, dark-blue nodes represent LncRNAs, light-blue nodes represent proteins, pink edges represent interactions documented in Homo, green edges represent interactions documented in Mus, orange edges represent interactions documented in both Homo and Mus. Meanwhile, there are some labels on the edges, categorized based on regulatory mechanisms including PTL (post-translational regulation), TC (transcriptional regulation), PTC (post-transcriptional regulation), TL (translational regulation), and NS (not sure). The distribution above is displayed in Figure 1B and nearly three fourth (714/925) of them are identified at the transcriptional level. Other labels on the edges are categorized based on regulatory relationships including down, up, active and inactive. The distribution above is displayed in Figure 1C. The down relationships (575) are more than up relationships (308), and the proportion of active/inactive is scarce (4.5%).
Figure 1E showed the distribution of a number of genes regulated by each lncRNA. It can be found that most lncRNA (~78%) regulate less than 5 genes. To show the specific details of the number of genes regulated by each lncRNA, Figure 1D is made, each point in the Figure 1D reflect the number of lncRNA (horizontal coordinate) that regulate certain number of genes (longitudinal coordinates) e.g. the point with coordinate (4, 14) in Figure 1D indicate that there are 14 lncRNA and each of them regulate 4 genes. The lncRNA that regulate more genes in Figure 1D belongs to the more concentrated parts in Figure 1(A).
With the quantile-based discretization method and biclustering analysis, there are some co-expressed gene modules (CEMs) are found. The details of the way we identify CEMs are showcased in Figure 2C. Figure 2A shows the number of co-expressed gene modules(CEMs) we have got from seed1 and seed2 processed by max(min [19])-based (QUBIC1.0, [15,20]) and KL-based bi-clustering analysis (QUBIC2.0 [21]) respectively. And the distributions of numbers of genes and conditions for each CEM can be found in Figure 2D and Figure 2E. For instance, label seed1_1genes represent that Qubic1.0 is performed on seed1. To better illustrate the distributions, we have constrained the number of each gene and the number of each condition below 100 (in Figure 2B). It is found that the KL-based biclustering method tends to generate CEMs that contain fewer genes while more conditions than max(min [22])-based biclustering method from Figure 2D and Figure 2E. The difference between the results (whether the distribution of genes sizes and condition sizes or the number of CEMs that we have predicted) we get from seed1 and seed2 is subtle.
The proportion of CEMs that have significant P-values (below a pre-selected P-value cutoff) as well as proportions of the number of unique enriched lncRNA in each bicluster that belong to certain categories (i.e., number of lncRNA = 2, 3 or > 3) are calculated and shown in Figure 3A and Figure 3B. In the figures, Seed1_qubic1 represent the proportions from the results obtained using quantile discretization and using max(min [23])-based biclustering on seed 1, seed1_qubic2 represent using quantile discretization and using KL-based biclustering on seed1. In Figure 3A, it can be found that most CEMs have P-value more than 0.001 and seed1_qubic1 seems to have more significant P-value. Constrain P-value below 0.00001 and there is barely CEMs remained (less than 7%). In Figure 3B the majority of (more than70%) CEMs are with enriched lncRNA more than 3 and especially most (around 85%) of CEMs from seed1_qubic1.
For validation, we separated the HG + MG genes into two parts randomly and equally for 10 times and obtained 10 cover ratios correspondingly to check the accuracy of the previously predicted genes. The results of our validation are calculated and shown in Table 1. From Table 1 it can be found that all of the cover ratios are under 25% and the average ratio is 16.25%. We further calculated the p-value of the coverage rates. The results indicated that even the coverage date has a lot of space to be improved, the statistical significance of them are acceptable.
Group | 1 | 2 | 3 | 4 | 5 | 6 | 7 | 8 | 9 | 10 |
Ratio | 14.00% | 19.60% | 14.00% | 15.70% | 14.50% | 21.30% | 9.80% | 24.30% | 14.00% | 15.30% |
P-value | 7.58E-07 | 5.14E-14 | 7.58E-07 | 8.11E-09 | 2.56E-07 | 1.24E-16 | 5.37E-03 | 1.27E-21 | 7.58E-07 | 2.65E-08 |
Since lncRNA plays an important role in regulation, they should have cooperation with transcription factor [23,24]. Thus we conduct the analysis about the DNA binding sites of related to CEMs [19,22,25]. As described in the Method section, we choose five CEMs to conducted TF motif analysis. The corresponding gene list files each containing 3, 6, 14, 20 and 21 genes. The predicted motifs and the comparison between them and JASPAR motifs are listed in Table 2, along with the function of the target TFs. In Table 2, the second column has the name of lncRNA related with this CEMs and the p-value of their correlations; The third column contains the motif consensus by Homer; The fourth column provides TF names of the most similar motifs in JASPAR, along with the similarity scores in the fifth column. These TFs may have cooperation with corresponding lncRNAs. In the first column, all the P-value of the LncRNA from the CEM is below 0.01 and the least P-value is from LncRNA HOTAIR. The supplementary table S1 with more details, including and the logo of discovered motifs and the functions of corresponding TFs, can be downloaded by visiting the GitHub link.
lncRNA (p-value) | Homer motifs | JASPAR TFs | Scores | |
1 | FOXCUT 3.6e-3 |
AACCAVTTHDCG | TFCP2 | 0.64 |
TCCTATCACACR | MEIS2 | 0.62 | ||
TTTTHAAAGGGG | CHR | 0.67 | ||
ARTGGTTGTWGA | FOXJ2 | 0.58 | ||
GCAATCTCGC | IRF4 | 0.66 | ||
2 | ANCR 1.1e-3 |
AGGGTGACAG | SPZ1 | 0.80 |
GGTATCTTAC | GATA5 | 0.64 | ||
CTCATAGGAG | GCM1 | 0.65 | ||
TAAGTGAAAG | PRDM1 | 0.86 | ||
CTTTTGGAAC | CHR | 0.65 | ||
3 | 250-280 2.2e-4 |
WYTRTCTTTGCG | RXR | 0.61 |
TCTTACGG | ELK1 | 0.71 | ||
GGCAAGGA | SD | 0.76 | ||
GAGGTATGTT | TEAD1 | 0.70 | ||
TGCCGGGAGCGT | POL | 0.64 | ||
4 | HOXD-AS1 6.1e-3 |
CTCGAGTAGG | PB0114 | 0.63 |
GCCCCCTGCA | PB0076 | 0.74 | ||
ACGYMYATKYCC | GFY | 0.59 | ||
AGCGGGTT | PH | 0.68 | ||
AGGCGCCGCGCC | SP1 | 0.69 | ||
5 | HOTAIR 5e-6 |
TGGCGCAGCGCG | PB | 0.67 |
GTACAACTTT | PB | 0.66 | ||
CMTSTGTCWCYK | NeuroG2 | 0.66 | ||
GTGATCCATT | RHOXF1 | 0.68 | ||
GGTMGRRGTGMW | TBX20 | 0.58 |
In order to further evaluate the biological significance of the identified CEMs, we tested the enrichment of the genes in each CEM in Gene ontology terms and KEGG pathways using clusterProfiler package of R project BioConductor under q-value cutoff 0.05, of which the description of the GO terms and KEGG pathways that the CEMs are enriched in are presented in Table 3 and Table 4 respectively. And the supplementary table S2 with more details, including original and adjusted P-value, proportion of the matched genes, gene's ID, etc., can be downloaded on GitHub link.
LncRNA | ID | Description | q-value |
FOXCUT | GO:0033613 | transmembrane receptor protein tyrosine kinase activity | 1.2937E-02 |
GO:0033613 | activating transcription factor binding | 1.2937E-02 | |
GO:0019199 | transmembrane receptor protein kinase activity | 1.2937E-02 | |
GO:0001085 | RNA polymerase Ⅱ transcription factor binding | 2.0767E-02 | |
250-280 | GO:0003735 | structural constituent of ribosome | 3.1600E-06 |
GO:0003729 | mRNA binding | 1.1140E-03 | |
GO:0008483 | transaminase activity | 1.1140E-03 | |
GO:0048027 | mRNA 5'-UTR binding | 1.1140E-03 | |
GO:0016769 | transferase activity, transferring nitrogenous groups | 1.1140E-03 | |
GO:0045182 | translation regulator activity | 1.5826E-03 | |
GO:0030170 | pyridoxal phosphate binding | 1.5826E-03 | |
GO:0070279 | vitamin B6 binding | 1.5826E-03 | |
GO:0019843 | rRNA binding | 1.5826E-03 | |
GO:0019842 | vitamin binding | 3.1903E-03 | |
HOXD-AS1 | GO:0004714 | transmembrane receptor protein tyrosine kinase activity | 1.2937E-02 |
GO:0033613 | activating transcription factor binding | 1.2937E-02 | |
GO:0019199 | transmembrane receptor protein kinase activity | 1.2937E-02 | |
GO:0001085 | RNA polymerase Ⅱ transcription factor binding | 2.0767E-02 | |
HOTAIR | GO:0005109 | frizzled binding | 1.9097E-03 |
GO:0001227 | transcriptional repressor activity, RNA polymerase Ⅱ transcription regulatory region sequence-specific binding | 1.9097E-03 | |
GO:0001664 | G-protein coupled receptor binding | 1.9686E-03 | |
GO:0001078 | transcriptional repressor activity, RNA polymerase Ⅱ core promoter proximal region sequence-specific binding | 1.1196E-02 | |
GO:0008201 | heparin binding | 1.2885E-02 | |
GO:0005539 | glycosaminoglycan binding | 1.8790E-02 | |
GO:1901681 | sulfur compound binding | 1.9016E-02 | |
GO:0045236 | CXCR chemokine receptor binding | 2.1785E-02 | |
GO:0008301 | DNA binding, bending | 2.1785E-02 | |
GO:0001223 | transcription coactivator binding | 2.1785E-02 | |
GO:0042813 | Wnt-activated receptor activity | 2.1785E-02 | |
GO:0035198 | miRNA binding | 2.2807E-02 | |
GO:0017147 | Wnt-protein binding | 2.5258E-02 | |
GO:1990841 | promoter-specific chromatin binding | 2.5258E-02 | |
GO:0000982 | transcription factor activity, RNA polymerase Ⅱ core promoter proximal region sequence-specific binding | 2.5258E-02 | |
GO:0001221 | transcription cofactor binding | 2.5587E-02 |
LncRNA | ID | Description | q-value |
FOXCUT | hsa05216 | Thyroid cancer | 8.8587E-03 |
hsa04510 | Focal adhesion | 8.8587E-03 | |
hsa05205 | Proteoglycans in cancer | 8.8587E-03 | |
hsa05218 | Melanoma | 1.7683E-02 | |
hsa05214 | Glioma | 1.7683E-02 | |
hsa04151 | PI3K-Akt signaling pathway | 1.8745E-02 | |
hsa05215 | Prostate cancer | 1.8745E-02 | |
hsa01522 | Endocrine resistance | 1.8745E-02 | |
hsa04919 | Thyroid hormone signaling pathway | 2.2317E-02 | |
hsa04152 | AMPK signaling pathway | 2.2317E-02 | |
hsa04068 | FoxO signaling pathway | 2.4442E-02 | |
hsa04550 | Signaling pathways regulating pluripotency of stem cells | 2.5129E-02 | |
hsa05224 | Breast cancer | 2.5129E-02 | |
hsa04218 | Cellular senescence | 2.7471E-02 | |
hsa05225 | Hepatocellular carcinoma | 2.7471E-02 | |
hsa04530 | Tight junction | 2.7471E-02 | |
250-280 | hsa03010 | Ribosome | 2.9900E-05 |
hsa01210 | 2-Oxocarboxylic acid metabolism | 3.7322E-03 | |
hsa00220 | Arginine biosynthesis | 3.7322E-03 | |
hsa00250 | Alanine, aspartate and glutamate metabolism | 4.7849E-03 | |
hsa01230 | Biosynthesis of amino acids | 7.9156E-03 | |
HOXD-AS1 | hsa05216 | Thyroid cancer | 8.8587E-03 |
hsa04510 | Focal adhesion | 8.8587E-03 | |
hsa05205 | Proteoglycans in cancer | 8.8587E-03 | |
hsa05218 | Melanoma | 1.7683E-02 | |
hsa05214 | Glioma | 1.7683E-02 | |
hsa04151 | PI3K-Akt signaling pathway | 1.8745E-02 | |
hsa05215 | Prostate cancer | 1.8745E-02 | |
hsa01522 | Endocrine resistance | 1.8745E-02 | |
hsa04919 | Thyroid hormone signaling pathway | 2.2317E-02 | |
hsa04152 | AMPK signaling pathway | 2.2317E-02 | |
hsa04068 | FoxO signaling pathway | 2.4442E-02 | |
hsa04550 | Signaling pathways regulating pluripotency of stem cells | 2.5129E-02 | |
hsa05224 | Breast cancer | 2.5506E-02 | |
hsa04218 | Cellular senescence | 2.7471E-02 | |
hsa05225 | Hepatocellular carcinoma | 2.7471E-02 | |
hsa04530 | Tight junction | 2.7471E-02 | |
HOTAIR | hsa04310 | Wnt signaling pathway | 5.7295E-03 |
Within this study, we have developed a method for elucidating lncRNA-gene and transcription factor-lncRNA interactions using a biclustering approach. The method was performed on 2 breast cancer RNA-seq datasets from TCGA. The bicluster method allows for the identification of particular expression patterns across multiple datasets, indicating networks of lncRNA and gene interactions. The developed method will also provide a way for future lncRNA interaction studies. Certainly, the predict performance still far from satisfactory, which is not unexpected since we only used RNA-Seq data. Actually, the interaction mechanism between lncRNA and genes are far more complex, and more data should be involved if we want to capture the whole picture of them. We are planning to include some other data, like proteomics and chromatin accessibility information, to improve the prediction. Besides, the evaluation on the relationship between lncRNA and predicted CEMs also has the potential to be improved, e.g. calculating the adjusted P-value or overall P-value in place of the original P-values used in this study. In view of the application, we will work on more specific examples of the regulatory functions of some particular lncRNAs and identify some hypothesized mechanisms of these regulatory functions. Also, the further analysis of the difference of lncRNA related genes between tumor and normal samples could provide more information for studying the process and mechanism of cancer occurrence and development, e.g. determination of the stage of developed tumors, which will be our concern in the future research.
This work was supported by the National Nature Science Foundation of China (NSFC) [61772313 and 61432010], Young Scholars Program of Shandong University [YSPSDU, 2015WLJH19], the Innovation Method Fund of China (2018IM020200), and Shanghai Municipal Science and Technology Major Project (2018SHZDZX01) and ZHANGJIANGLAB. Qin Ma's work was supported by an R01 Award from the National Institute of General Medical Sciences of the National Institutes of Health [GM131399-01]. This work used the Extreme Science and Engineering Discovery Environment (XSEDE), which is supported by the National Science Foundation [ACI-1548562]. The content is solely the responsibility of the authors and does not necessarily represent the official views of the National Institutes of Health and the National Science Foundation.
All authors declare no conflicts of interest in this paper.
[1] | Available online: https://patch.com/new-jersey/oceancity/nj-coronavirus-update-gov-murphy-considers-curfew-31-new-cases (accessed on16 March 2020). |
[2] | Available online: https://www.osha.gov/SLTC/covid-19/medicalinformation.html (accessed on.17 March 2020). |
[3] | Centers for Disease Control and Prevention, https://www.cdc.gov/coronavirus/2019-ncov/prevent-getting-sick/social-distancing.html (accessed on 8 April 2020) |
[4] |
H. Pham, On estimating the number of deaths related to Covid-19, Mathematics, 8 (2020), 655. https://doi.org/10.3390/math8050655 doi: 10.3390/math8050655
![]() |
[5] | Worldometers, 2022 (accessed on April 1, 2022). |
[6] | https://www.worldometers.info/coronavirus/?utm_campaign = homeAdvegas1?#countries |
[7] | World Health Organization, https://covid19.who.int/ (accessed on April 1, 2022). |
[8] |
H. Pham, Estimating the COVID-19 death toll by considering the time-dependent effects of various pandemic restrictions, Mathematics, 8 (2020), 1628. https://doi.org/10.3390/math8091628 doi: 10.3390/math8091628
![]() |
[9] |
A. W. H. Chin, J. T. S. Chu, M. R. A. Perera, K. P. Y. Hui, H. L. Yen, M. C. W. Chan, et al., Stability of SARS-CoV-2 in different environmental conditions, Lancet Microbe, 1 (2020), 1. https://doi.org/10.1016/S2666-5247(20)30003-3 doi: 10.1016/S2666-5247(20)30003-3
![]() |
[10] |
E. Dong, H. Du, L. Gardner, An interactive web-based dashboard to track COVID-19 in real time, Lancet Infect. Dis. 20 (2020), 533-534. https://doi.org/10.1016/S1473-3099(20)30120-1 doi: 10.1016/S1473-3099(20)30120-1
![]() |
[11] |
K. Prem, Y. Liu, T. W. Russell, A. J. Kucharski, R. M. Eggo, N. Davies, et al., The effect of control strategies to reduce social mixing on outcomes of the COVID-19 epidemic in Wuhan, China: A modelling study, Lancet Public Health, 20 (2020), e261-e270. https://doi.org/10.1016/S2468-2667(20)30073-6 doi: 10.1016/S2468-2667(20)30073-6
![]() |
[12] |
G. Sebastiani, M. Massa, E. Riboli, Covid-19 epidemic in Italy: Evolution, projections and impact of government measures, Eur. J. Epidemiol., 35 (2020), 341-345. https://doi.org/10.1007/s10654-020-00631-6 doi: 10.1007/s10654-020-00631-6
![]() |
[13] |
G. Onder, G. Rezza, S. Brusaferro, Case-Fatality rate and characteristics of patients dying in relation to COVID-19 in Italy, JAMA, 20 (2020), 1775-1776. https://doi.org/10.1001/jama.2020.4683 doi: 10.1001/jama.2020.4683
![]() |
[14] |
D. D. Rajgor, M. H. Lee, S. Archuleta, N. Bagdasarian, S. C. Quek, The many estimates of the COVID-19 case fatality rate, Lancet Infect. Dis., 20 (2020), 776-777. https://doi.org/10.1016/S1473-3099(20)30244-9 doi: 10.1016/S1473-3099(20)30244-9
![]() |
[15] |
A. J. Kucharski, T. W. Russell, C. Diamond, Y. Liu, J. Edmunds, S. Funk, et al., Early dynamics of transmission and control of COVID-19: A mathematical modelling study, Lancet Infect. Dis., 20 (2020), 553-558. https://doi.org/10.1016/S1473-3099(20)30144-4 doi: 10.1016/S1473-3099(20)30144-4
![]() |
[16] |
X. Wang, Y. Zhang, F. Fang, Role of vitamin D in COVID-19 infections and deaths, J. Evid. Based Med., 14 (2021), 5-6. https://doi.org/10.1111/jebm.12421 doi: 10.1111/jebm.12421
![]() |
[17] |
E. D. Gorham, C. F. Garland, F. C. Garland, W. B. Grant, S. B. Mohr, M. Lipkin, et al., Optimal vitamin D status for colorectal cancer prevention: A quantitative meta analysis, Am. J. Prev. Med., 32 (2007), 210-216. https://doi.org/10.1016/j.amepre.2006.11.004 doi: 10.1016/j.amepre.2006.11.004
![]() |
[18] |
A. G. Pittas, S. S. Harris, P. C. Stark, H. B. Dawson, The effects of calcium and vitamin D supplementation on blood glucose and markers of inflammation in nondiabetic adults, Diabetes Care, 30 (2007), 980-986. https://doi.org/10.2337/dc06-1994 doi: 10.2337/dc06-1994
![]() |
[19] |
M. Urashima, T. Segawa, M. Okazaki, M. Kurihara, Y. Wada, H. Ida, Randomized trial of vitamin D supplementation to prevent seasonal influenza A in schoolchildren, Am. J. Clin. Nutr., 91 (2010), 1255-1260. https://doi.org/10.3945/ajcn.2009.29094 doi: 10.3945/ajcn.2009.29094
![]() |
[20] |
H. Pham, D. H. Pham, A novel generalized logistic dependent model to predict the presence of breast cancer based on biomarkers, Concurr. Comput. Pract. Exper., 32 (2020), 1. https://doi.org/10.1002/cpe.5467 doi: 10.1002/cpe.5467
![]() |
[21] |
V. Verhoeven, K. Vanpuyenbroeck, M. Lopez-Hartmann, J. Wens, R. Remmen, Walk on the sunny side of life—epidemiology of hypovitaminosis D and mental health in elderly nursing home residents, J. Nutr. Health Aging, 16 (2012), 417-420. https://doi.org/10.1007/s12603-011-0361-5 doi: 10.1007/s12603-011-0361-5
![]() |
[22] |
D. Boersma, O. Demontiero, A. Z. Mohtasham, S. Hassan, H. Suarez, D. Geisinger, et al., Vitamin D status in relation to postural stability in the elderly, J. Nutr. Health Aging, 16 (2012), 270-275. https://doi.org/10.1007/s12603-011-0345-5 doi: 10.1007/s12603-011-0345-5
![]() |
[23] |
N. Charoenngam, M. F. Holick, Immunologic effects of vitamin D on human health and disease, Nutrients, 12 (2020), 2097. https://doi.org/10.3390/nu12072097 doi: 10.3390/nu12072097
![]() |
[24] |
A. R. Martineau, D. A. Jolliffe, L. Greenberg, J. F. Aloia, P. Bergman, G. Dubnov-Raz, Vitamin D supplementation to prevent acute respiratory infections: Individual participant data meta-analysis, Health Technol. Assess., 23 (2019), 23020. https://doi.org/10.3310/hta23020 doi: 10.3310/hta23020
![]() |
[25] | C. E. Hayes, J. M. Ntambi, Multiple sclerosis: Lipids, Lymphocytes, and vitamin D, Immunometabolism, 10 (2020), 19. |
[26] |
K. de Haan, A. B. J. Groeneveld, H. R. H. de Geus, M. Egal, A. Struijs, Vitamin D deficiency as a risk factor for infection, sepsisand mortality in the critically ill: Systematic review and meta-analysis, Crit. Care, 66 (2014), 6604. https://doi.org/10.1186/s13054-014-0660-4 doi: 10.1186/s13054-014-0660-4
![]() |
[27] |
A. Braun, D. Chang, K. Mahadevappa, F. K. Gibbons, Y. Liu, E. Giovannucci, Association of low serum 25-hydroxyvitamin D levels and mortality in the critically ill, Crit. Care Med., 39 (2011), 671. https://doi.org/10.1097/CCM.0b013e318206ccdf doi: 10.1097/CCM.0b013e318206ccdf
![]() |
[28] |
P. Autier, M. Boniol, C. Pizot, P. Mullie, Vitamin D status and ill health: A systematic review, Lancet Diabetes Endocrinol., 2 (2014) 76. https://doi.org/10.1016/S2213-8587(13)70165-7 doi: 10.1016/S2213-8587(13)70165-7
![]() |
[29] |
Y. F. Zhou, B. A. Luo, L. L. Qin, The association between vitamin D deficiency and community-acquired pneumonia, Medicine, 98 (2019), e17252. https://doi.org/10.1097/MD.0000000000017252 doi: 10.1097/MD.0000000000017252
![]() |
[30] |
E. C. Goodall, A. C. Granados, K. Luinstra, E. Pullenayegum, B. L. Coleman, M. Loeb, Vitamin D3 and gargling for the prevention of upper respiratory tract infections: A randomized controlled trial, BMC Infect. Dis., 11 (2014), 14273. https://doi.org/10.1186/1471-2334-14-273 doi: 10.1186/1471-2334-14-273
![]() |
[31] |
A. S. Vanherwegen, C. Gysemans, C. Mathieu, Regulation of immune function by vitamin D and its use in diseases of immunity, Endocrinol. Metab. Clin. N. Am., 46 (2017), 1061. https://doi.org/10.1016/j.ecl.2017.07.010 doi: 10.1016/j.ecl.2017.07.010
![]() |
[32] |
C. Greiller, A. Martineau, Modulation of the immune response to respiratory viruses by vitamin D, Nutrients, 7 (2015), 4240-4270. https://doi.org/10.3390/nu7064240 doi: 10.3390/nu7064240
![]() |
[33] |
M. T. Zdrenghea, H. Makrinioti, C. Bagacean, A. Bush, S. L. Johnston, L.A. Stanciu, Vitamin D modulation of innate immune responses to respiratory viral infections, Rev. Med. Virol., 27 (2016), e1909. https://doi.org/10.1002/rmv.1909 doi: 10.1002/rmv.1909
![]() |
[34] |
J. R. Sabetta, P. DePetrillo, R. J. Cipriani, J. Smardin, L. A. Burns, M. L. Landry, Serum 25-hydroxyvitamin d and the incidence of acute viral respiratory tract infections in healthy adults, PLoS One, 5 (2010), e11088. https://doi.org/10.1371/journal.pone.0011088 doi: 10.1371/journal.pone.0011088
![]() |
[35] | T. R. Ingham, B. Jones, C. A. Camargo, J. Kirman, A. C. Dowell, J. Crane, Association of vitamin D deficiency with severity of acute respiratory infection: A case-control study in New Zealand children, Eur. Respir. J. Eur. Respir. Soc., 44 (2014), 124. |
[36] |
C. FGunville, P. MMourani, A. AGinde, The role of vitamin D in prevention and treatment of infection, Inflamm. Allergy-DrugTargets, 12 (2013), 239-245. https://doi.org/10.2174/18715281113129990046 doi: 10.2174/18715281113129990046
![]() |
[37] |
A. L. Khoo, L. Chai, H. Koenen, I. Joosten, M. Netea, A. van der Ven, Translating the role of vitamin D3 in infectious diseases, Crit. Rev. Microbiol., 38 (2012), 122. https://doi.org/10.3109/1040841X.2011.622716 doi: 10.3109/1040841X.2011.622716
![]() |
[38] |
R. Taha, S. Abureesh, S. Alghamdi, R. Y. Hassan, M. M. Cheikh, R. A. Bagabir, The relationship between vitamin D and infections including COVID-19: Any hopes? Int. J. Gen. Med., 14 (2021), 3849. https://doi.org/10.2147/IJGM.S317421 doi: 10.2147/IJGM.S317421
![]() |
[39] |
A. R. Martineau, D. A. Jolliffe, R. L. Hooper, L. Greenberg, J. F. Aloia, P. Bergman, Vitamin D supplementation to prevent acute respiratory tract infections: Systematic review and meta-analysis of individual participant data, BMJ, 65 (2017), 6583. https://doi.org/10.1136/bmj.i6583 doi: 10.1136/bmj.i6583
![]() |
[40] |
R. C. A. Dancer, D. Parekh, S. Lax, V. D'Souza, S. Zheng, C. R. Bassford, Vitamin D deficiency contributes directly to the acute respiratory distress syndrome (ARDS), Thorax BMJ, 70 (2015), 617. https://doi.org/10.1136/thoraxjnl-2014-206680 doi: 10.1136/thoraxjnl-2014-206680
![]() |
[41] |
D. R. Thickett, T. Moromizato, A. A. Litonjua, K. Amrein, S. A. Quraishi, K. A. Lee-Sarwar, Association between prehospital vitamin D status and incident acute respiratory failure in critically ill patients: A retrospective cohort study, BMJ Open Respir. Res., 2 (2015), e74. https://doi.org/10.1136/bmjresp-2014-000074 doi: 10.1136/bmjresp-2014-000074
![]() |
[42] | M. T. Cantorna, B. D. Mahon, D-hormone and the immune system, J. Rheumatol. Suppl., 76 (2005), 11-20. |
[43] |
A. Antico, M. Tampoia, R. Tozzoli, N. Bizzaro, Can supplementation with vitamin D reduce the risk or modify the course of autoimmune diseases? A systematic review of the literature, Autoimmun. Rev., 12 (2012), 127. https://doi.org/10.1016/j.autrev.2012.07.007 doi: 10.1016/j.autrev.2012.07.007
![]() |
[44] | T. Michigami, O. Rickets, Consensus on vitamin D deficiency and insufficiency in children, Clin. Calcium., 28 (2018), 1307-1311. |
[45] |
W. Grant, H. Lahore, S. McDonnell, C. Baggerly, C. French, J. Aliano, Evidence that vitamin D supplementation could reduce risk of influenza and COVID-19 infections and deaths, Nutrients, 12 (2020), 988. https://doi.org/10.3390/nu12040988 doi: 10.3390/nu12040988
![]() |
[46] |
P. A. Danai, S. Sinha, M. Moss, M. J. Haber, G. S. Martin, Seasonal variation in the epidemiology of sepsis, Crit. Care Med., 35 (2007), 410-415. https://doi.org/10.1097/01.CCM.0000253405.17038.43 doi: 10.1097/01.CCM.0000253405.17038.43
![]() |
[47] |
W. B. Grant, Variations in vitamin D production could possibly explain the seasonality of childhood respiratory infections in Hawaii, Pediatr. Infect. Dis. J., 27 (2008), 853. https://doi.org/10.1097/INF.0b013e3181817bc1 doi: 10.1097/INF.0b013e3181817bc1
![]() |
[48] |
J. J. Cannell, R. Vieth, J. Umhau, M. Holick, W. Grant, S. Madronich, et al., Epidemic influenza and vitamin D, Epidemiol. Infect., 134 (2006), 1129-1140. https://doi.org/10.1017/S0950268806007175 doi: 10.1017/S0950268806007175
![]() |
[49] |
V. Dimitrov, C. Barbier, A. Ismailova, Y. Wang, K. Dmowski, R. Salehi-Tabar, et al., Vitamin D-regulated gene expression profiles: species-specificity and cell-specific effects on metabolism and immunity, Endocrinology, 162 (2021), 218. https://doi.org/10.1210/endocr/bqaa218 doi: 10.1210/endocr/bqaa218
![]() |
[50] |
M. Olliver, L. Spelmink, J. Hiew, U. Meyer-Hoffert, B. Henriques-Normark, P. Bergman, Immunomodulatory effects of vitamin D on innate and adaptive immune responses to Streptococcus pneumoniae, J. Infect. Dis., 208 (2013), 1474-1481. https://doi.org/10.1093/infdis/jit355 doi: 10.1093/infdis/jit355
![]() |
[51] |
S. Maggini, E. S. Wintergerst, S. Beveridge, D. H. Hornig, Selected vitamins and trace elements support immune function by strengthening epithelial barriers and cellular and humoral immune responses, Br. J. Nut., 98 (2007), S29-S35. https://doi.org/10.1017/S0007114507832971 doi: 10.1017/S0007114507832971
![]() |
[52] |
W. B. Grant, H. Lahore, S. L. McDonnell, C. A. Baggerly, C. B. French, J. L. Aliano, et al., Evidence that vitamin D supplementation could reduce risk of influenza and COVID-19 infections and deaths, Nutrients, 12 (2020), 988. https://doi.org/10.3390/nu12040988 doi: 10.3390/nu12040988
![]() |
[53] |
S. Piumika, T. J. Dhanushya, K. Neil, J. Ranil, Impact of vitamin D deficiency on COVID-19, Clin. Nutr. ESPEN, 44 (2021), 372-378. https://doi.org/10.1016/j.clnesp.2021.05.011 doi: 10.1016/j.clnesp.2021.05.011
![]() |
[54] | E. Laird, R. A. Kenny, Vitamin D deficiency in Ireland-implications for COVID-19. Results from the Irish Longitudinal Study on Ageing (TILDA), April 2020. https://doi.org/10.38018/TildaRe.2020-05 |
[55] |
D. P. Richardson, J. A. Lovegrove, Nutritional status of micronutrients as a possible and modifiable risk factor for COVID-19: A UK perspective, Br. J. Nutr., 125 (2021), 678-684. https://doi.org/10.1017/S000711452000330X doi: 10.1017/S000711452000330X
![]() |
[56] |
M. Ebadi, A. J. Montano-Loza, Perspective: Improving vitamin D status in the management of COVID-19, Eur. J. Clin. Nutr., 74 (2020), 856-859. https://doi.org/10.1038/s41430-020-0661-0 doi: 10.1038/s41430-020-0661-0
![]() |
[57] |
W. B. Grant, H. Lahore, S. L. McDonnell, C. A. Baggerly, C. B. French, J. L. Aliano, et al., Evidence that vitamin D supplementation could reduce risk of influenza and COVID-19 infections and deaths, Nutrients, 12 (2020), 988. https://doi.org/10.3390/nu12040988 doi: 10.3390/nu12040988
![]() |
[58] | D. M. McCartney, D. G. Byrne, Optimisation of vitamin D status for enhanced Immuno-protection against Covid-19, Ir. Med. J., 113 (2020), 58. |
[59] |
M. Di Rosa, M. Malaguarnera, F. Nicoletti, L. Malaguarnera, Vitamin D3: A helpful immuno-modulator. Immunology, 134 (2020), 123-139. https://doi.org/10.1111/j.1365-2567.2011.03482.x doi: 10.1111/j.1365-2567.2011.03482.x
![]() |
[60] |
J. Zhong, J. Tang, C. Ye, L. Dong, The immunology of COVID-19: Is immune modulation an option for treatment? Lancet Rheumatol., 2 (2020), e428-e436. https://doi.org/10.1016/S2665-9913(20)30120-X doi: 10.1016/S2665-9913(20)30120-X
![]() |
[61] |
A. Panarese, E. Shahini, Covid-19, and vitamin D, Aliment. Pharmacol. Ther., 51 (2020), 993. https://doi.org/10.1111/apt.15752 doi: 10.1111/apt.15752
![]() |
[62] |
G. Grasselli, T. Tonetti, A. Protti, T. Langer, M. Girardis, G. Bellani, et al., Pathophysiology of COVID-19-associated acute respiratory distress syndrome: A multicentre prospective observational study, Lancet Respir. Med., 8 (2020), 1201-1208. https://doi.org/10.1016/S2213-2600(20)30370-2 doi: 10.1016/S2213-2600(20)30370-2
![]() |
[63] |
A. A. Ginde, J. M. Mansbach, C. A. Camargo, Association between serum 25-hydroxyvitamin D level and upper respiratory tract infection in the Third National Health and Nutrition Examination Survey, Arch. Int. Med., 169 (2009), 384-390. https://doi.org/10.1001/archinternmed.2008.560 doi: 10.1001/archinternmed.2008.560
![]() |
[64] |
D. A. Jolliffe, C. J. Griffiths, A. R. Martineau, Vitamin D in the prevention of acute respiratory infection: Systematic review of clinical studies, J. Steroid Biochem. Mol. Biol., 136 (2020), 321-329. https://doi.org/10.1016/j.jsbmb.2012.11.017 doi: 10.1016/j.jsbmb.2012.11.017
![]() |
[65] |
D. Prantas, S. Nandeeta, O. A. Bright, E. H. John, P. Prince, M. Aliu, et al., Effect of vitamin D deficiency on COVID-19 status: A systematic review, COVID, 1 (2021), 97-104. https://doi.org/10.3390/covid1010008 doi: 10.3390/covid1010008
![]() |
[66] |
A. Radujkovic, T. Hippchen, S. Tiwari-Heckler, S. Dreher, M. Boxberger, U. Merle, Vitamin D deficiency and outcome of COVID-19 patients, Nutrients, 12 (2020), 2757. https://doi.org/10.3390/nu12092757 doi: 10.3390/nu12092757
![]() |
[67] |
D. O. Meltzer, T. J. Best, H. Zhang, T. Vokes, V. Arora, J. Solway, Association of vitamin D status and other clinical characteristics with COVID-19 test results, JAMA Netw. Open, 3 (2020), e2019722. https://doi.org/10.1001/jamanetworkopen.2020.19722 doi: 10.1001/jamanetworkopen.2020.19722
![]() |
[68] |
C. E. Hastie, D. F. Mackay, F. Ho, C. A. Celis-Morales, S. V. Katikireddi, C. L. Niedzwiedz, et al., Vitamin D concentrations and COVID-19 infection in UK Biobank, Diabetes Metab. Syndr. Clin. Res. Rev., 14 (2020), 561-565. https://doi.org/10.1016/j.dsx.2020.04.050 doi: 10.1016/j.dsx.2020.04.050
![]() |
[69] |
A. Abrishami, N. Dalili, P. M. Torbati, R. Asgari, M. Arab-Ahmadi, B. Behnam, et al., Possible association of vitamin D status with lung involvement and outcome in patients with COVID-19: A retrospective study, Eur. J. Nutr., 60 (2020), 2249-2257. https://doi.org/10.1007/s00394-020-02411-0 doi: 10.1007/s00394-020-02411-0
![]() |
[70] |
V. Baktash, T. Hosack, N. Patel, S. Shah, P. Kandiah, K. Van den Abbeele, et al., Vitamin D status and outcomes for hospitalised older patients with COVID-19, Postgrad. Med. J., 2 (2020), 1-6. https://doi.org/10.1136/postgradmedj-2020-138712 doi: 10.1136/postgradmedj-2020-138712
![]() |
[71] |
Z. Maghbooli, M. A. Sahraian, M. Ebrahimi, M. Pazoki, M. Kafan, S. Tabriz, et al., Vitamin D sufficiency, a serum 25-hydroxyvitamin D at least 30 ng/mL reduced risk for adverse clinical outcomes in patients with COVID-19 infection, PLoS One, 15 (2020), e0239799. https://doi.org/10.1371/journal.pone.0239799 doi: 10.1371/journal.pone.0239799
![]() |
[72] |
K. Ye, F. Tang, X. Liao, B. A. Shaw, M. Deng, G. Huang, et al., Does serum vitamin D level affect COVID-19 infection and its severity? A case-control study, J. Am. Coll. Nutr., (2020), 1-8. https://doi.org/10.1080/07315724.2020.1826005 doi: 10.1080/07315724.2020.1826005
![]() |
[73] |
P. Bergman, A. C. Norlin, S. Hansen, R. S. Rekha, B. Agerberth, L. Björkhem-Bergman, et al., Vitamin D3 supplementation in patients with frequent respiratory tract infections: A randomised and double-blind intervention study, BMJ Open, 2 (2012), e001663. https://doi.org/10.1136/bmjopen-2012-001663 doi: 10.1136/bmjopen-2012-001663
![]() |
[74] |
A. R. Martineau, N. G. Forouhi, Vitamin D for COVID-19: A case to answer? Lancet Diabetes Endocrinol, 8 (2020), 735-736. https://doi.org/10.1016/S2213-8587(20)30268-0 doi: 10.1016/S2213-8587(20)30268-0
![]() |
[75] |
N. Ali, Role of vitamin D in preventing of COVID-19 infection, progression and severity, J. Infect. Public Health, 13 (2020), 1373-1380. https://doi.org/10.1016/j.jiph.2020.06.021 doi: 10.1016/j.jiph.2020.06.021
![]() |
[76] |
P. C. Ilie, S. Stefanescu, L. Smith, The role of vitamin D in the prevention of coronavirus disease 2019 infection and mortality, Aging Clin. Exp. Res., 32 (2020), 1195-1198. https://doi.org/10.1007/s40520-020-01570-8 doi: 10.1007/s40520-020-01570-8
![]() |
[77] |
S. Walrand, Autumn COVID-19 surge dates in Europe correlated to latitudes, not to temperature-humidity, pointing to vitamin D as contributing factor, Sci. Rep., 11 (2021), 1981. https://doi.org/10.1038/s41598-021-81419-w doi: 10.1038/s41598-021-81419-w
![]() |
[78] |
E. Klingberg, G. Oleröd, J. Konar, M. Petzold, O. Hammarsten, Seasonal variations in serum 25-hydroxy vitamin D levels in a Swedish cohort, Endocrine, 49 (2015), 800-808. https://doi.org/10.1007/s12020-015-0548-3 doi: 10.1007/s12020-015-0548-3
![]() |
[79] | Institute of Medicine. Dietary reference intakes for calcium and vitamin D. Washington, DC: The National Academies Press, 2011. |
[80] |
M. F. Holick N. C. Binkley, H. A. Bischoff-Ferrari, C. M. Gordon, D. A. Hanley, R. P. Heaney, et al., Evaluation, treatment, and prevention of vitamin D deficiency: An Endocrine Society Clinical Practice Guideline, J. Clin. Endocrinol. Metab., 96 (2011), 1911-1130. https://doi.org/10.1210/jc.2011-0385 doi: 10.1210/jc.2011-0385
![]() |
[81] |
D. E. Roth, P. Martz, R. Yeo, C. Prosser, M. Bell, A. B. Jones, Are national vitamin D guidelines sufficient to maintain adequate blood levels in children? Can. J. Public Health, 96 (2005), 443-449. https://doi.org/10.1007/BF03405185 doi: 10.1007/BF03405185
![]() |
[82] | D. Rucker, J. A. Allan, G. H. Fick, D. A. Hanley, Vitamin D insufficiency in a population of healthy western Canadians, CMAJ, 166 (2002), 1517-1524. |
[83] |
R. Vieth, D. E. Cole, G. A. Hawker, H. M. Trang, L. A. Rubin, Winter time vitamin D insufficiency is common in young Canadian women, and their vitamin D intake does not prevent it, Eur. J. Clin. Nutr., 55 (2001), 1091-1097. https://doi.org/10.1038/sj.ejcn. 1601275 doi: 10.1038/sj.ejcn.1601275
![]() |
[84] |
R. P. Heaney, K. M. Davies, T. C. Chen, M. F. Holick, M. J. Barger-Lux, Human serum 25-hydroxycholecalciferol response to extended oral dosing with cholecalciferol, Am. J. Clin. Nutr., 77 (2003), 204-210. https://doi.org/10.1093/ajcn/77.1.204 doi: 10.1093/ajcn/77.1.204
![]() |
[85] |
J. N. Hathcock, A. Shao, R. Vieth, R. Heaney, Risk assessment for vitamin D, Am. J. Clin. Nutr., 85 (2007), 6-18. https://doi.org/10. 1093/ajcn/85.1.6 doi: 10.1093/ajcn/85.1.6
![]() |
[86] |
M. Pfeifer, B. Begerow, H. W. Minne, C. Abrams, D. Nachtigall, C. Hansen, Effects of a short-term vitamin D and calcium supplementation on body sway and secondary hyperparathyroidism in elderly women, J. Bone Miner Res., 15 (2000), 1113-1118. https://doi.org/10.1359/jbmr.2000.15.6.1113 doi: 10.1359/jbmr.2000.15.6.1113
![]() |
[87] | Medicine Io, Dietary reference intakes for calcium, phosphorus, magnesium, vitamin D, and fluoride. The Washington, DC: National Academies Press, 1997. |
[88] |
J. F. Aloia, M. Patel, R. DiMaano, M. Li-Ng, S. A. Talwar, M. Mikhail, et al., Vitamin D intake to attain a desired serum 25- hydroxyvitamin D concentration, Am. J. Clin. Nutr., 87 (2008), 1952-1958. https://doi.org/10.1093/ajcn/87.6.1952 doi: 10.1093/ajcn/87.6.1952
![]() |
[89] |
A. C. Ross, J. E. Manson, S. A. Abrams, J. F. Aloia, P. M. Brannon, S. K. Clinton, et al., The 2011 report on dietary reference intakes for calcium and vitamin D from the institute of medicine: what clinicians need to know, J. Clin. Endocrinol. Metab., 96 (2011), 53-58. https://doi.org/10.1016/j.jada.2011.01.004 doi: 10.1016/j.jada.2011.01.004
![]() |
[90] | EFSA Panel on Dietetic Products N, Allergies. Scientific opinion on the tolerable upper intake level of vitamin D, EFSA J., 10 (2012), 2813. https://doi.org/10.2903/j.efsa.2012.2813 |
[91] |
D. A. Hanley, A. Cranney, G. Jones, S. J. Whiting, W. D. Leslie, D. E. Cole, et al., Vitamin D in adult health and disease: A review and guideline statement from Osteoporosis Canada, CMAJ, 182 (2010), E610-E618. https://doi.org/10.1503/cmaj.080663 doi: 10.1503/cmaj.080663
![]() |
[92] |
S. M. Pietras, B. K. Obayan, M. H. Cai, M. F. Holick, Vitamin D2 treatment for vitamin D deficiency and insufficiency for up to 6 Years, JAMA Intern. Med., 169 (2009), 1806-1818. https://doi.org/10. 1001/archinternmed.2009.361 doi: 10.1001/archinternmed.2009.361
![]() |
[93] |
S. L. McDonnell, C. A. Baggerly, C. B. French, L. L. Baggerly, C. F. Garland, E. D. Gorham, et al., Breast cancer risk markedly lower with serum 25-hydroxyvitamin D concentrations ≥60 vs < 20 ng/ml (150 vs 50 nmol/L): Pooled analysis of two randomized trials and a prospective cohort, PLoS One, 13 (2018), e0199265. https://doi.org/10.1371/journal.pone.0199265 doi: 10.1371/journal.pone.0199265
![]() |
[94] |
J. M. Madden, L. Murphy, L. Zgaga, K. K. Bennett, De novo vitamin D supplement use post-diagnosis is associated with breast cancer survival, Breast Cancer Res. Treat., 172 (2018), 179-90. https://doi.org/10.1007/s10549-018-4896-6 doi: 10.1007/s10549-018-4896-6
![]() |
[95] |
N. Mirhosseini, H. Vatanparast, S. M. Kimball, The association between Serum 25(OH)D status and blood pressure in participants of a community-based program taking vitamin D supplements, Nutrients, 9 (2017), 1244. https://doi.org/10.3390/nu9111244 doi: 10.3390/nu9111244
![]() |
[96] |
A. Rusińska, P. Płudowski, M. Walczak, M. K. Borszewska-Kornacka, A. Bossowski, D. Chlebna-Sokół, et al., Vitamin D supplementation guidelines for general population and groups at risk of vitamin D deficiency in poland—recommendations of the polish society of pediatric endocrinology and diabetes and the expert panel with participation of national specialist consultants and representatives of scientific societies, Front. Endocrinol., 9 (2018). https://doi.org/10.3389/fendo.2018.00246 doi: 10.3389/fendo.2018.00246
![]() |
[97] |
K. Amrein, M. Scherkl, M. Hoffmann, S. Neuwersch-Sommeregger, M. Köstenberger, A. Tmava Berisha, et al., Vitamin D deficiency 2.0: An update on the current status worldwide, European J. Clin. Nutr., 74 (2020), 1498-1513. https://doi.org/10.1038/s41430-020-0558-y doi: 10.1038/s41430-020-0558-y
![]() |
[98] |
N. Charoenngam, M. F. Holick, Immunologic effects of vitamin d on human health and disease, Nutrients, 12 (2020), 1-28. https://doi.org/10.3390/nu12072097 doi: 10.3390/nu12072097
![]() |
[99] |
M. Chakhtoura, M. Rahme, N. Chamoun, G. El-Hajj Fuleihan, Vitamin D in the Middle East and North Africa, Bone Rep., 8 (2018), 135-146. https://doi.org/10.1016/j.bonr.2018.03.004 doi: 10.1016/j.bonr.2018.03.004
![]() |
[100] |
A. Batieha, Y. Khader, H. Jaddou, D. Hyassat, Z. Batieha, M. Khateeb, et al., Vitamin D status in Jordan: dress style and gender discrepancies, Ann. Nutr. Metab., 58 (2011), 10-18. https://doi.org/10.1159/000323097 doi: 10.1159/000323097
![]() |
[101] |
P. Bergman, A. U. Lindh, L. Björkhem-Bergman, J. D. Lindh, Vitamin D and respiratory tract infections: A systematic review and meta-analysis of randomized controlled trials, PLoS One, 8 (2013), e65835. https://doi.org/10.1371/journal.pone.0065835 doi: 10.1371/journal.pone.0065835
![]() |
[102] |
D. A. Jolliffe, C. A. Camargo, J. D. Sluyter, M. Aglipay, J. F. Aloia, D. Ganmaa, et al., Vitamin D supplementation to prevent acute respiratory infections: A systematic review and meta-analysis of aggregate data from randomised controlled trials, Lancet Diab. Endocrinol., 9 (2021), 276-292. https://doi.org/10.1136/thorax-2020-BTSabstracts.105 doi: 10.1136/thorax-2020-BTSabstracts.105
![]() |
[103] |
J. M. Rhodes, S. Subramanian, E. Laird, G. Griffig, R. A. Kenny, Perspective: Vitamin D deficiency and COVID-19 severity—plausibly linked by latitude, ethnicity, impacts on cytokines, ACE2 and thrombosis, J. Intern. Med., 289 (2021), 97-115. https://doi.org/10.1111/joim.13149 doi: 10.1111/joim.13149
![]() |
[104] |
D. O. Meltzer, T. J. Best, H. Zhang, T. Vokes, V. Arora, J. Solway, Association of vitamin D status and other clinical characteristics with COVID-19 test results, JAMA Netw. Open., 3 (2020), e2019722. https://doi.org/10.1001/jamanetworkopen.2020.19722 doi: 10.1001/jamanetworkopen.2020.19722
![]() |
[105] |
E. Merzon, D. Tworowski, A. Gorohovski, S. Vinker, A. G. Cohen, I. Green, et al., Low plasma 25(OH) vitamin D level is associated with increased risk of COVID-19 infection: An Israeli population-based study, FEBS J., 287 (2020), 3693-3702. https://doi.org/10.1111/febs.15495 doi: 10.1111/febs.15495
![]() |
[106] |
A. Jain, R. Chaurasia, N. S. Sengar, M. Singh, S. Mahor, S, Narain, Analysis of vitamin D level among asymptomatic and critically ill COVID-19 patients and its correlation with inflammatory markers, Sci. Rep., 10 (2020), 20191. https://doi.org/10.1038/s41598-020-77093-z doi: 10.1038/s41598-020-77093-z
![]() |
[107] | D. De Smet, K. De Smet, P. Herroelen, S. Gryspeerdt, G. A. Martens, Vitamin D deficiency as risk factor for severe COVID-19: A convergence of two pandemics, medRxiv, (2020). https://doi.org/10.1101/2020.05.01.20079376 |
[108] |
R. L. Schleicher, M. R. Sternberg, A. C. Looker, E. A. Yetley, D. A. Lacher, C. T. Sempos, et al., National estimates of serum total 25-Hydroxyvitamin D and metabolite concentrations measured by liquid chromatography-Tandem mass spectrometry in the US population during 2007-2010, J. Nutr., 146 (2016), 1051-1061.https://doi.org/10.3945/jn.115.227728 doi: 10.3945/jn.115.227728
![]() |
[109] |
K. Sarafin, R. Durazo-Arvizu, L. Tian, K. W. Phinney, S. Tai, J. E. Camara, et al., Standardizing 25-hydroxyvitamin D values from the Canadian health measures survey, Am. J. Clin. Nutr., 102 (2015), 1044-1050. https://doi.org/10.3945/ajcn.114.103689 doi: 10.3945/ajcn.114.103689
![]() |
[110] |
K. D. Cashman, K. G. Dowling, Z. Škrabáková, M. Gonzalez-Gross, J. Valtueña, S. De Henauw, et al., Vitamin D deficiency in Europe: pandemic? Am. J. Clin. Nutr., 103 (2016), 1033-1044. https://doi.org/ 10.3945/ajcn.115.120873 doi: 10.3945/ajcn.115.120873
![]() |
[111] |
K. D. Cashman, Vitamin D deficiency: Defining, prevalence, causes, and strategies of addressing, Calcif. Tissue Int., (2019). https://doi.org/10.1007/s00223-019-00559-4 doi: 10.1007/s00223-019-00559-4
![]() |
[112] |
H. Pham, Modeling U.S. mortality and risk-cost optimization on life expectancy, IEEE Trans. Reliabil., 60 (2011), 125-133. https://doi.org/10.1109/TR.2010.2103990 doi: 10.1109/TR.2010.2103990
![]() |
[113] |
A. A. Dror, N. Morozov, A. Daoud, Y. Namir, O. Yakir, Y. Shachar, et al., Pre-infection 25-hydroxyvitamin D3 levels and association with severity of COVID-19 illness, PLoS One, 17 (2022), e0263069. https://doi.org/10.1371/journal.pone.0263069 doi: 10.1371/journal.pone.0263069
![]() |
[114] |
J. Ng, Y. R. Stovezky, D. J. Brenner, S. C. Formenti, I. Shuryak, Development of a model to estimate the association between delay in cancer treatment and local tumor control and risk of metastates, JAMA Netw. Open, 4 (2021), 1-10. https://doi.org/10.1001/jamanetworkopen.2020.34065 doi: 10.1001/jamanetworkopen.2020.34065
![]() |
[115] |
A. Talkington, R. Durrett, Estimating tumor growth rates in vivo, Bull. Math. Biol., 77 (2015), 1934-1954. https://doi.org/10.1007/s11538-015-0110-8 doi: 10.1007/s11538-015-0110-8
![]() |
[116] |
C. Vaghi, A. Rodallec, R. Fanciullino, J. Ciccolini, J. P. Mochel, M. Mastri, Population modeling of tumor growth curves and the reduced Gompertz model improve prediction of the age of experimental tumors, PLoS Comput. Biol., 16 (2020), e1007178. https://doi.org/10.1371/journal.pcbi.1007178 doi: 10.1371/journal.pcbi.1007178
![]() |
[117] |
D. J. Yin, A. R. Moes, J. G. C. van Hasselt, J. J. Swen, H. J. Guchelaar, A review of mathematical models for tumor dynamics and treatment resistance evolution of solid tumors, CPTPharmacomet. Syst. Pharmacol., 8 (2019), 720-737. https://doi.org/10.1002/psp4.12450 doi: 10.1002/psp4.12450
![]() |
[118] |
T. L. Jackson, H. M. A. Byrne, A mathematical model to study the effects of drug resistance and vasculature on the response of solid tumors to chemotherapy, Math. Biosci., 104 (2000), 17-38. https://doi.org/10.1016/S0025-5564(99)00062-0 doi: 10.1016/S0025-5564(99)00062-0
![]() |
[119] |
C. E. Meacham, S. J. Morrison, Tumour heterogeneity and cancer cell plasticity, Nature, 501 (2013), 328-337. https://doi.org/10.1038/nature12624 doi: 10.1038/nature12624
![]() |
[120] |
X. Sun, J. Bao, Y. Z. Shao, Mathematical modeling of therapy-induced cancer drug resistance: Connecting cancer mechanisms to population survival rates, Sci. Rep., 6 (2016) 22498. https://doi.org/10.1038/srep22498 doi: 10.1038/srep22498
![]() |
[121] |
K. Taniguchi, J. Okami, K. Kodama, M. Higashiyama, K. Kato, Intratumor heterogeneity of epidermal growth factor receptor mutations in lung cancer and its correlation to the response to gefitinib, Cancer Sci., 99 (2008), 929-935. https://doi.org/10.1111/j.1349-7006.2008.00782.x doi: 10.1111/j.1349-7006.2008.00782.x
![]() |
[122] |
E. R. Lestari, H. Arifah, Dynamics of a mathematical model of cancer cells with chemotherapy, J. Phys. Conf. Ser., 1320 (2019), 1-8. https://doi.org/10.1088/1742-6596/1320/1/012026 doi: 10.1088/1742-6596/1320/1/012026
![]() |
[123] |
H. Pham, A dynamic model of multiple time-delay interactions between the virus-infected cells and body's immune system with autoimmune diseases, Axioms, 10 (2021), 216. https://doi.org/10.3390/axioms10030216 doi: 10.3390/axioms10030216
![]() |
[124] |
H. Pham, Mathematical modeling the time-delay interactions between tumor viruses and the immune system with the effects of chemotherapy and autoimmune Diseases, Mathematics, 10 (2022), 756. https://doi.org/10.3390/math10050756 doi: 10.3390/math10050756
![]() |
1. | Sen Yang, Yan Wang, Shuangquan Zhang, Xuemei Hu, Qin Ma, Yuan Tian, NCResNet: Noncoding Ribonucleic Acid Prediction Based on a Deep Resident Network of Ribonucleic Acid Sequences, 2020, 11, 1664-8021, 10.3389/fgene.2020.00090 | |
2. | Lijun Dou, Xiaoling Li, Hui Ding, Lei Xu, Huaikun Xiang, Is There Any Sequence Feature in the RNA Pseudouridine Modification Prediction Problem?, 2020, 19, 21622531, 293, 10.1016/j.omtn.2019.11.014 | |
3. | Guangmin Liang, Jin Wu, Lei Xu, A prognosis-related based method for miRNA selection on liver hepatocellular carcinoma prediction, 2021, 91, 14769271, 107433, 10.1016/j.compbiolchem.2020.107433 | |
4. | Hua-Sheng Chiu, Sonal Somvanshi, Ting-Wen Chen, Pavel Sumazin, 2021, Chapter 22, 978-1-0716-1696-3, 263, 10.1007/978-1-0716-1697-0_22 | |
5. | Juexin Wang, Yan Wang, Towards Machine Learning in Molecular Biology, 2020, 17, 1551-0018, 2822, 10.3934/mbe.2020156 | |
6. | Consolata Gakii, Paul O. Mireji, Richard Rimiru, Graph Based Feature Selection for Reduction of Dimensionality in Next-Generation RNA Sequencing Datasets, 2022, 15, 1999-4893, 21, 10.3390/a15010021 |
Group | 1 | 2 | 3 | 4 | 5 | 6 | 7 | 8 | 9 | 10 |
Ratio | 14.00% | 19.60% | 14.00% | 15.70% | 14.50% | 21.30% | 9.80% | 24.30% | 14.00% | 15.30% |
P-value | 7.58E-07 | 5.14E-14 | 7.58E-07 | 8.11E-09 | 2.56E-07 | 1.24E-16 | 5.37E-03 | 1.27E-21 | 7.58E-07 | 2.65E-08 |
lncRNA (p-value) | Homer motifs | JASPAR TFs | Scores | |
1 | FOXCUT 3.6e-3 |
AACCAVTTHDCG | TFCP2 | 0.64 |
TCCTATCACACR | MEIS2 | 0.62 | ||
TTTTHAAAGGGG | CHR | 0.67 | ||
ARTGGTTGTWGA | FOXJ2 | 0.58 | ||
GCAATCTCGC | IRF4 | 0.66 | ||
2 | ANCR 1.1e-3 |
AGGGTGACAG | SPZ1 | 0.80 |
GGTATCTTAC | GATA5 | 0.64 | ||
CTCATAGGAG | GCM1 | 0.65 | ||
TAAGTGAAAG | PRDM1 | 0.86 | ||
CTTTTGGAAC | CHR | 0.65 | ||
3 | 250-280 2.2e-4 |
WYTRTCTTTGCG | RXR | 0.61 |
TCTTACGG | ELK1 | 0.71 | ||
GGCAAGGA | SD | 0.76 | ||
GAGGTATGTT | TEAD1 | 0.70 | ||
TGCCGGGAGCGT | POL | 0.64 | ||
4 | HOXD-AS1 6.1e-3 |
CTCGAGTAGG | PB0114 | 0.63 |
GCCCCCTGCA | PB0076 | 0.74 | ||
ACGYMYATKYCC | GFY | 0.59 | ||
AGCGGGTT | PH | 0.68 | ||
AGGCGCCGCGCC | SP1 | 0.69 | ||
5 | HOTAIR 5e-6 |
TGGCGCAGCGCG | PB | 0.67 |
GTACAACTTT | PB | 0.66 | ||
CMTSTGTCWCYK | NeuroG2 | 0.66 | ||
GTGATCCATT | RHOXF1 | 0.68 | ||
GGTMGRRGTGMW | TBX20 | 0.58 |
LncRNA | ID | Description | q-value |
FOXCUT | GO:0033613 | transmembrane receptor protein tyrosine kinase activity | 1.2937E-02 |
GO:0033613 | activating transcription factor binding | 1.2937E-02 | |
GO:0019199 | transmembrane receptor protein kinase activity | 1.2937E-02 | |
GO:0001085 | RNA polymerase Ⅱ transcription factor binding | 2.0767E-02 | |
250-280 | GO:0003735 | structural constituent of ribosome | 3.1600E-06 |
GO:0003729 | mRNA binding | 1.1140E-03 | |
GO:0008483 | transaminase activity | 1.1140E-03 | |
GO:0048027 | mRNA 5'-UTR binding | 1.1140E-03 | |
GO:0016769 | transferase activity, transferring nitrogenous groups | 1.1140E-03 | |
GO:0045182 | translation regulator activity | 1.5826E-03 | |
GO:0030170 | pyridoxal phosphate binding | 1.5826E-03 | |
GO:0070279 | vitamin B6 binding | 1.5826E-03 | |
GO:0019843 | rRNA binding | 1.5826E-03 | |
GO:0019842 | vitamin binding | 3.1903E-03 | |
HOXD-AS1 | GO:0004714 | transmembrane receptor protein tyrosine kinase activity | 1.2937E-02 |
GO:0033613 | activating transcription factor binding | 1.2937E-02 | |
GO:0019199 | transmembrane receptor protein kinase activity | 1.2937E-02 | |
GO:0001085 | RNA polymerase Ⅱ transcription factor binding | 2.0767E-02 | |
HOTAIR | GO:0005109 | frizzled binding | 1.9097E-03 |
GO:0001227 | transcriptional repressor activity, RNA polymerase Ⅱ transcription regulatory region sequence-specific binding | 1.9097E-03 | |
GO:0001664 | G-protein coupled receptor binding | 1.9686E-03 | |
GO:0001078 | transcriptional repressor activity, RNA polymerase Ⅱ core promoter proximal region sequence-specific binding | 1.1196E-02 | |
GO:0008201 | heparin binding | 1.2885E-02 | |
GO:0005539 | glycosaminoglycan binding | 1.8790E-02 | |
GO:1901681 | sulfur compound binding | 1.9016E-02 | |
GO:0045236 | CXCR chemokine receptor binding | 2.1785E-02 | |
GO:0008301 | DNA binding, bending | 2.1785E-02 | |
GO:0001223 | transcription coactivator binding | 2.1785E-02 | |
GO:0042813 | Wnt-activated receptor activity | 2.1785E-02 | |
GO:0035198 | miRNA binding | 2.2807E-02 | |
GO:0017147 | Wnt-protein binding | 2.5258E-02 | |
GO:1990841 | promoter-specific chromatin binding | 2.5258E-02 | |
GO:0000982 | transcription factor activity, RNA polymerase Ⅱ core promoter proximal region sequence-specific binding | 2.5258E-02 | |
GO:0001221 | transcription cofactor binding | 2.5587E-02 |
LncRNA | ID | Description | q-value |
FOXCUT | hsa05216 | Thyroid cancer | 8.8587E-03 |
hsa04510 | Focal adhesion | 8.8587E-03 | |
hsa05205 | Proteoglycans in cancer | 8.8587E-03 | |
hsa05218 | Melanoma | 1.7683E-02 | |
hsa05214 | Glioma | 1.7683E-02 | |
hsa04151 | PI3K-Akt signaling pathway | 1.8745E-02 | |
hsa05215 | Prostate cancer | 1.8745E-02 | |
hsa01522 | Endocrine resistance | 1.8745E-02 | |
hsa04919 | Thyroid hormone signaling pathway | 2.2317E-02 | |
hsa04152 | AMPK signaling pathway | 2.2317E-02 | |
hsa04068 | FoxO signaling pathway | 2.4442E-02 | |
hsa04550 | Signaling pathways regulating pluripotency of stem cells | 2.5129E-02 | |
hsa05224 | Breast cancer | 2.5129E-02 | |
hsa04218 | Cellular senescence | 2.7471E-02 | |
hsa05225 | Hepatocellular carcinoma | 2.7471E-02 | |
hsa04530 | Tight junction | 2.7471E-02 | |
250-280 | hsa03010 | Ribosome | 2.9900E-05 |
hsa01210 | 2-Oxocarboxylic acid metabolism | 3.7322E-03 | |
hsa00220 | Arginine biosynthesis | 3.7322E-03 | |
hsa00250 | Alanine, aspartate and glutamate metabolism | 4.7849E-03 | |
hsa01230 | Biosynthesis of amino acids | 7.9156E-03 | |
HOXD-AS1 | hsa05216 | Thyroid cancer | 8.8587E-03 |
hsa04510 | Focal adhesion | 8.8587E-03 | |
hsa05205 | Proteoglycans in cancer | 8.8587E-03 | |
hsa05218 | Melanoma | 1.7683E-02 | |
hsa05214 | Glioma | 1.7683E-02 | |
hsa04151 | PI3K-Akt signaling pathway | 1.8745E-02 | |
hsa05215 | Prostate cancer | 1.8745E-02 | |
hsa01522 | Endocrine resistance | 1.8745E-02 | |
hsa04919 | Thyroid hormone signaling pathway | 2.2317E-02 | |
hsa04152 | AMPK signaling pathway | 2.2317E-02 | |
hsa04068 | FoxO signaling pathway | 2.4442E-02 | |
hsa04550 | Signaling pathways regulating pluripotency of stem cells | 2.5129E-02 | |
hsa05224 | Breast cancer | 2.5506E-02 | |
hsa04218 | Cellular senescence | 2.7471E-02 | |
hsa05225 | Hepatocellular carcinoma | 2.7471E-02 | |
hsa04530 | Tight junction | 2.7471E-02 | |
HOTAIR | hsa04310 | Wnt signaling pathway | 5.7295E-03 |
Group | 1 | 2 | 3 | 4 | 5 | 6 | 7 | 8 | 9 | 10 |
Ratio | 14.00% | 19.60% | 14.00% | 15.70% | 14.50% | 21.30% | 9.80% | 24.30% | 14.00% | 15.30% |
P-value | 7.58E-07 | 5.14E-14 | 7.58E-07 | 8.11E-09 | 2.56E-07 | 1.24E-16 | 5.37E-03 | 1.27E-21 | 7.58E-07 | 2.65E-08 |
lncRNA (p-value) | Homer motifs | JASPAR TFs | Scores | |
1 | FOXCUT 3.6e-3 |
AACCAVTTHDCG | TFCP2 | 0.64 |
TCCTATCACACR | MEIS2 | 0.62 | ||
TTTTHAAAGGGG | CHR | 0.67 | ||
ARTGGTTGTWGA | FOXJ2 | 0.58 | ||
GCAATCTCGC | IRF4 | 0.66 | ||
2 | ANCR 1.1e-3 |
AGGGTGACAG | SPZ1 | 0.80 |
GGTATCTTAC | GATA5 | 0.64 | ||
CTCATAGGAG | GCM1 | 0.65 | ||
TAAGTGAAAG | PRDM1 | 0.86 | ||
CTTTTGGAAC | CHR | 0.65 | ||
3 | 250-280 2.2e-4 |
WYTRTCTTTGCG | RXR | 0.61 |
TCTTACGG | ELK1 | 0.71 | ||
GGCAAGGA | SD | 0.76 | ||
GAGGTATGTT | TEAD1 | 0.70 | ||
TGCCGGGAGCGT | POL | 0.64 | ||
4 | HOXD-AS1 6.1e-3 |
CTCGAGTAGG | PB0114 | 0.63 |
GCCCCCTGCA | PB0076 | 0.74 | ||
ACGYMYATKYCC | GFY | 0.59 | ||
AGCGGGTT | PH | 0.68 | ||
AGGCGCCGCGCC | SP1 | 0.69 | ||
5 | HOTAIR 5e-6 |
TGGCGCAGCGCG | PB | 0.67 |
GTACAACTTT | PB | 0.66 | ||
CMTSTGTCWCYK | NeuroG2 | 0.66 | ||
GTGATCCATT | RHOXF1 | 0.68 | ||
GGTMGRRGTGMW | TBX20 | 0.58 |
LncRNA | ID | Description | q-value |
FOXCUT | GO:0033613 | transmembrane receptor protein tyrosine kinase activity | 1.2937E-02 |
GO:0033613 | activating transcription factor binding | 1.2937E-02 | |
GO:0019199 | transmembrane receptor protein kinase activity | 1.2937E-02 | |
GO:0001085 | RNA polymerase Ⅱ transcription factor binding | 2.0767E-02 | |
250-280 | GO:0003735 | structural constituent of ribosome | 3.1600E-06 |
GO:0003729 | mRNA binding | 1.1140E-03 | |
GO:0008483 | transaminase activity | 1.1140E-03 | |
GO:0048027 | mRNA 5'-UTR binding | 1.1140E-03 | |
GO:0016769 | transferase activity, transferring nitrogenous groups | 1.1140E-03 | |
GO:0045182 | translation regulator activity | 1.5826E-03 | |
GO:0030170 | pyridoxal phosphate binding | 1.5826E-03 | |
GO:0070279 | vitamin B6 binding | 1.5826E-03 | |
GO:0019843 | rRNA binding | 1.5826E-03 | |
GO:0019842 | vitamin binding | 3.1903E-03 | |
HOXD-AS1 | GO:0004714 | transmembrane receptor protein tyrosine kinase activity | 1.2937E-02 |
GO:0033613 | activating transcription factor binding | 1.2937E-02 | |
GO:0019199 | transmembrane receptor protein kinase activity | 1.2937E-02 | |
GO:0001085 | RNA polymerase Ⅱ transcription factor binding | 2.0767E-02 | |
HOTAIR | GO:0005109 | frizzled binding | 1.9097E-03 |
GO:0001227 | transcriptional repressor activity, RNA polymerase Ⅱ transcription regulatory region sequence-specific binding | 1.9097E-03 | |
GO:0001664 | G-protein coupled receptor binding | 1.9686E-03 | |
GO:0001078 | transcriptional repressor activity, RNA polymerase Ⅱ core promoter proximal region sequence-specific binding | 1.1196E-02 | |
GO:0008201 | heparin binding | 1.2885E-02 | |
GO:0005539 | glycosaminoglycan binding | 1.8790E-02 | |
GO:1901681 | sulfur compound binding | 1.9016E-02 | |
GO:0045236 | CXCR chemokine receptor binding | 2.1785E-02 | |
GO:0008301 | DNA binding, bending | 2.1785E-02 | |
GO:0001223 | transcription coactivator binding | 2.1785E-02 | |
GO:0042813 | Wnt-activated receptor activity | 2.1785E-02 | |
GO:0035198 | miRNA binding | 2.2807E-02 | |
GO:0017147 | Wnt-protein binding | 2.5258E-02 | |
GO:1990841 | promoter-specific chromatin binding | 2.5258E-02 | |
GO:0000982 | transcription factor activity, RNA polymerase Ⅱ core promoter proximal region sequence-specific binding | 2.5258E-02 | |
GO:0001221 | transcription cofactor binding | 2.5587E-02 |
LncRNA | ID | Description | q-value |
FOXCUT | hsa05216 | Thyroid cancer | 8.8587E-03 |
hsa04510 | Focal adhesion | 8.8587E-03 | |
hsa05205 | Proteoglycans in cancer | 8.8587E-03 | |
hsa05218 | Melanoma | 1.7683E-02 | |
hsa05214 | Glioma | 1.7683E-02 | |
hsa04151 | PI3K-Akt signaling pathway | 1.8745E-02 | |
hsa05215 | Prostate cancer | 1.8745E-02 | |
hsa01522 | Endocrine resistance | 1.8745E-02 | |
hsa04919 | Thyroid hormone signaling pathway | 2.2317E-02 | |
hsa04152 | AMPK signaling pathway | 2.2317E-02 | |
hsa04068 | FoxO signaling pathway | 2.4442E-02 | |
hsa04550 | Signaling pathways regulating pluripotency of stem cells | 2.5129E-02 | |
hsa05224 | Breast cancer | 2.5129E-02 | |
hsa04218 | Cellular senescence | 2.7471E-02 | |
hsa05225 | Hepatocellular carcinoma | 2.7471E-02 | |
hsa04530 | Tight junction | 2.7471E-02 | |
250-280 | hsa03010 | Ribosome | 2.9900E-05 |
hsa01210 | 2-Oxocarboxylic acid metabolism | 3.7322E-03 | |
hsa00220 | Arginine biosynthesis | 3.7322E-03 | |
hsa00250 | Alanine, aspartate and glutamate metabolism | 4.7849E-03 | |
hsa01230 | Biosynthesis of amino acids | 7.9156E-03 | |
HOXD-AS1 | hsa05216 | Thyroid cancer | 8.8587E-03 |
hsa04510 | Focal adhesion | 8.8587E-03 | |
hsa05205 | Proteoglycans in cancer | 8.8587E-03 | |
hsa05218 | Melanoma | 1.7683E-02 | |
hsa05214 | Glioma | 1.7683E-02 | |
hsa04151 | PI3K-Akt signaling pathway | 1.8745E-02 | |
hsa05215 | Prostate cancer | 1.8745E-02 | |
hsa01522 | Endocrine resistance | 1.8745E-02 | |
hsa04919 | Thyroid hormone signaling pathway | 2.2317E-02 | |
hsa04152 | AMPK signaling pathway | 2.2317E-02 | |
hsa04068 | FoxO signaling pathway | 2.4442E-02 | |
hsa04550 | Signaling pathways regulating pluripotency of stem cells | 2.5129E-02 | |
hsa05224 | Breast cancer | 2.5506E-02 | |
hsa04218 | Cellular senescence | 2.7471E-02 | |
hsa05225 | Hepatocellular carcinoma | 2.7471E-02 | |
hsa04530 | Tight junction | 2.7471E-02 | |
HOTAIR | hsa04310 | Wnt signaling pathway | 5.7295E-03 |