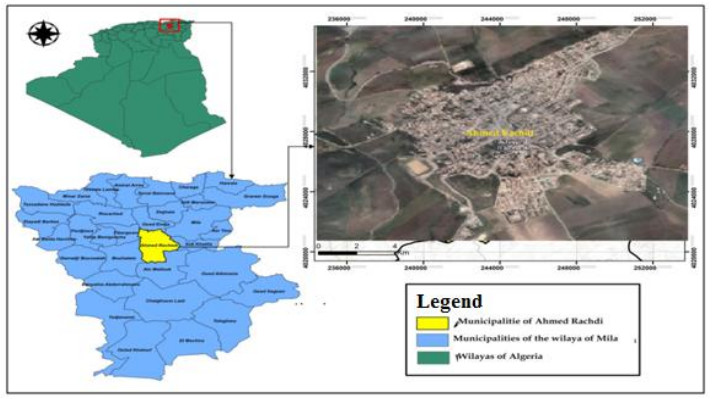
Increasing urbanization related to land pressure and the soil arising from it are aggravating factors of flood risk in urban areas, including storm water runoff. Therefore, urban sanitation networks face an excess of water that exceeds their absorption capacity. This article deals with the effect of impermeability in densely populated urban areas and the function of a combined sewer system under higher rainfall intensities using the Storm Water Management Model (SWMM). The objective is to simulate a possible increase in rainfall intensities for reducing overflow points in the combined sewage system at the study area, which was the city of Ahmed Rachdi, Mila in Algeria. The excessive rainfall intensities were modeled using the SWMM software program to estimate maximum water volumes inside the combined sewage system of the study area. To evaluate the model's performance, a comparison process was used between the values of the flow rates of the pipelines of the sewerage system combined with the design flow rates in the current state and the flow rates of a single modeling of future events available during the study interval. The comparison results showed a good and convergent performance for these models. The results of the flooding volumes using different values of rainfall intensities and different return periods, which were 2, 5, 10 and 25 years, in the modeling of the combined sewage system are 3626, 6888, 8636 and 12676m3, respectively. The suggested scenario included increasing diameters of some pipes in the combined sewage system pipelines. The results using this scenario showed reductions in the total percentage of overflow points from the integrated sewage system of 52.42%, 40.63%, 31.83% and 20.51% using the rainfall intensities for the return periods of 2, 5, 10 and 25 years, respectively. The present study can provide technical support for using software in the planning, controlling and tests of the sewer systems, which contribute to solving the sewer systems' problems.
Citation: RAHMOUN Ibrahim, BENMAMAR Saâdia, RABEHI Mohamed. Comparison between different Intensities of Rainfall to identify overflow points in a combined sewer system using Storm Water Management Model[J]. AIMS Environmental Science, 2022, 9(5): 573-592. doi: 10.3934/environsci.2022034
[1] | Gene T. Señeris . Nabaoy River Watershed potential impact to flooding using Geographic Information System remote sensing. AIMS Environmental Science, 2022, 9(3): 381-402. doi: 10.3934/environsci.2022024 |
[2] | Bedassa D. Kitessa, Semu M. Ayalew, Geremew S. Gebrie, Solomon T. Teferi . Quantifying water-energy nexus for urban water systems: A case study of Addis Ababa city. AIMS Environmental Science, 2020, 7(6): 486-504. doi: 10.3934/environsci.2020031 |
[3] | Zinabu A. Alemu, Emmanuel C. Dioha, Michael O. Dioha . Hydro-meteorological drought in Addis Ababa: A characterization study. AIMS Environmental Science, 2021, 8(2): 148-168. doi: 10.3934/environsci.2021011 |
[4] | Kamonrat Suphawan, Kuntalee Chaisee . Gaussian process regression for predicting water quality index: A case study on Ping River basin, Thailand. AIMS Environmental Science, 2021, 8(3): 268-282. doi: 10.3934/environsci.2021018 |
[5] | Lei Wang, Huan Du, Jiajun Wu, Wei Gao, Linna Suo, Dan Wei, Liang Jin, Jianli Ding, Jianzhi Xie, Zhizhuang An . Characteristics of soil erosion in different land-use patterns under natural rainfall. AIMS Environmental Science, 2022, 9(3): 309-324. doi: 10.3934/environsci.2022021 |
[6] | Nurtiti Sunusi, Giarno . Comparison of some schemes for determining the optimal number of rain gauges in a specific area: A case study in an urban area of South Sulawesi, Indonesia. AIMS Environmental Science, 2022, 9(3): 260-276. doi: 10.3934/environsci.2022018 |
[7] | Joseph A. Kazery, Dayakar P. Nittala, H. Anwar Ahmad . Meteorological effects on trace element solubility in Mississippi coastal wetlands. AIMS Environmental Science, 2019, 6(1): 1-13. doi: 10.3934/environsci.2019.1.1 |
[8] | Abebe Kebede Habtegebreal, Abebaw Bizuneh Alemu, U. Jaya Prakash Raju . Examining the Role of Quasi-biennial Oscillation on Rainfall patterns over Upper Blue Nile Basin of Ethiopia. AIMS Environmental Science, 2021, 8(3): 190-203. doi: 10.3934/environsci.2021013 |
[9] | Leon J. van Ruiten, Thomas Hartmann . The spatial turn and the scenario approach in flood risk management—Implementing the European Floods Directive in the Netherlands. AIMS Environmental Science, 2016, 3(4): 697-713. doi: 10.3934/environsci.2016.4.697 |
[10] | Suprava Ranjan Laha, Binod Kumar Pattanayak, Saumendra Pattnaik . Advancement of Environmental Monitoring System Using IoT and Sensor: A Comprehensive Analysis. AIMS Environmental Science, 2022, 9(6): 771-800. doi: 10.3934/environsci.2022044 |
Increasing urbanization related to land pressure and the soil arising from it are aggravating factors of flood risk in urban areas, including storm water runoff. Therefore, urban sanitation networks face an excess of water that exceeds their absorption capacity. This article deals with the effect of impermeability in densely populated urban areas and the function of a combined sewer system under higher rainfall intensities using the Storm Water Management Model (SWMM). The objective is to simulate a possible increase in rainfall intensities for reducing overflow points in the combined sewage system at the study area, which was the city of Ahmed Rachdi, Mila in Algeria. The excessive rainfall intensities were modeled using the SWMM software program to estimate maximum water volumes inside the combined sewage system of the study area. To evaluate the model's performance, a comparison process was used between the values of the flow rates of the pipelines of the sewerage system combined with the design flow rates in the current state and the flow rates of a single modeling of future events available during the study interval. The comparison results showed a good and convergent performance for these models. The results of the flooding volumes using different values of rainfall intensities and different return periods, which were 2, 5, 10 and 25 years, in the modeling of the combined sewage system are 3626, 6888, 8636 and 12676m3, respectively. The suggested scenario included increasing diameters of some pipes in the combined sewage system pipelines. The results using this scenario showed reductions in the total percentage of overflow points from the integrated sewage system of 52.42%, 40.63%, 31.83% and 20.51% using the rainfall intensities for the return periods of 2, 5, 10 and 25 years, respectively. The present study can provide technical support for using software in the planning, controlling and tests of the sewer systems, which contribute to solving the sewer systems' problems.
Sewerage systems have been used to evacuate liquid and solid wastewater since the first civilizations [1]. However, sewerage systems are still not properly developed in many cities [2], which often causes urban pollution due to sewer overflow at a given time during peak hours or during heavy rainfall. For good management of urban sewers, it is important to understand the causes of sewer overflow. However, the mastering of sewer overflow in urban areas is not simple. It is often very difficult to simulate runoff in a city's watershed due to man-made changes in topography. Variations in water use in household and business activities also cause large variability in sanitary sewer flow. Simulating the urban drainage system is therefore a difficult task. This is specifically true for the cities where rainfall is very sparse and erratic. Unexpected extreme rainfall regularly causes flash flooding in such cities. Surface inundation often occurs when rainfall exceeds the urban drainage capacity. Therefore, simulation of flow in a sewerage system is very crucial for designing and management of sewerage systems and mitigation of urban flooding [3]. Flooding is an unsteady flow that gradually varies with time and place. One of the first problems with hydraulic structures such as bridges, tunnels, embankments, dams and spillways, is whether these structures will be able to withstand the peak flood or not. Prediction of flood depth is also important for controlling floods and reducing their damage. Therefore, it is important to predict the flood depth and peak discharge for the design of flood control projects [4]. Combined sewer systems in cities are increasingly equipped with additional storage facilities or other installations necessary for keeping the wastewater treatment plants from overloading during wet weather and reducing combined sewer overflows into receiving waters. [5] showed that the management and law of urban floods, wherein urban drainage schemes are under stress due to urbanization increases, population and climate change, is one of the greatest challenges for municipalities. Also, city flooding causes damage to the surroundings and the infrastructure. In a study conducted by [6]. In addition, rainfall increases the unit weight of the soil, but the infiltration of water softened the soil and reduced its shear strength. Compared with the monitoring data of the earth pressure and surface displacement, the slope displayed a small deformation at the water flooding [7]. The saturation of the soil mass would be increased by rainfall while the shear strength of the soil decrease can cause damage to houses and buildings [8]. About the impacts of urbanization on the duration and variation of the flow (FDC) curve analysis showed rising curve percentages with 34% in urban areas due to conversion in the hydrological path and urbanization that causes an increase in the impermeable area. At the same time, the opposite happened in the rural area due to a drop in groundwater level, where groundwater is extracted for agriculture purposes. [9] studied the impacts of climate change and urbanization on the quality of water receivers in serviced areas via a combined sewage system. Study findings indicated that impenetrable urban surfaces and rainfall rates are important factors that affect the cumulative overflows of the combined sewer systems and, subsequently, the quality of water receivers. According to [10] the population increase and migration from rural to urban regions have resulted in the production of grey towns specifically composed of impervious surfaces. [11] concluded that urban flooding is self-invited because of the human appetite for more land and unregulated infrastructure construction with changes in natural land use, land cover and stream flow ways. Also, the study supported the use of separated systems for the limitation of this sewer flooding. The extensive financial losses and human injuries are stay as a result of city flooding at some stage in extreme climate and recent years have shown that world towns are quickly liable to the prevalence and consequences of pluvial floods [12,13,14].
In this article, the objectives were to evaluate the performance of the combined sewer system of the city of Ahmed Rachdi subjected to different rainfall intensities and to compare the effects of each rainfall intensity on the performance of the system to show the effects of flow changes in the combined sewer system using SWMM. The land of the Ahmed Rachdi commune is characterized by a slightly sloping topography which does not require the use of lifting stations. Then again, the geography of this territory is characterized by natural borders; the waste water flows into Oued Endja wadi, which passes near the city, causing disastrous effects on the environment. The climatic conditions of the city are characterized by a maximum of precipitation in December and January and a minimum in July and August. Spring rains are low; rare but surprising and violent summer thunderstorms cause flooding of wadis [16]. The storm water control model (SWMM) is used to meet countrywide goals in achieving good storm water management and lowering the surface runoff via many mechanisms, such as discharges retention and decrease infiltration methods, which are cause the declining level of water bodies. In a study conducted by [17], the SWMM modelling outcomes (the nodes' overflows) were taken from simulating the runoff. The examination scenarios used different rainfall intensities and return periods. The results showed that node flooding, water depths and region flooding increased proportionally with the return periods. Theobservations showed that node flooding, water depth and location flooding extended proportionally to return intervals. Also, changes in the aforementioned modelling parameters influence the flood characteristics, such as the inundation extent, the flow depth and the velocity across the model domain [18].
The city of Ahmed Rahdi (at latitude 36°23′26″ N and longitude 6°07′31″ E) is shown in Figure 1 with a total area of 89.90 km2, away about 15 km to the south-west of the region of Mila. The study area corresponds to an administrative entity, which explains the simplicity of its geographical delimitation [19].
The total area of the city of Ahmed Rachdi is about 1.376 km2, of which 0.342 km2 is permeable (25% of the total area) and 1.027 km2 impermeable (75% of the total area), of which 0.085 km2 is paved roads. The impermeable area can be classified as roofs, roads and sidewalks and the permeable area can be classified as gardens and unpaved roads. Depending on the land use and the slope of the area, the climatic conditions are characterized by maximum rainfall in December and January and minimum rainfall in July and August. Spring rains are low; summer storms rare but surprising and violent, causing floods. The average temperature in winter is 7 ℃, reaching 35 ℃ in summer. The total annual rainfall in Ahmed Rachdi is less than 95 mm [19]. The study area suffers from flooding during the rainy season. The city of Ahmed Rachdi is served by a combined sewerage system that covers all streets. Figure 2 shows the study area and the map of the integrated sewage system using Google Maps.
Ahmed Rachdi city quarters were divided into 7 sub-catchments with areas ranging from 10.25 to 37.27 Ha, as shown in Figure 4. Depths and types of manholes; diameters, roughness's, lengths and slopes of pipes and areas of sub-catchmentsare summarized in Tables 1, 2 and 3. The 410 manholes in the study area, shown in Figure 2, are made of reinforced concrete with depths ranging from 1 m to 3.82 m. The pipe diameters range from 315 mm to 800 mm and are made of PVC material.
Mandole (Node) Information | Provided data |
No. of mandoles | 343 |
Manholes types | As, Bd, Bs |
Manholes Materials | Reinforced concrete |
Maximum depth range (m) | 0.92–4.67 |
Invertelevation range (m) | 17.85–21.23 |
Normal ground level (m) | 24–29 |
Ponded area | 0 |
Pipe property | Provided data |
No of pipes in the system | 352 |
Shape | Circular |
Length range (m) | 1–65 |
Diameter range (m) | 0.25–0.6 |
Manning's roughness | 0.009 |
Pipe material type | PVC |
No | Sub-catchment property | Range |
1 | Number of sub-catchments | 7 units |
2 | Width of sub-catchments | 18.86–170.03 m |
3 | Runoff on (the total area) | 137.65 hectares |
4 | Infiltration/Inflow model [29] | Green-Ampt formula was used for the study area soil with the values of Saturated hydraulic conductivity (k) = 0.02 mm/hr., Suction head (Ψ) = 9.45 mm and Cumulative infiltration at the time t (F) = 0.321 inches. |
5 | Pervious and impervious area in sub-catchment. | Pervious area of 25% Impervious area of 75% |
6 | Slopes of sub-catchments | 0.1 to 8.0 % |
7 | Manning's roughness (n) of the sub-catchment runoff [30] | 0.011 for streets paved with smooth asphalt, 0.013 for the interior roads paved with smooth brick concrete and 0.014 for houses' roofs. |
8 | Depression Storage | 2 mm and 5 mm for impervious and pervious areas respectively. |
The spatial data of the combined sewer system and the sub-catchments of the study area were accrued from the sewage water resources directorate of Mila (MWRD) and the countrywide water resources corporation, Mila unit (NWRA). These data included the properties of the sub-catchments, pipes and nodes(manholes) and were transformed by Arc GIS as shape files, exported to AutoCAD Civil 3D to collect data in one file and then exported as (inp.) format to the SWMM program. Also, the rainfall intensities' values were taken from [15].
The representation of runoff using the SWMM is relatively easy, as shown in Figure 3. In the SWMM, each sub-catchment surface is treated as a non-linear store by an indivisible inflow (rainfall), and the surface runoff, filtering and evaporation are considered as outflows. The surface runoff occurs when the water depth in the reservoir exceeds the extra storage of depression, where the reservoir capacity (or depression storage, dp) is represented by ponds and wetting of the surface [21,22].
Hydraulic data were provided from MNSO (National Sanitation Office unit of Mila), for the combined sewage system of the study area regarding data on both the sub-catchments, nodes (manholes), pipes and the hydraulic data included the areas, widths, types, depths, diameters, lengths, invert elevations, etc. The summary of the hydraulic data for the system manholes and pipes is shown in Tables 1 and 2.
Manning's equation for partially full pipe flow was used to calculate the external inflows entering from adjacent districts to the sewer systems in the study area, where they will join as a direct inflow in the SWMM.
To model the combined sewage system by the SWMM, the dry weather wastewater flow will be adding to the appropriate conveyance nodes in this sewer system. The dry weather waste water flows typically represent the locations where the collected sewage discharges to the trunk sewer. Variations in sewage generation or time pattern occur through the season, month and day, depending on water consumption rate. There is a wide variance of water consumption quantities because some months have a high consumption rate, in the hot or warm months. Also, the expected high demand for water supply happens on specific days, like Fridays and holidays. Also, the variation occurs in the same hours of the day; where the peak demand occurs through one day and its hour's occurrence depend on city characteristics and people's habits. The ratio between the waste water generation to water consumption for each capita ranges from 70% to 100% [25]. In Ahmed Rachdi, the average ratio is about 80% [16]. Usually, in the study area, the peaks of water consumption occur between 6 and 10 in the morning, when people's activities are beginning in the day and between 6 and 10 in the evening. The minimum water consumption always happens between midnight and 4 in the morning [16]. An hourly pattern of the dry weather flow (DWF) prepared by [23] is approximately proper for the present study area and will be used in the combined sewage system modeling by the SWMM. The average daily water consumption in Ahmed Rachdi city based on the available data from [16] is about 275 liters per capita per day. In 2009, the number of people in the study area arrived at 15900 people, and the yearly growth factor for Ahmed Rachdi city during the previous decade was equal to 3.5% [26]. The forecast of the study area population in the year 2020 can be estimated at about 17541 people who are used in calculating DWF.
The hydrological data modeling target represents the best presentation of the precipitation in the study area to estimate the water movement in sub-catchments, the runoff and the run on. The modeling of the hydrological data includes the rainfall intensities and sub-catchments' properties.
Since the sewerage network must be able to evacuate domestic and rain-fed water, it is important to know the quantities with sufficient rigor. For this, rainfall measurements are essential to be able to characterize the events to which the network will react and to correctly model this reaction. All the precipitation resulting from the same meteorological disturbance constitutes the rain [27]. The latter are defined as sudden and rare rains, generally of short time; and can vary from one minute to several hours. Rainfall is considered the source of the most runoff. The rainfall intensity has a wide range, according to the geographic location and the seasons of the year. Sometimes, the rainfall intensity is very high and causes damages to the facilities and human lives in the precipitation region, so it is a very concerning case. In the present SWMM, the high rainfall intensities for different return periods, which are 2, 5, 10 and 25 years and for duration of 2 hours with a time of concentration (Tc) of 5 minutes were taken from [28]. where data of Ahmed Rachdi city annual precipitation has analysis using Grumble distribution, based on the annual precipitation data that collected by [28] Algerian National Meteorological Office for the duration from 1996 to 2021.
In the SWMM, sub-catchments are hydrologic units of land whose topography and drainage system dispose the direct surface runoff to a single discharge point. These discharge points are designated as nodes and carry the runoff through links to another node which can be a junction of two or more than two conduits or discharge points of any other sub-catchment. Unlike a natural watershed, in urban watershed, the overland flow is often intercepted by the drains, which carry the water to the designated outlet. This phenomenon of interception of surface runoff by drains should be taken into account while discretizing sub-watersheds in the SWMM. Figure 4 shows the watershed identification and sub-catchment discretization in the SWMM model setup for simulating the rainfall–runoff response of the catchment.
Properties associated with a sub-catchment in the SWMM are area, width, % slope, % imperviousness, soil properties, Manning's n for impervious and pervious surfaces for overland flow, depth of depression storage in pervious and impervious area and % impervious area with no depression storage. Areas of the sub-catchments discretized in the present study range from 10.25 to 37.26 ha. The characteristics of the study area are shown in Tables 3 and 4.
Rainfall intensities' return period | Normalized Mean Square Error (NMSE) | R | Coefficient of determination(%) | Root Mean Square Error (RMSE) |
2 | 0.7110 | 0.9667 | 93.31 | 0.0361 |
5 | 0.4112 | 0.8234 | 67.78 | 0.0272 |
10 | 0.2428 | 0.8023 | 64.36 | 0.0244 |
25 | 0.1988 | 0.7235 | 52.32 | 0.0236 |
There was a lack of data required for calibration and validation of the SWMM. In the present study, for the model's validation, a comparison was conducted between the pipes' flow rates in the modeling of the combined sewage system using different rainfall intensities, design flow rates and the lines' flow rates from event modeling, using the methods of Normalized Mean Square Error (NMSE), Coefficient of determination (R2) and Root Mean Square Error (RMSE) to evaluate the SWMM performance. These methods were used in the studies conducted by [31,32,33,34,35]. The comparison results are shown in Table 4.
The results of the comparison between the design flow rates of the pipes and max flow rates of the SWMM using rainfall intensities with different return periods using the NMSE method showed that all R values ranged between 0.7235 and 0.9667 (the acceptable limitation value from 0–1), and the NMSE values ranged between 0.1988 and 0.7110 (the acceptable limitation value is less than 1.5). Also, the results of the NMSE showed that rainfall intensity with a 25-year return period gave the minimum value of the NMSE, which was 0.1988. The NMSE results appear that the best performance by the SWMM model was by using the rainfall intensity with 25 years return period. The coefficient of determination (R2) values ranged between 52.32% and 93.31% for the different return periods, where the results of R2 showed that at the SWMM using the rainfull intensity for the return period of 10 years, the model gave the high value of R2 and best performance, this when the maximum flow rates values have close to the values of the design flow rates. All the values of RMSE were close to zero for all SWMM models for the different return periods, ranging between 0.0236 and 0.0361 (the acceptable limitation value is less than 1), where the results of RMSE showed that when using rainfall intensity with a 25-year return period, the SWMM model gave a better performance. Only one event of precipitation occurred during the study interval, on 28/11/2020. The rainfall depth of the event was 55 mm, and the rainfall duration was 120 minutes. The event data was used as an accumulative value in the Time Series of the SWMM. The comparison was conducted using the RMSE and the Coefficient of determination (R2) methods between the max flow rates in the pipes of the event model with the different rainfall intensities models. The comparison results using RMSE and R2 are shown in Table 5 and Figure 5.
Rainfall intensity return period | RMSE |
2 | 0.0503 |
5 | 0.0391 |
10 | 0.0573 |
25 | 0.0612 |
The obtained results by using the Root Mean Square Error (RMSE) method showed low values of RMSE (the acceptable limitation value is less than 1) for the relationship between pipes flow rate of the rainfall event and flow rate using the different rainfall intensities for the additional return periods and this indicates a good performance of the SWMM models. The value using the rainfall intensity with a return period of 5 years, which is 0.0391, was closer to the zero value than other RMSE values.
The variances in R2 values indicate the convergence and divergence between the values of the rainfall intensity used in the event model and the values of the different rainfall intensities used in SWMM, where the increase of the R2 value indicates the convergence of the rainfall intensity values used in the SWMM with the rainfall intensity values used in the event model, and the decrease of the R2 value indicates that the rainfall intensity values used in the SWMM are getting away from the rainfall intensity values used in the event model. The value of R2 equal 70.22, utilizing the rainfall intensity for the return period of 5 years, gave the best coefficient of determination (R2), which means that the rainfall event is close to the rainfall intensity for the return period of 5 years. The obtained results gave more credibility to the SWMM in this study.
In the SWMM modeling process for the combined sewage system, there are two sources of wastewater: first, the run-off on the sub-catchments due to the precipitation and, second, the wastewater produced from domestic uses. When these liquids reach volumes that exceed the design capacity of the sewer system, it causes floods in the nodes of the sewage system. This is what happened within varying percentages in the combined sewage system of the study area when using the different rainfall intensities for different return periods. The node flooding, in most cases, is caused by the misuse and the irregular distribution of sewage quantities in the combined sewage system in the study area, where the flooding in some nodes occurs by exceeding the criteria of the sewer system design. For example, by observing the nodes during the rainfall event, the flooding occurs caused by the direct connections of the home sewers to the nodes, not on houses connections (sewer pipes).The remaining volume of the runoff is distributed between infiltration in the soil, depression storage and the sewage liquid volume discharged by the combined sewage system. Table 6 and Figure 6 list the volumes of the total runoff and other above.
Rainfall intensity return period (years) |
Total Runoff (m3) | Total Node Flooding (m3) |
Percentage of flooding nodes / total nodes in combined sewage system | Remaining volume (m3) |
2 | 5043 | 3626 | (33/343) ≈ 10% | 1417 |
5 | 10510 | 6888 | (87/343) ≈ 25% | 3622 |
10 | 13780 | 8636 | (111/343) ≈ 32% | 5144 |
25 | 22560 | 12676 | (145/343) ≈ 42% | 9884 |
The results in Table 7 show that the total of the over sub-catchments runoff, node flooding volume, number of flooded nodes and the remaining from the total runoff volume increased with the increase of the rainfall intensity having the high return period. The rainfall intensity for the return period of 25 years recorded a high value for total runoff volume (22,560 m3). Total node flooding volume was 12,676 m3 and the number of flooding nodes was 145, representing 42% of the total system nodes. The remaining volume from the total runoff volume was 9884 m3 and this is considered a logical result because of the increase in the runoff rates. Figure 6 shows percentages for the total flooding volume from nodes comparing with the remaining runoff from the total runoff volume due to precipitation, where the ratio of total nodes flooding volume comparing with the total runoff decreases with the higher rainfall intensity having the higher return period and these results agree with results of [36,37].
Rainfall intensity return period | Total flooding volume before pipes' diameter change (m3) | Total flooding volume after pipes' diameter change (m3) | Percentage of flooding reduction efficiency (%) |
2 | 3626 | 1725 | 52.42 |
5 | 6888 | 4089 | 40.63 |
10 | 8636 | 5887 | 31.83 |
25 | 12676 | 10075 | 20.51 |
Figures 7, 8, 9 and 10 presents' hydrographs of the maximum flooding rate occurring from the SWMM for the combined sewage system using the four rainfall intensities.
As shown in all hydrographs, the flooding flow begins 10 minutes after the simulation start. The node flooding occurs at a late time of the simulation starting (at minute 20) when using the rainfall intensity of the return period of 2 years due to the low value of this rainfall intensity, which is considered less than other rainfall intensities for the return periods of 5, 10 and 25 years (at minute 15). Also, the maximum value of the flooding flow rate using the rainfall intensity for the return period of 2 years is about 1100 CMS and this value is considering the lowest among the values obtained from the simulations of the three other rainfall intensities having 5, 10 and 25 year return periods), which were 2.400, 3.300 and 4.100 CMS respectively. All rainfall intensities are equaled approximately at the same time in the flooding rate values in the minute of 150, where equal to 0.05 CMS, after that time, the values continue to decrease until the flooding flow rate stops when the wastewater flow rate arriving at the design capacity for the system.
The previously mentioned sewer flooding volumes are submissive to the increase due to population behaviors (activities and habits), and these behaviors can cause an increase in the flooding rate during dry and rainy weather. The population behaviors affect the sewer systems' operation such as the misuse and illegal sewer connections, closing of the inlets of sewer systems by trash, wastes from commercial activities, spills of untreated liquid and solid wastes, such as cars oils, butchers waste and food industries such as vinegar and vegetable market waste, ..., etc. Also, the above reasons cause pollution in the rainwater during the rainy weather, which is finally deposited in the receiving environment of Oued Endja (at a distance of 4 km from the center of Ahmed Rachdi city [3] at some point of the wet weather, that is ultimately deposited within the receiving environment which is Ouedendja (at a distance of four km from the center of Ahmed Rachdi city [24].
All of the above causes, along with the lack of periodic maintenance of sewer networks, the weakness of municipal waste collection services and the significant increase in population growth in the study area, increase the pressure on the sewer networks in terms of both quantity and quality and affect the operating performance of the sanitation network in the city of Ahmed Rachdi during rainy periods. Figure11 shows the places of flooding and the effects of the population's activities on the sewer networks during the dry period and rainy weather in the study area.
The scenario of changing pipes' diameters has been used before in the study of [38] as one of the solutions for reducing the overflows in the sewer systems. This scenario involves increasing the diameters of some pipes in pipelines of the combined sewage system, which includes pipes that have diameters of 0.25, 0.4 and 0.6 m, where the diameter change was based on the balance between the economic selection and the practical solution. The targeted pipelines collect rainwater either from large-space areas or from other sewage pipelines that come from the sub-streets, which have similar diameters to the diameters of the targeted pipelines. This causes a slow discharging of the sewage in these pipelines, which presses on the targeted pipeline's nodes and causes node overflow. Using this scenario achieves two targets:
1. The first target is to reduce the overflow quantities from the nodes of the combined sewage system produce during rainfall events and therefore, reduce the flooding volume in the study area.
2. The second target of this scenario is to decrease pollutant concentrations in the study area that come from the combined sewage system node overflow, where these pollutants inflow to the storm water system by the runoff during the rainy weather.
Figure 12 shows the mechanism of applying the scenario on the pipelines of the combined sewage system. The pipelines with green color represent the lines that need to change their pipes' diameters from 0.25 m to 0.4 m, and the pipelines with yellow color represent the lines that need to change their pipes' diameters from 0.6 m to 0.8 m. Also, lines with red color represent the lines that need to change their pipes' diameters from 0.5 m to 0.6 m.
Table 7 shows the results obtained from using this scenario in the SWMM to reduce the total flooding volumes in the combined sewage system nodes using the different rainfall intensities for the different return periods.
The results in Table 7 present the efficiency of using the scenario in reducing the combined sewage system flooding, where the results appear that the scenario efficiency in the flooding reduction was high when using the model of the rainfall intensity for the return period of 2 years, which was 52.42%, more than the other models using different rainfall intensities. Also, these results showed that the scenario efficiency is negatively related to the return period of the rainfall intensity. In other words, the scenario efficiency decreases with the increase of the rainfall intensity return period because of the increase in the runoff produced by the higher rainfall intensity.
In this paper, the objectives were to evaluate the performance of combined sewer system subjected to different intensities of precipitations and to compare the effects of each rainfall intensity on the system performance to show the effect of changes flow in a combined sewer system and also take into consideration variations in flow that exceed the design discharge capacity and achieve to identify overflow points in the system and correct anomalies in the combined sewer system.
The storm water management model was used to simulate the Ahmed Rachdi city combined sewer system using rainfall intensities with return times of 2, 5, 10 and 25 years.
The comparison results, between the designed and the predicted flow rates, showed that the obtained values of NMSE ranged between 0.1988 and 0.7110. The obtained values of the Coefficients of determination ranged between 52.32% and 93.31%, and the values of RMSE ranged between (0.0236–0.0361). Also, the comparison results, between the design flow rates and the rainfall event flow rates on 28/11/2020, using the Normalized Mean Square Error (NMSE) and the Coefficient of determination (R2), showed that the obtained values of NMSE ranged between 0.0391 and 0.0613, and the values of the Coefficient of determination ranged between 36.07% and 71.93%.The results acquired from the combined sewage system models by SWMM confirmed the flooding volumes of the nodes using the rainfall intensities for the return periods of 2, 5, 10 and 25 years were 3626, 6888, 8636 and 12676 m3, respectively.
The suggested scenario of the simulation included increasing diameters of some pipes in the combined sewage system pipelines. The results of this scenario showed a reduction in the total flooding volumes percentage of overflow points from the combined sewage system of 52.42%, 40.63%, 31.83% and 20.51%. Using the rainfall intensities for the return periods of 2, 5, 10 and 25 years respectively.
The SWMM played a key role in the simulation of urban flooding within the study area, providing results which are in agreement with observations and therefore appropriate for use in the estimation of urban flooding.
The present study is expected to provide a technical solution for experts, engineers and decision makers in order to identify flood problems controlling and tests of the sewer systems, which contribute to solving the sewer system's problems.
The authors declare that there is no acknowledgment to disclose.
The authors declare no conflict of interest.
[1] | Metcalf, Eddy, Inc. (1981) Wastewater engineering: collection and pumping of waste water, Tchobanoglous, G., Ed. New York: McGraw-Hill Book Company. |
[2] |
Burian S, Edwards F (2002) Historical Perspectives of Urban Drainage. In Global Solutions for Urban Drainage 2002: 1-16. https://doi.org/10.1061/40644(2002)284 doi: 10.1061/40644(2002)284
![]() |
[3] | Hussein A, Obaid S, Shahid KN, et al. (2014) Modeling sewerage overflow in an urban residential area using storm water management model. MalaysianJournal of Civil Engineering 26: 163-171. |
[4] | Safavi HR (2014) Engineering hydrology, 4thedn. Isfahan University of Technology. |
[5] |
Hassan WH, Nile BK, Al-Masody BA (2017) Climate change effect on storm drainage networks by stormwater management model. Environmental Engineering Research 22: 393-400. https://doi.org/10.4491/eer.2017.036 doi: 10.4491/eer.2017.036
![]() |
[6] |
Fasheng M, Yiping W, Török Á, et al. (2022) Centrifugal model test on a riverine landslide in the Three Gorges Reservoir induced by rainfall and water level fluctuation.Geoscience Frontiers 13: 101378. https://doi.org/10.1016/j.gsf.2022.101378 doi: 10.1016/j.gsf.2022.101378
![]() |
[7] |
Miao F, Wu Y, Xie Y, et al. (2018) Prediction of landslide displacement with steplike behavior based on multialgorithm optimization and a support vector regression model. Landslides 15: 475-488. https://doi.org/10.1007/s10346-017-0883-y doi: 10.1007/s10346-017-0883-y
![]() |
[8] |
Rosburg TT, Nelson PA, Bledsoe BP (2017) Effects of urbanization on flow duration and stream flashiness: a case study of Puget Sound streams, western Washington, USA. JAWRA Journal of the American Water Resources Association 53: 493-507. https://doi.org/10.1111/1752-1688.12511 doi: 10.1111/1752-1688.12511
![]() |
[9] |
Salerno F, Gaetano V, Gianni T (2018) Urbanization and climate change impacts on surface water quality: Enhancing the resilience by reducing impervious surfaces. Water research 144: 491-502. https://doi.org/10.1016/j.watres.2018.07.058 doi: 10.1016/j.watres.2018.07.058
![]() |
[10] |
Chang NB, Lu JW, Chui TFM, et al. (2018) Global policy analysis of low impact development for stormwater management in urban regions. Land Use Policy 70: 368-383. https://doi.org/10.1016/j.landusepol.2017.11.024 doi: 10.1016/j.landusepol.2017.11.024
![]() |
[11] |
Rangari VA, Sridhar V, Umamahesh NV, et al. (2019) Floodplain mapping and management of urban catchment using HEC-RAS: a case study of Hyderabad city. Journal of The Institution of Engineers (India): Series A 100: 49-63. https://doi.org/10.1007/s40030-018-0345-0 doi: 10.1007/s40030-018-0345-0
![]() |
[12] | Ho G (2000) International Source Book on Environmentally Sound Technologies. Forwastewater and stormwater management. UNEP Division of Technology, Industry and Economics. International Environmental Technology Centre, Osaka/Shiga, 151. |
[13] |
Jia H, Yao H, Shaw LY (2013) Advances in LID BMPs research and practice for urban runoff control in China. Frontiers of Environmental Science & Engineering 7: 709-720. https://doi.org/10.1007/s11783-013-0557-5 doi: 10.1007/s11783-013-0557-5
![]() |
[14] |
Barredo JI (2007) Major flood disasters in Europe: 1950-2005. Natural Hazards 42: 125-148. https://doi.org/10.1007/s11069-006-9065-2 doi: 10.1007/s11069-006-9065-2
![]() |
[15] | National Water Resources Agency (2003) Synthesis study on surface water resources in northern Algeria (Study report), Algeria: Algiers, 36. |
[16] | National Sanitation Office unit of Mila (1993) Report of Directories wastewater network. |
[17] |
Yang Y, Sun L, Li R, et al. (2020) Linking a stormwater management model to a novel two-dimensional model for urban pluvial flood modelling. International Journal of Disaster Risk Science 11: 508-518. https://doi.org/10.1007/s13753-020-00278-7 doi: 10.1007/s13753-020-00278-7
![]() |
[18] |
Adeniyi AG, Michael OD, Assela P (2016) Coupled 1D-2D hydrodynamic inundation model for sewer overflow: Influence of modeling parameters. Water Science 29: 146-155. https://doi.org/10.1016/j.wsj.2015.12.001 doi: 10.1016/j.wsj.2015.12.001
![]() |
[19] | Mila Water Resources Directorate (2009) Report of Directories |
[20] | Directorate of urban planning, architecture and construction, wilaya of Mila (2022). |
[21] | Rossman LA (2010) Stormwater management model user's manual, version 5.0 (p. 276). Cincinnati: National Risk Management Research Laboratory, Office of Research and Development, US Environmental Protection Agency. |
[22] | Lockie T (2009) Catchment modelling using SWMM. In Modelling Stream at the 49th Water New Zealand Annual Conference and Expo. |
[23] | Choi NJ (2016) Understanding sewer infiltration and inflow using impulse response functions derived from physics-based models (Doctoral dissertation, the University of Illinois at Urbana-Champaign). |
[24] | MNSO (National Sanitation Office unit of Mila), (2017). Report of Directories wastewater network. |
[25] | Steel EW, McGhee TJ (1979) Water Supply and. Sewerage. (McCraw). |
[26] | Housing statistics (2020) report Communal People's Assembly of the city of Ahmed Rachdi. |
[27] | World Health Organization (2011) Guidance on water supply and sanitation in extreme weather events, World Health Organization, Regional Office for Europe. |
[28] | Algerian National Meteorological Office.(ANMO).(2021).Bultin meteorologic |
[29] |
Rawls WJ, Brakensiek DL, Miller N (1983) Green-Ampt infiltration parameters from soils data. Journal of hydraulic engineering 9: 62-70. https://doi.org/10.1061/(ASCE)0733-9429(1983)109:1(62) doi: 10.1061/(ASCE)0733-9429(1983)109:1(62)
![]() |
[30] | Richard H (1989) Hydrologic Analyses and Design Englewood Cliffs, New Jersey, 9-10. |
[31] |
Zaini N, Malek MA, Yusoff M (2015)Application of computational intelligence methods in modeling river flow prediction: A review. In 2015International Conference on Computer, Communications, and Control Technology (I4CT) 2015: 370-374. https://doi.org/10.1109/I4CT.2015.7219600 doi: 10.1109/I4CT.2015.7219600
![]() |
[32] |
Badieizadeh S, Bahrehmand A, Ahmad DA (2016) Calibration and Evaluation of the HydrologicHydraulic Model SWMM to Simulate Runoff (Case study: Gorgan). Journal of Watershed Management Research 2016: 1-10. https://doi.org/10.29252/jwmr.7.14.10 doi: 10.29252/jwmr.7.14.10
![]() |
[33] | Kourtis IM, Kopsiaftis G, Bellos V, et al. (2017) Calibration and validation of SWMM model in two urban catchments in Athens, Greece. In International Conference on Environmental Science and Technology (CEST). |
[34] | Taatpour F, Kouhanestani ZK, Armin M (2019) Evaluating the Performance of Collection and Disposal of Surface Runoff Network Using SWMM Model (Case Study: the City of Likak, Kohgiluyeh and Boyer Ahmad Province). Irrigation Sciences and Engineering 42: 33-48. |
[35] |
Hendrawan AP (2020) Alternatives of flood control for the Line river, city of Toboali (a case study of the Rawabangun region). In IOP Conference Series: Earth and Environmental Science 437: 012047. https://doi.org/10.1088/1755-1315/437/1/012047 doi: 10.1088/1755-1315/437/1/012047
![]() |
[36] |
Nile BK (2018) Effectiveness of hydraulic and hydrologic parameters in assessing storm system flooding. Advances in Civil Engineering, 2018. https://doi.org/10.1155/2018/4639172 doi: 10.1155/2018/4639172
![]() |
[37] | Nile BK, Hassan WH, Alshama GA (2019) Analysis of the effect of climate change on rainfall intensity and expected flooding by using ANN and SWMM programs. ARPN Journal of Engineering and Applied Sciences 14: 974-984. |
[38] | Li YW, You XY, Ji M, et al. (2010) Optimization of rainwater drainage system based on SWMM model. China Water & Wastewater 26: 40-43. |
1. | Nejat Zeydalinejad, Akbar A. Javadi, James L. Webber, Global perspectives on groundwater infiltration to sewer networks: A threat to urban sustainability, 2024, 262, 00431354, 122098, 10.1016/j.watres.2024.122098 | |
2. | Amir Karim, Mostafa Babaeian Jelodar, Teo Susnjak, Monty Sutrisna, A Review of Climate Change Impact Assessment and Methodologies for Urban Sewer Networks, 2025, 25901230, 104625, 10.1016/j.rineng.2025.104625 |
Mandole (Node) Information | Provided data |
No. of mandoles | 343 |
Manholes types | As, Bd, Bs |
Manholes Materials | Reinforced concrete |
Maximum depth range (m) | 0.92–4.67 |
Invertelevation range (m) | 17.85–21.23 |
Normal ground level (m) | 24–29 |
Ponded area | 0 |
Pipe property | Provided data |
No of pipes in the system | 352 |
Shape | Circular |
Length range (m) | 1–65 |
Diameter range (m) | 0.25–0.6 |
Manning's roughness | 0.009 |
Pipe material type | PVC |
No | Sub-catchment property | Range |
1 | Number of sub-catchments | 7 units |
2 | Width of sub-catchments | 18.86–170.03 m |
3 | Runoff on (the total area) | 137.65 hectares |
4 | Infiltration/Inflow model [29] | Green-Ampt formula was used for the study area soil with the values of Saturated hydraulic conductivity (k) = 0.02 mm/hr., Suction head (Ψ) = 9.45 mm and Cumulative infiltration at the time t (F) = 0.321 inches. |
5 | Pervious and impervious area in sub-catchment. | Pervious area of 25% Impervious area of 75% |
6 | Slopes of sub-catchments | 0.1 to 8.0 % |
7 | Manning's roughness (n) of the sub-catchment runoff [30] | 0.011 for streets paved with smooth asphalt, 0.013 for the interior roads paved with smooth brick concrete and 0.014 for houses' roofs. |
8 | Depression Storage | 2 mm and 5 mm for impervious and pervious areas respectively. |
Rainfall intensities' return period | Normalized Mean Square Error (NMSE) | R | Coefficient of determination(%) | Root Mean Square Error (RMSE) |
2 | 0.7110 | 0.9667 | 93.31 | 0.0361 |
5 | 0.4112 | 0.8234 | 67.78 | 0.0272 |
10 | 0.2428 | 0.8023 | 64.36 | 0.0244 |
25 | 0.1988 | 0.7235 | 52.32 | 0.0236 |
Rainfall intensity return period | RMSE |
2 | 0.0503 |
5 | 0.0391 |
10 | 0.0573 |
25 | 0.0612 |
Rainfall intensity return period (years) |
Total Runoff (m3) | Total Node Flooding (m3) |
Percentage of flooding nodes / total nodes in combined sewage system | Remaining volume (m3) |
2 | 5043 | 3626 | (33/343) ≈ 10% | 1417 |
5 | 10510 | 6888 | (87/343) ≈ 25% | 3622 |
10 | 13780 | 8636 | (111/343) ≈ 32% | 5144 |
25 | 22560 | 12676 | (145/343) ≈ 42% | 9884 |
Rainfall intensity return period | Total flooding volume before pipes' diameter change (m3) | Total flooding volume after pipes' diameter change (m3) | Percentage of flooding reduction efficiency (%) |
2 | 3626 | 1725 | 52.42 |
5 | 6888 | 4089 | 40.63 |
10 | 8636 | 5887 | 31.83 |
25 | 12676 | 10075 | 20.51 |
Mandole (Node) Information | Provided data |
No. of mandoles | 343 |
Manholes types | As, Bd, Bs |
Manholes Materials | Reinforced concrete |
Maximum depth range (m) | 0.92–4.67 |
Invertelevation range (m) | 17.85–21.23 |
Normal ground level (m) | 24–29 |
Ponded area | 0 |
Pipe property | Provided data |
No of pipes in the system | 352 |
Shape | Circular |
Length range (m) | 1–65 |
Diameter range (m) | 0.25–0.6 |
Manning's roughness | 0.009 |
Pipe material type | PVC |
No | Sub-catchment property | Range |
1 | Number of sub-catchments | 7 units |
2 | Width of sub-catchments | 18.86–170.03 m |
3 | Runoff on (the total area) | 137.65 hectares |
4 | Infiltration/Inflow model [29] | Green-Ampt formula was used for the study area soil with the values of Saturated hydraulic conductivity (k) = 0.02 mm/hr., Suction head (Ψ) = 9.45 mm and Cumulative infiltration at the time t (F) = 0.321 inches. |
5 | Pervious and impervious area in sub-catchment. | Pervious area of 25% Impervious area of 75% |
6 | Slopes of sub-catchments | 0.1 to 8.0 % |
7 | Manning's roughness (n) of the sub-catchment runoff [30] | 0.011 for streets paved with smooth asphalt, 0.013 for the interior roads paved with smooth brick concrete and 0.014 for houses' roofs. |
8 | Depression Storage | 2 mm and 5 mm for impervious and pervious areas respectively. |
Rainfall intensities' return period | Normalized Mean Square Error (NMSE) | R | Coefficient of determination(%) | Root Mean Square Error (RMSE) |
2 | 0.7110 | 0.9667 | 93.31 | 0.0361 |
5 | 0.4112 | 0.8234 | 67.78 | 0.0272 |
10 | 0.2428 | 0.8023 | 64.36 | 0.0244 |
25 | 0.1988 | 0.7235 | 52.32 | 0.0236 |
Rainfall intensity return period | RMSE |
2 | 0.0503 |
5 | 0.0391 |
10 | 0.0573 |
25 | 0.0612 |
Rainfall intensity return period (years) |
Total Runoff (m3) | Total Node Flooding (m3) |
Percentage of flooding nodes / total nodes in combined sewage system | Remaining volume (m3) |
2 | 5043 | 3626 | (33/343) ≈ 10% | 1417 |
5 | 10510 | 6888 | (87/343) ≈ 25% | 3622 |
10 | 13780 | 8636 | (111/343) ≈ 32% | 5144 |
25 | 22560 | 12676 | (145/343) ≈ 42% | 9884 |
Rainfall intensity return period | Total flooding volume before pipes' diameter change (m3) | Total flooding volume after pipes' diameter change (m3) | Percentage of flooding reduction efficiency (%) |
2 | 3626 | 1725 | 52.42 |
5 | 6888 | 4089 | 40.63 |
10 | 8636 | 5887 | 31.83 |
25 | 12676 | 10075 | 20.51 |