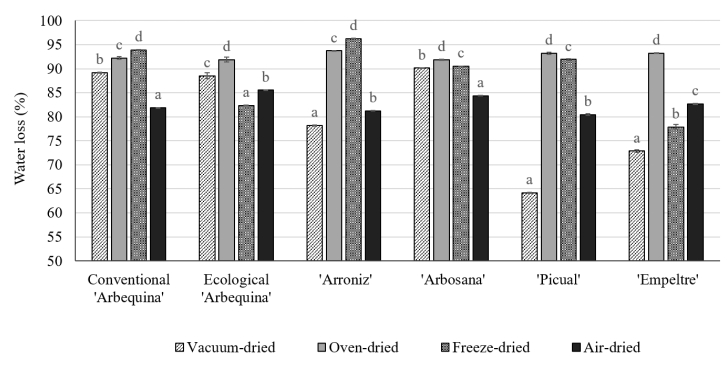
Up to 5% of the total olive weight arriving at the mill is discarded as leaves. Interest in the possible uses of these residues is growing, because they constitute a potential cheap and abundant source of compounds with high total antioxidant capacity (TAC) associated with total phenolic content (TPC) and biophenols such as hydroxytyrosol (HC) and oleuropein (OC), which could be used as nutraceuticals or as natural substitutes for synthetic antioxidants. However, studies that characterize specific cultivars, interannual variability, and different drying methods are lacking. This work investigates the TAC, TPC, HC and OC in olive (Olea europaea L.) leaves under four drying methods (vacuum-drying, oven-drying, freeze-drying and air-drying). Leaves were collected from cultivars 'Arbequina' grown under organic methods and from 'Arroniz', 'Empeltre', 'Arbosana', 'Picual' and 'Arbequina' grown under conventional systems. Among fresh samples, 'Arbosana' leaves presented the highest TPC (34.0 ± 1.1 mg gallic acid equivalents/g dry weight (DW)) and TAC (146 ± 20 μmol Trolox equivalents/g DW) and the lowest interannual variability of the TPC (3.2%). The four tested drying methods were also compared as the effect on TPC, TAC, HC and OC. Freeze-drying and air-drying best preserved TPC and TAC in olive leaves. However, air-drying maintained greater OC (14–40 mg/g DW) than freeze-drying (3–20 mg/g DW). Air-dried ecological 'Arbequina' leaves exhibited the highest TPC and TAC. Consequently, this cultivar presented more valorization opportunities as a source of nutraceuticals or natural antioxidants.
Citation: Itxaso Filgueira-Garro, Carolina González-Ferrero, Diego Mendiola, María R. Marín-Arroyo. Effect of cultivar and drying methods on phenolic compounds and antioxidant capacity in olive (Olea europaea L.) leaves[J]. AIMS Agriculture and Food, 2022, 7(2): 250-264. doi: 10.3934/agrfood.2022016
[1] | Ramadan ElGamal, Omar A. Hamed, Ahmed M. Rayan, Chuanping Liu, Sameh Kishk, Salim Al-Rejaie, Gamal ElMasry . Effect of convective and vacuum drying on some physicochemical and phytochemical characteristics of peppermint leaves. AIMS Agriculture and Food, 2025, 10(1): 17-39. doi: 10.3934/agrfood.2025002 |
[2] | Evi Mintowati Kuntorini, Laurentius Hartanto Nugroho, Maryani, Tri Rini Nuringtyas . Maturity effect on the antioxidant activity of leaves and fruits of Rhodomyrtus tomentosa (Aiton.) Hassk.. AIMS Agriculture and Food, 2022, 7(2): 282-296. doi: 10.3934/agrfood.2022018 |
[3] | Alexandros Tsoupras, Eirini Panagopoulou, George Z. Kyzas . Olive pomace bioactives for functional foods and cosmetics. AIMS Agriculture and Food, 2024, 9(3): 743-766. doi: 10.3934/agrfood.2024040 |
[4] | Pham Thi Thu Ha, Nguyen Thi Bao Tran, Nguyen Thi Ngoc Tram, Vo Hoang Kha . Total phenolic, total flavonoid contents and antioxidant potential of Common Bean (Phaseolus vulgaris L.) in Vietnam. AIMS Agriculture and Food, 2020, 5(4): 635-648. doi: 10.3934/agrfood.2020.4.635 |
[5] | Zina Guermazi, Cinzia Benincasa . Olive pomace as spreadable pulp: A new product for human consumption. AIMS Agriculture and Food, 2018, 3(4): 441-454. doi: 10.3934/agrfood.2018.4.441 |
[6] | Ebrahim Falahi, Zohre Delshadian, Hassan Ahmadvand, Samira Shokri Jokar . Head space volatile constituents and antioxidant properties of five traditional Iranian wild edible plants grown in west of Iran. AIMS Agriculture and Food, 2019, 4(4): 1034-1053. doi: 10.3934/agrfood.2019.4.1034 |
[7] | Rossaporn Jiamjariyatam, Orachorn Mekkerdchoo, Pakkapong Phucharoenrak, Lu Zheng . Effects of freeze-drying and vacuum-drying on the quality, total phenolic contents, and antioxidant activities of bee honey in northern Thailand. AIMS Agriculture and Food, 2024, 9(2): 430-444. doi: 10.3934/agrfood.2024025 |
[8] | Hesti Kurniasari, Wahyudi David, Laras Cempaka, Ardiansyah . Effects of drying techniques on bioactivity of ginger (Zingiber officinale): A meta-analysis investigation. AIMS Agriculture and Food, 2022, 7(2): 197-211. doi: 10.3934/agrfood.2022013 |
[9] | Nicolas Nagahama, Bruno Gastaldi, Michael N. Clifford, María M. Manifesto, Renée H. Fortunato . The influence of environmental variations on the phenolic compound profiles and antioxidant activity of two medicinal Patagonian valerians (Valeriana carnosa Sm. and V. clarionifolia Phil.). AIMS Agriculture and Food, 2021, 6(1): 106-124. doi: 10.3934/agrfood.2021007 |
[10] | Febiani Dwi Utari, Dessy Agustina Sari, Laeli Kurniasari, Andri Cahyo Kumoro, Mohamad Djaeni, Ching-Lik Hii . The enhancement of sappanwood extract drying with foaming agent under different temperature. AIMS Agriculture and Food, 2023, 8(1): 214-235. doi: 10.3934/agrfood.2023012 |
Up to 5% of the total olive weight arriving at the mill is discarded as leaves. Interest in the possible uses of these residues is growing, because they constitute a potential cheap and abundant source of compounds with high total antioxidant capacity (TAC) associated with total phenolic content (TPC) and biophenols such as hydroxytyrosol (HC) and oleuropein (OC), which could be used as nutraceuticals or as natural substitutes for synthetic antioxidants. However, studies that characterize specific cultivars, interannual variability, and different drying methods are lacking. This work investigates the TAC, TPC, HC and OC in olive (Olea europaea L.) leaves under four drying methods (vacuum-drying, oven-drying, freeze-drying and air-drying). Leaves were collected from cultivars 'Arbequina' grown under organic methods and from 'Arroniz', 'Empeltre', 'Arbosana', 'Picual' and 'Arbequina' grown under conventional systems. Among fresh samples, 'Arbosana' leaves presented the highest TPC (34.0 ± 1.1 mg gallic acid equivalents/g dry weight (DW)) and TAC (146 ± 20 μmol Trolox equivalents/g DW) and the lowest interannual variability of the TPC (3.2%). The four tested drying methods were also compared as the effect on TPC, TAC, HC and OC. Freeze-drying and air-drying best preserved TPC and TAC in olive leaves. However, air-drying maintained greater OC (14–40 mg/g DW) than freeze-drying (3–20 mg/g DW). Air-dried ecological 'Arbequina' leaves exhibited the highest TPC and TAC. Consequently, this cultivar presented more valorization opportunities as a source of nutraceuticals or natural antioxidants.
Annually the olive oil industry produces more than 3 million tons of oil worldwide. The main production area is located in the Mediterranean countries of Spain, Italy, Greece, Tunisia and Turkey [1]. Olive leaves constitute as much as 5% of the total olive (Olea europaea L.) weight that enters an olive mill. It is discarded along with another 75% as olive pomace; only the 20% is extracted to produce olive oil [2]. Consequently, the olive oil industry generates large amounts of wastes. Olive leaves, which are separated from the olives before olive oil production, present a high concentration of phenolic compounds among which hydroxytyrosol and oleuropein stand out [3]. Extracts of these compounds could be used in the food industry as preservatives, due to their antioxidant and antimicrobial properties [4]. Consequently, the extraction of phenolic compounds from olive leaves could be a way to add value to this residue; thus, presenting the oil industry with another option for greater sustainability in their processing activities.
Phenol content and antioxidant capacity of olive leaves depend on cultivar type, olive tree age, cultivation area [5] and sampling time [6,7,8]. Cultivar effect on phenol content and antioxidant capacity has been previously studied [9,10,11] in 'Arbequina' and 'Picual' leaves [12,13,14]. However, there is no scientific data characterizing 'Arroniz' nor 'Empeltre' leaves and only, scarce information is available about 'Arbosana' leaves [5]. Few studies have been published about the interannual variability [15]; none of which contain Spanish cultivars.
Polyphenol oxidases (PPO) are enzymes that cause the degradation of phenol compounds in olive leaves, specifically, oleuropein [16]. To prevent PPO activity, the water content of olive leaves should be decreased. The most studied method for the preservation of phenolic compounds from olive leaves is dehydration. Traditionally, olive leaves have been air-dried, which is the easiest and most economic drying method. However, air-drying is more time-consuming compared to oven and freeze-drying. Even so, several studies [17,18] have suggested air-drying to be effective for the preservation of phenolic compounds in olive leaves.
The effect of oven-drying is unclear; some studies [19,20] have presented polyphenol degradation while other investigations [18,21] concluded that drying olive leaves at high temperatures for a short time does not degrade their polyphenols. Vacuum-drying was studied to prevent the degradation of phenolic compounds; no differences were observed when compared to oven-drying [20,22]. Freeze-drying has proved to be effective in the preservation of olive leaf phenols [18,21]. Despite the quantity of literature that characterizes the phenols in olive leaves dried by the mentioned methods, comparison of them with fresh olive leaf phenol content has not been reported. Therefore, further studies assessing the effect of the drying methods on the phenolic compounds and antioxidant capacity of the olive leaf are required.
To valorize olive leaves by the extraction of their phenolic compounds, all the above-mentioned factors must be kept in mind to produce extracts with homogeneous characteristics. Olive cultivar, interannual variability and drying methods can influence the phenolic content and antioxidant capacity in olive leaves. Thus, the aim of this research was to characterize total antioxidant capacity, total phenol, hydroxytyrosol and oleuropein content in 'Arroniz', 'Empeltre', 'Arbosana', 'Picual', and 'Arbequina' olive leaves and to assess the effect of four drying methods on these parameters.
Fresh olive leaves were collected from the cleaning process of olives in an oil mill in Tudela (Navarre, Spain). Olive leaves (8 kg of each one) were sampled from five different cultivars ('Arroniz', 'Empeltre', 'Arbosana', 'Picual', conventional 'Arbequina' and ecological 'Arbequina') in two consecutive years during the local harvesting season in November. All the five orchards were cultivated under conventional methods using synthetic pesticides and fertilizers. Leaves collected from ecological 'Arbequina' grove were also used. To prevent influence of agricultural and environmental conditions, the leaves were taken from olive trees of the same age (ten years old) and from the same orchard "La Estanca" (42.015827° N, 1.705438° W, 368 m above sea level) located near Cascante (Navarra). Leaves were detached from branches, vacuum (95%) packaged and stored in refrigeration (4 ℃) and darkness until their analysis, which was carried out within the first five days of storage.
Oleuropein analytical standard (≥98%), Trolox (97%), Folin & Ciocalteu's phenol reagent (2 N) and gallic acid monohydrate (≥98%) were purchased from Sigma-Aldrich (St. Louis, Missouri, USA). Hydroxytyrosol analytical standard (≥98%) was acquired from Extrasynthèse (Genay, France) and 2, 2-diphenyl-1-picrylhydrazyl, DPPH (95%), from Alfa Aesar–Thermo Fisher Scientific (Haverhill, Massachusetts, USA). Sodium carbonate anhydrous (100%), acetic acid (100%) and methanol (≥99.9%) were procured from VWR International Eurolab (Barcelona, Spain). Acetonitrile (≥99.9%) was acquired from Merck (Darmstadt, Germany). Type II and type I water were obtained from an Automatic Plus GR (Wasserlab, Barbatain, Spain) purification system.
The effect of four drying methods (vacuum-drying, oven-drying, freeze-drying and air-drying) on phenolic compounds and antioxidant capacity were compared in six olive leaf samples collected in the second year. Vacuum-drying was carried out in a Memmert VO 400 oven (DD Biolab, Barcelona, Spain) at 40 ℃ and 150 mbar of vacuum for four days. Oven-drying was conducted in the same oven, at 105 ℃, for 4.5 h, without vacuum. Olive leaf samples were freeze-dried in a Lyoalfa-6 laboratory freeze-dryer (Azbil Telstar, Barcelona, Spain) under vacuum (50 mbar) at −72 ℃ for three days. Air-drying was performed by spreading leaves onto a closed surface with natural air at 20–25 ℃ without direct sunlight for fourteen days.
Fresh and dried leaves (2 g) were ground with a Grindomix GM 200 (Retsch, Germany) and maintained at 105 ℃ until constant weight using a HR83 Halogen Moisture Analyzer (Mettler Toledo, Barcelona, Spain). The leaf water content was expressed as water loss (%) referred to the initial water content.
Fresh and dried olive leaves were ground in Grindomix GM 200 at 10000 rpm for 2 min and passed through a 1 mm mesh sieve. The ground material (0.50 ± 0.01 g) was mixed with 4 mL of methanol/water 70:30 (v/v) in Genogrinder 2010 (Spex, Spain) at 800 rpm for 15 min, at room temperature (RT). The mixture was centrifuged at 4816 x g for 10 min at 20 ℃ (Sorvall ST40R, Thermo Scientific). The supernatant was removed and saved. The extraction process was repeated from the pellet. Finally, both supernatants were mixed and diluted to a final volume of 10 mL. The olive leaf extract was filtered through a 0.22 μm PVDF Whatman filter and stored for further analysis in refrigeration (4 ℃) and darkness.
Total phenol content (TPC) of the olive leaf extract was analyzed by the Folin-Ciocalteu method as described by Obied et al. (2005) [23] with some modifications. Briefly, a gallic acid (0–750 mg/L) calibration curve (R2 0.9918–0.9999) was prepared from the standard solution of gallic acid in methanol (1 g/L). Each extract was appropriately diluted with methanol/water 70/30 (v/v). The diluted extract (0.2 mL) was mixed with 5 mL of 10% Folin-Ciocalteu reagent and 2.8 mL of deionized water. This mixture was vortexed, stored 5 min at RT, and added with 2 mL of sodium carbonate solution (20% w/v in deionized water). After an incubation time of 1.5 h, absorbance was measured at 750 nm in a V-530 UV/VIS Spectrophotometer (Jasco, Tokyo, Japan). Deionized water was used as the comparative blank. The results were expressed as mg gallic acid equivalents/g dry weight (mg GAE/g DW) of olive leaf. TPC was determined in fresh olive leaves collected in both years and in dried leaf in the second year. TPC interannual variability was calculated as the relative standard deviation (RSD) of the values of the two years.
Total antioxidant capacity (TAC) of the olive leaves extract was assessed using DPPH radical scavenging activity method described by Brand-Williams et al. (1995) [24] with slight modifications. Briefly, to obtain the calibration curve (0–600 µmol/mL), R2 0.9879–0.9990, the standard solution was prepared with 0.03 g of Trolox dissolved in 50 mL of methanol. The DPPH solution was prepared dissolving a DPPH concentrated solution (0.12 g/L) in methanol (approximately 1/5 v/v) until absorbance at 515 nm was adjusted to 0.75 ± 0.05. In a cuvette, 1960 μL of the DPPH adjusted solution was mixed with 40 μL of olive leaf extract, covered with parafilm to prevent solvent evaporation and incubated 1 h at RT. Absorbance was measured at 515 nm against methanol as blank in a V-530 UV/VIS Spectrophotometer. The results were expressed as μmol Trolox equivalents/g leaf DW (μmol TE/g DW). TAC was measured in fresh olive leaves collected in both years and in dried leaves collected in the second year. TAC interannual variability was calculated as the relative standard deviation (RSD) of the values of the two years.
Determinations of hydroxytyrosol (HC) and oleuropein (OC) contents in olive leaf extracts were performed according to the method described by Suárez et al. (2008) [25] with some modifications. The compounds were separated by HPLC with an e2695 Separation module coupled to a 2998 Photodiode Array Detector (PAD) controlled by Empower software (Waters Alliance, USA) together with an Atlantis dC18 (4.6 x 100 mm, 3 µm) reverse-phased column (Waters Alliance, USA).
Injection volume was 20 µL and the flow rate was 0.8 mL/min. The mobile phase was a gradient of 0.2% acetic acid aqueous solution (A) and 50/50 (v/v) methanol/acetonitrile (B) as follows: 0 min, 96% A, 4% B; 0–40 min, 50% A, 50% B; 40–60 min, 0% A, 100% B; 60–65 min, 0% A, 100% B; 65–67 min, 96% A, 4% B; 67–75 min, 96% A, 4% B. Effluent was monitored under an absorbance detector at A280. Chromatographic peaks were identified by comparing the retention time of samples with those of standard (hydroxytyrosol, 10–1000 µg/mL, R2 0.9967–0.9999; oleuropein, 50–2000 µg/mL, R2 0.9868-0.9997). HC and OC were determined in fresh and dried olive leaf samples collected in the second year.
Experimental data of TPC and TAC from fresh olive leaves collected in both years and water content results were analyzed by one-way ANOVA with a confidence level of 95%. The TPC, TAC, HC and OC data from fresh and dried olive leaf samples collected in the second year were analyzed by two-way ANOVA with interaction and a confidence level of 95%. Tukey test was applied as multiple post-hoc comparison to find means that were significantly different (p-value < 0.05) from each other. The results were expressed as mean values ± standard deviation (SD) of the experimental data obtained in three replications, except for water content data, which was analyzed in duplicate. The statistical analysis was performed using the IBM SPSS Statistics 22 software.
Fresh olive leaves contained a water percentage between 41.7 ± 0.4% ('Picual') and 55.8 ± 0.3% ('Arbosana'). Dried olive leaf water content ranged from 1.75 ± 0.03% (freeze-dried 'Arroniz' olive leaves) to 14.95 ± 0.04% (vacuum-dried 'Picual' olive leaves). All the analyzed drying methods were effective since they reached a water loss over 60% (Figure 1), ensuring a water content below 15% in all the dried samples. Oven-drying and freeze-drying were the most effective methods.
Fresh olive leaves contained a TPC from 15.0 ± 0.7 to 37.4 ± 2.0 mg GAE/g DW (Figure 2). Other authors reported similar TPC in olive leaves: 17–25 [26], 35 [21] and 10–49 mg GAE/g DW [9]. Among the fresh olive leaf cultivars evaluated, 'Arbosana' leaves had the highest mean TPC value (34.0 ± 1.1 mg GAE/g DW). 'Arroniz' and 'Empeltre' olive leaves had TPCs (25.2 ± 1.8 and 26.2 ± 0.9 mg GAE/g DW, respectively) similar to 'Picual' (24.8 ± 3.0 mg GAE/g DW), conventional 'Arbequina' (26.2 ± 15.9 mg GAE/g DW), and ecological 'Arbequina' (26.6 ± 3.6 mg GAE/g DW) leaves. Significant differences (p-value < 0.05) in TPC were not observed between conventional and ecological 'Arbequina' olive leaves. In ecological cultivation, as synthetic pesticides cannot be used, plants are exposed to greater stress; this normally induces the production of defense substances, such as phenolic compounds [27]. However, this effect was not clearly observed in the present work.
'Empeltre' (3.4%) and 'Arbosana' (3.2%) fresh leaves had the lowest TPC interannual variability. Conventional 'Arbequina' fresh leaves presented the highest TPC RSD (60.5%). The only study including TPC interannual variability concluded that it was cultivar dependent and significant (p-value < 0.05) for 'Arbequina' olive leaves [15]. These results could be critical in the selection of the most suitable olive leaves to be valorized, as the supply of phenolic compounds will depend on the interannual variability of TPC. Therefore, among fresh olive leaves 'Arbosana' were the most suitable to valorize because they showed the highest mean TPC and one of the lowest TPC interannual variability.
Vacuum-drying significantly decreased TPC in 'Arroniz' (11.5 ± 0.8 mg GAE/g DW), 'Empeltre' (6.0 ± 1.1 mg GAE/g DW) and 'Picual' (10.0 ± 1.2 mg GAE/g DW) olive leaf samples (Table 1). While in 'Arbosana' (35.4 ± 2.0 mg GAE/g DW) and 'Arbequina' leaves, conventional (28.9 ± 0.9 mg GAE/g DW) and ecological (34.6 ± 3.8 mg GAE/g DW) TPC was maintained or significantly higher after vacuum-drying. The diminution of TPC could be attributed to PPO activity. The vacuum-drying treatment took 4 days throughout which olive leaves were exposed to 40 ℃, conditions that could promote the action of PPO. Browning in 'Arroniz', 'Empeltre' and 'Picual' vacuum-dried olive leaves was visible (Figure 3) indicating that enzymatic reactions may have taken place. Therefore, at these conditions, some phenols could have been degraded by the action of enzymes [18,28].
Cultivar | Fresh | Vacuum-dried | Oven-dried | Freeze-dried | Air-dried | p-value |
Total phenol content (mg GAE/g DW) | ||||||
'Arroniz' | 26.5 ± 1.3 Cc | 11.5 ± 0.8 Ba | 17.4 ± 1.0 Bb | 42.2 ± 2.6 CDd | 37.9 ± 3.2 ABd | 0.000 |
'Empeltre' | 26.8 ± 0.2 Cc | 6.0 ± 1.1 Aa | 15.3 ± 0.6 ABb | 25.6 ± 2.1 Bc | 39.8 ± 4.2 ABd | 0.000 |
'Arbosana' | 34.8 ± 0.1 Db | 35.4 ± 2.0 Db | 21.6 ± 0.3 Ca | 44.3 ± 1.2 Dc | 42.6 ± 1.7 Bc | 0.000 |
'Picual' | 22.6 ± 0.6 Bc | 10.0 ± 1.2 ABa | 13.2 ± 0.3 Aab | 16.0 ± 0.7 Ab | 37.8 ± 4.8 ABd | 0.000 |
Conventional 'Arbequina' | 15.0 ± 0.7 Aa | 28.9 ± 0.9 Cb | 33.0 ± 0.7 Db | 34.5 ± 5.4 Cb | 29.2 ± 1.0 Ab | 0.000 |
Ecological 'Arbequina' | 24.1 ± 1.0 Ba | 34.6 ± 3.8 Db | 37.0 ± 2.0 Ebc | 46.1 ± 2.8 Dc | 45.4 ± 6.6 Bc | 0.000 |
p-value | 0.000 | 0.000 | 0.000 | 0.000 | 0.007 | |
Total antioxidant capacity (µmol TE/g DW) | ||||||
'Arroniz' | 113 ± 15 BCb | 44 ± 5 Aa | 209 ± 10 Bc | 204 ± 29 ABCc | 276 ± 42 Bd | 0.000 |
'Empeltre' | 86 ± 17 ABab | 38 ± 5 Aa | 114 ± 37 Ab | 134 ± 27 ABb | 218 ± 34 ABc | 0.000 |
'Arbosana' | 160 ± 18 Cab | 120 ± 35 Bab | 89 ± 27 Aa | 201 ± 49 ABCb | 198 ± 10 ABb | 0.005 |
'Picual' | 109 ± 32 Bbc | 31 ± 2 Aa | 59 ± 6 Aab | 57 ± 6 Aa | 150 ± 27 Ac | 0.000 |
Conventional 'Arbequina' | 57 ± 1 Aa | 137 ± 39 Bab | 190 ± 32 Bab | 283 ± 112 Cb | 161 ± 10 Aab | 0.007 |
Ecological 'Arbequina' | 82 ± 4 ABa | 147 ± 20 Bab | 212 ± 10 Bb | 222 ± 31 BCb | 225 ± 53 ABb | 0.000 |
p-value | 0.000 | 0.000 | 0.000 | 0.004 | 0.006 | |
Hydroxytyrosol content (mg /g DW) | ||||||
'Arroniz' | 0.06 ± 0.01 Aa | 0.14 ± 0.00 Aa | 0.34 ± 0.01 Bb | 0.92 ± 0.06 Cd | 0.65 ± 0.09 Cc | 0.000 |
'Empeltre' | 1.37 ± 0.11 Dd | 0.15 ± 0.00 Aa | 0.36 ± 0.05 BCb | 0.62 ± 0.02 Bc | 0.51 ± 0.05 BCbc | 0.000 |
'Arbosana' | 1.53 ± 0.04 Dc | 0.80 ± 0.08 Cb | 0.44 ± 0.03 Ca | 0.82 ± 0.04 Cb | 0.41 ± 0.08 Ba | 0.000 |
'Picual' | 0.20 ± 0.02 ABab | 0.18 ± 0.00 Aa | 0.23 ± 0.01 Ab | 0.21 ± 0.01 Aab | 0.90 ± 0.02 Dc | 0.000 |
Conventional 'Arbequina' | 0.96 ± 0.02 Cb | 1.01 ± 0.04 Db | 1.31 ± 0.02 Dc | 0.93 ± 0.06 Cb | 0.13 ± 0.02 Aa | 0.000 |
Ecological 'Arbequina' | 0.27 ± 0.09 Ba | 0.45 ± 0.03 Bb | 1.34 ± 0.03 Dd | 1.44 ± 0.03 Dd | 0.96 ± 0.04 Dc | 0.000 |
p-value | 0.000 | 0.000 | 0.000 | 0.000 | 0.000 | |
Oleuropein content (mg/g DW) | ||||||
'Arroniz' | 0.3 ± 0.1 Ba | 1.8 ± 0.1 Aa | 0.6 ± 0.1 ABa | 20.1 ± 1.8 Cb | 36.8 ± 3.1 CDc | 0.000 |
'Empeltre' | < 0.05 Aa | 1.3 ± 0.0 Ab | 0.2 ± 0.0 Aab | 4.0 ± 0.1 Ac | 32.4 ± 1.0 Cd | 0.000 |
'Arbosana' | < 0.05 Aa | 30.4 ± 1.7 Cc | 1.3 ± 0.1 Ba | 20.4 ± 1.0 Cb | 23.5 ± 2.0 Bc | 0.000 |
'Picual' | 0.8 ± 0.1 Ca | 2.5 ± 0.2 Aab | 0.5 ± 0.1 ABa | 3.4 ± 0.0 Ab | 40.1 ± 2.1 Dc | 0.000 |
Conventional 'Arbequina' | < 0.05 Aa | 20.9 ± 0.6 Bc | 23.8 ± 0.5 Dd | 14.6 ± 1.8 Bb | 14.4 ± 1.2 Ab | 0.000 |
Ecological 'Arbequina' | < 0.05 Aa | 30.0 ± 2.2 Cd | 8.3 ± 0.5 Cb | 16.0 ± 0.2 Bc | 35.6 ± 1.5 CDe | 0.000 |
p-value | 0.000 | 0.000 | 0.000 | 0.000 | 0.000 | |
Values are the mean (n = 3) ± standard deviation. Distinct capital letters show significant differences between cultivars and distinct lower-case letters represent significant differences between drying methods (two-way ANOVA; Tukey test: p-value < 0.05). GAE: gallic acid equivalents; TE: Trolox equivalents; DW: dry weigh; SD: standard deviation. |
Oven-drying decreased TPC in all samples except in 'Arbequina' (Table 1). The exposure to high temperatures during oven-drying could have caused browning (Figure 3) and the degradation of phenolic compounds [29]. However, some authors concluded that applying high temperatures for a short time, aiming at dehydrating the olive leaves, does not always decrease their phenol content [18,21]; as shown by the TPCs of oven-dried conventional and ecological 'Arbequina' samples evaluated in the present research.
TPC of the freeze-dried leaves were similar or significantly higher (p-value < 0.05) compared to their fresh leaf counterparts, except for 'Picual' olive leaves, which had significantly lower (p-value < 0.05) TPC (Table 1). Freeze-dried olive leaves did not show any browning (Figure 3), which indicated that PPO was not active as olive leaves were not exposed to temperatures above 10 ℃ [18]. In this research, freeze-drying proved to preserve phenolic compounds confirming the conclusion of other researchers [18,21].
The TPC in the air-dried leaf samples was significantly greater (p-value < 0.05) than that in the fresh leaves (Table 1). The moderate conditions used during this method prevented the degradation of phenolic compounds and maintained the green color of the olive leaves better (Figure 3). Afaneh et al. (2015) [17] and Kamran et al. (2015) [18] also concluded that air-drying was an effective method for the preservation of phenolic compounds. Among air-dried olive leaves (Table 1), ecological 'Arbequina' had the highest TPC (conventional 'Arbequina' ≤ 'Picual' = 'Arroniz' = Empeltre ≤ 'Arbosana = ecological 'Arbequina with 29.2 ± 1.0, 37.8 ± 4.8, 37.9 ± 3.2, 39.8 ± 4.2, 42.6 ± 1.7, and 45.4 ± 6.6 mg GAE/g DW, respectively).
TAC of fresh olive leaves was between 55.3 ± 0.1 and 160 ± 18 µmol TE/g DW (Figure 4). These results agree with the values (60 µmol TE/g DW) observed by Ahmad-Qasem et al. (2016) [21]. 'Arbosana' olive leaves presented one of the highest mean TAC values (146 ± 20 µmol TE/g DW) together with 'Picual' (113 ± 5 µmol TE/g DW) and conventional 'Arbequina' (104 ± 69 µmol TE/g DW). The TAC of 'Arroniz' (101 ± 18 µmol TE/g DW) and 'Empeltre' (80 ± 8 µmol TE/g DW) olive leaves were not significantly different (p-value > 0.05) from the TAC of 'Picual' (113 ± 5 µmol TE/g DW), conventional 'Arbequina' (104 ± 69 µmol TE/g DW) nor ecological 'Arbequina' (85 ± 4 µmol TE/g DW) olive leaves. Significant differences (p-value < 0.05) in TAC were not reported between conventional and ecological 'Arbequina' olive leaves. Thus, the effect of increasing phenolic compounds production to enhance the natural defense system observed by other authors in ecological cultivars [27] was not observed in the TAC values reported in this research.
'Picual' and ecological 'Arbequina' fresh leaves presented the lowest RSD of TAC (4.4% and 4.7%, respectively). The TAC interannual variability in 'Empeltre' (9.3%) and 'Arbosana' (13.4%) were also low. Conventional 'Arbequina' fresh leaves showed the highest RSD of TAC (66.5%). Therefore, among fresh olive leaves, 'Arbosana' leaves were the most suitable to valorize due to their high TAC and low interannual variability.
Vacuum-drying decreased TAC in olive leaves, except for both conventional and ecological 'Arbequina' samples (Table 1). Differences were only significant (p-value < 0.05) for 'Arroniz' and 'Picual' olive leaves. The reduction of TAC could have been caused by PPO that could be active at vacuum-drying conditions (40 ℃ for 4 days). In Figure 3 the visible browning in 'Arroniz', 'Empeltre' and 'Picual' vacuum-dried olive leaves may indicate that the effect of PPO activity degraded the phenolic compound and their antioxidant capacity [18,28].
After oven-drying, TAC did not significantly decrease (p-value > 0.05) in any of the studied olive leaf samples. Other authors also concluded that dehydrating olive leaves by applying high temperatures for a short time did not always decrease their TAC [18,21]. TAC of the freeze-dried leaves analyzed in this work were similar or significantly higher (p-value < 0.05) compared to their fresh leaf sample counterparts, except for 'Picual' olive leaves. In 'Picual' the TAC was significantly lower (p-value < 0.05). In this research, freeze-drying proved to have the ability to preserve TAC, confirming the conclusion of other researchers [18,21].
Air-drying maintained or significantly increased (p-value < 0.05) TAC of all the olive leaf samples. As observed by other authors [17,18], the moderate conditions used during this method prevented the degradation of TAC and maintained the original color of the leaves (Figure 3). Among air-dried leaves the highest TAC ('Picual' = conventional 'Arbequina' ≤ 'Arbosana' = 'Empeltre' = ecological 'Arbequina' ≤ 'Arroniz' with 150 ± 27,161 ± 10,198 ± 10,218 ± 34,225 ± 53, and 276 ± 42 µmol TE/g DW, respectively) was observed in 'Arroniz' and ecological 'Arbequina' leaves.
In 'Empeltre' and 'Arbosana' leaves, the samples with the highest HC in fresh samples (1.37 ± 0.11 and 1.53 ± 0.04 mg hydroxytyrosol/g DW, respectively), HC was significantly lower after vacuum-drying (0.15 ± 0.00 and 0.80 ± 0.08 mg hydroxytyrosol/g DW, respectively), oven-drying (0.36 ± 0.05 and 0.44 ± 0.03 mg hydroxytyrosol/g DW, respectively), freeze-drying (0.62 ± 0.02 and 0.82 ± 0.04 mg hydroxytyrosol/g DW, respectively) and air-drying (0.51 ± 0.05 and 0.41 ± 0.08 mg hydroxytyrosol/g DW, respectively). In 'Arroniz', 'Picual', conventional and ecological 'Arbequina' olive leaves HC was maintained or higher after the different drying methods, except for conventional 'Arbequina' leaves after air-drying (0.13 ± 0.02 mg hydroxytyrosol/g DW). When the investigated drying methods were applied, OC of the olive leaf samples was stable, or significantly higher (Table 1).
Among air-dried leaves, ecological 'Arbequina' presented the highest HC (conventional 'Arbequina' < 'Arbosana' ≤ 'Empeltre' ≤ 'Arroniz' < 'Picual' = ecological 'Arbequina' with 0.13 ± 0.02, 0.41 ± 0.08, 0.51 ± 0.05, 0.65 ± 0.09, 0.90 ± 0.02, and 0.96 ± 0.04 mg hydroxytyrosol/g DW, respectively). Whereas, 'Picual', 'Arroniz' and ecological 'Arbequina' leaf samples contained the highest OC (conventional 'Arbequina' < 'Arbosana' < 'Empeltre' ≤ ecological 'Arbequina' = 'Arroniz' ≤ 'Picual with 14.4 ± 1.2, 23.5 ± 2.0, 32.4 ± 1.0, 35.6 ± 1.5, 36.8 ± 3.1, and 40.1 ± 2.1 mg oleuropein/g DW, respectively).
In general, vacuum-drying and oven-drying could be effective drying methods for some olive leaf cultivars as long as the olive leaves are not exposed to high temperatures for long periods of time [18,21]. Freeze-drying proved to preserve phenolic compounds in leaves, confirming the conclusion of other researchers [18,21]. The moderate conditions used during air-drying prevented the degradation of phenolic compounds, as reported by other authors [17,18].
In the present study, the TPC, TAC, HC and OC of several olive leaf samples were significantly higher (p-value < 0.05) after drying methods. This could have been caused by the damage on plant tissues caused by drying. This damage enhances the transference of matter allowing better penetration of the solvent and therefore a higher concentration of phenolic compounds in the extracts [17,28].
This research characterizes 'Arroniz' and 'Empeltre' olive leaves for the first time and compares them with olive leaves from other cultivars like 'Arbosana', 'Picual' and 'Arbequina'. Among fresh olive leaf samples, 'Arbosana' stood out for its high TPC, TAC and HC and low TPC and TAC interannual variabilities. With the data illustrating the effect of drying methods on the TPC, TAC, HC and OC, the most suitable drying method for each olive leaf cultivar can be easily selected. For almost all the studied olive leaves, with exception of conventional 'Arbequina', air-dried olive leaves had higher TPC, TAC and OC than vacuum-dried, oven-dried or freeze-dried leaves. Thus, air-drying was selected as the most suitable drying method. Among air-dried leaves, ecological 'Arbequina' were selected as the most suitable to valorize because they stood out in all the studied parameters (TPC, TAC, HC and OC).
This work was supported by the Government of Navarra (grant numbers 0011-1365-2017-000227, 0011-1408-2017-000023, 0011-1411-2018-000032).
All authors declare no conflicts of interest in this paper.
[1] | International Olive Council (2019) International Olive Council Newsletter, The international market, 2019. Available from: https://www.internationaloliveoil.org/wp-content/uploads/2019/12/newsletter_144_english.pdf. |
[2] |
Molina-Alcaide E, Yáñez-Ruiz DR (2008) Potential use of olive by-products in ruminant feeding: A review. Anim Feed Sci Technol 147: 247–264. https://doi.org/10.1016/j.anifeedsci.2007.09.021 doi: 10.1016/j.anifeedsci.2007.09.021
![]() |
[3] |
Žugčić T, Abdelkebir R, Alcantara C, et al. (2019) From extraction of valuable compounds to health promoting benefits of olive leaves through bioaccessibility, bioavailability and impact on gut microbiota. Trends Food Sci Technol 83: 63–77. https://doi.org/10.1016/j.tifs.2018.11.005 doi: 10.1016/j.tifs.2018.11.005
![]() |
[4] |
Nunes MA, Pimentel FB, Costa ASG, et al. (2016) Olive by-products for functional and food applications: Challenging opportunities to face environmental constraints. Innov Food Sci Emerg Technol 35: 139–148. https://doi.org/10.1016/j.ifset.2016.04.016 doi: 10.1016/j.ifset.2016.04.016
![]() |
[5] |
Talhaoui N, Taamalli A, Gómez-Caravaca AM, et al. (2015) Phenolic compounds in olive leaves: Analytical determination, biotic and abiotic influence, and health benefits. Food Res Int 77: 92–108. https://doi.org/10.1016/j.foodres.2015.09.011 doi: 10.1016/j.foodres.2015.09.011
![]() |
[6] |
Talhaoui N, Gómez-Caravaca AM, Roldán C, et al. (2015) Chemometric analysis for the evaluation of phenolic patterns in olive leaves from six cultivars at different growth stages. J Agric Food Chem 63: 1722–1729. https://doi.org/10.1021/jf5058205 doi: 10.1021/jf5058205
![]() |
[7] | Şahin S, Ahmed Malik NS, Perez JL, et al. (2012) Seasonal changes of individual phenolic compounds in leaves of twenty olive cultivars grown in Texas. J Agric Sci Technol 2: 242–247. |
[8] |
Özcan MM, Fındık S, AlJuhaimi F, et al. (2019) The effect of harvest time and varieties on total phenolics, antioxidant activity and phenolic compounds of olive fruit and leaves. J Food Sci Technol 56: 2373–2385. https://doi.org/10.1007/s13197-019-03650-8 doi: 10.1007/s13197-019-03650-8
![]() |
[9] |
Nicolì F, Negro C, Vergine M, et al. (2019) Evaluation of phytochemical and antioxidant properties of 15 Italian Olea europaea L. cultivar leaves. Molecules 24: 1998. https://doi.org/10.3390/molecules24101998 doi: 10.3390/molecules24101998
![]() |
[10] |
Hülya-Orak H, Karamać M, Amarowicz R, et al. (2019) Genotype-related differences in the phenolic compound profile and antioxidant activity of extracts from olive (Olea europaea L.) leaves. Molecules 24: 1130. https://doi.org/10.3390/molecules24061130 doi: 10.3390/molecules24061130
![]() |
[11] |
Olmo-García L, Bajoub A, Benlamaalam S, et al. (2018) Establishing the phenolic composition of Olea europaea L. leaves from cultivars grown in Morocco as a crucial step towards their subsequent exploitation. Molecules 23: 2524. https://doi.org/10.3390/molecules23102524 doi: 10.3390/molecules23102524
![]() |
[12] |
Guinda Á, Castellano JM, Santos-Lozano JM, et al. (2015) Determination of major bioactive compounds from olive leaf. LWT-Food Sci Technol 64: 431–438. https://doi.org/10.1016/j.lwt.2015.05.001 doi: 10.1016/j.lwt.2015.05.001
![]() |
[13] |
Romero C, Medina E, Mateo MA, et al. (2017) Quantification of bioactive compounds in Picual and Arbequina olive leaves and fruit. J Sci Food Agric 97: 1725–1732. https://doi.org/10.1002/jsfa.7920 doi: 10.1002/jsfa.7920
![]() |
[14] |
Lama-Muñoz A, Contreras MM, Espínola F, et al. (2020) Content of phenolic compounds and mannitol in olive leaves extracts from six Spanish cultivars: Extraction with the Soxhlet method and pressurized liquids. Food Chem 320: 126626. https://doi.org/10.1016/j.foodchem.2020.126626 doi: 10.1016/j.foodchem.2020.126626
![]() |
[15] |
Papoti VT, Papageorgiou M, Dervisi K, et al. (2018) Screening olive leaves from unexploited traditional Greek cultivars for their phenolic antioxidant dynamic. Foods 7: 197. https://doi.org/10.3390/foods7120197 doi: 10.3390/foods7120197
![]() |
[16] |
De Leonardis A, Macciola V, Cuomo F, et al. (2015) Evidence of oleuropein degradation by olive leaf protein extract. Food Chem 175: 568–574. https://doi.org/10.1016/j.foodchem.2014.12.016 doi: 10.1016/j.foodchem.2014.12.016
![]() |
[17] |
Afaneh I, Yateem H, Al-Rimawi F (2015) Effect of olive leaves drying on the content of oleuropein. Am J Anal Chem 6: 246–252. http://dx.doi.org/10.4236/ajac.2015.63023 doi: 10.4236/ajac.2015.63023
![]() |
[18] |
Kamran M, Hamlin AS, Scott CJ, et al. (2015) Drying at high temperature for a short time maximizes the recovery of olive leaf biophenols. Ind Crop Prod 78: 29–38. https://doi.org/10.1016/j.indcrop.2015.10.031 doi: 10.1016/j.indcrop.2015.10.031
![]() |
[19] |
Attya M, Benabdelkamel H, Perri E, et al. (2010) Effects of conventional heating on the stability of major olive oil phenolic compounds by tandem mass spectrometry and isotope dilution assay. Molecules 15: 8734. https://doi.org/10.3390/molecules15128734 doi: 10.3390/molecules15128734
![]() |
[20] |
Şahin S, Elhussein E, Bilgin M, et al. (2018) Effect of drying method on oleuropein, total phenolic content, flavonoid content, and antioxidant activity of olive (Olea europaea) leaf. J Food Process Preserv 42: e13604. https://doi.org/10.1111/jfpp.13604 doi: 10.1111/jfpp.13604
![]() |
[21] |
Ahmad-Qasem MH, Ahmad-Qasem BH, Barrajón-Catalán E, et al. (2016) Drying and storage of olive leaf extracts. Influence on polyphenols stability. Ind Crop Prod 79: 232–239. http://dx.doi.org/10.1016/j.indcrop.2015.11.006 doi: 10.1016/j.indcrop.2015.11.006
![]() |
[22] |
Ali Elhussein EA, Şahin S (2018) Drying behaviour, effective diffusivity and energy of activation of olive leaves dried by microwave, vacuum and oven drying methods. Heat Mass Transf 54: 1901–1911. https://doi.org/10.1007/s00231-018-2278-6 doi: 10.1007/s00231-018-2278-6
![]() |
[23] |
Obied HK, Allen MS, Bedgood DR, et al. (2005) Investigation of Australian olive mill waste for recovery of biophenols. J Agric Food Chem 53: 9911–9920. https://doi.org/10.1021/jf0518352 doi: 10.1021/jf0518352
![]() |
[24] |
Brand-Williams W, Cuvelier M., Berset C (1995) Use of a free radical method to evaluate antioxidant activity. Food Sci Technol 28: 25–30. https://doi.org/10.1016/S0023-6438(95)80008-5 doi: 10.1016/S0023-6438(95)80008-5
![]() |
[25] |
Suárez M, Macià A, Romero MP, et al. (2008) Improved liquid chromatography tandem mass spectrometry method for the determination of phenolic compounds in virgin olive oil. J Chromatogr A 1214: 90–99. https://doi.org/10.1016/j.chroma.2008.10.098 doi: 10.1016/j.chroma.2008.10.098
![]() |
[26] |
Abaza L, Ben-Youssef N, Manai H, et al. (2011) Chétoui olive leaf extracts: influence of the solvent type on phenolics and antioxidant activities. Grasas y aceites 62: 96–104. https://doi.org/10.3989/gya.044710 doi: 10.3989/gya.044710
![]() |
[27] |
López-Yerena A, Lozano-Castellón J, Olmo-Cunillera A, et al. (2019) Effects of organic and conventional growing systems on the phenolic profile of extra-virgin olive oil. Molecules 24: 1986. https://doi.org/10.3390/molecules24101986 doi: 10.3390/molecules24101986
![]() |
[28] |
Taamalli A, Lozano-Sánchez J, Jebabli H, et al. (2019) Monitoring the bioactive compounds status in Olea europaea according to collecting period and drying conditions. Energies 12: 947. https://doi.org/10.3390/en12050947 doi: 10.3390/en12050947
![]() |
[29] |
Babu AK, Kumaresan G, Aroul-Raj VA, et al. (2018) Review of leaf drying: Mechanism and influencing parameters, drying methods, nutrient preservation, and mathematical models. Renew Sustain Energy Rev 90: 536–556. https://doi.org/10.1016/j.rser.2018.04.002 doi: 10.1016/j.rser.2018.04.002
![]() |
1. | Ioanna Pyrka, Fani Th. Mantzouridou, Nikolaos Nenadis, Optimization of olive leaves' thin layer, intermittent near-infrared-drying, 2023, 84, 14668564, 103264, 10.1016/j.ifset.2022.103264 | |
2. | Dalel Mechi, Bechir Baccouri, Daniel Martín-Vertedor, Leila Abaza, Bioavailability of Phenolic Compounds in Californian-Style Table Olives with Tunisian Aqueous Olive Leaf Extracts, 2023, 28, 1420-3049, 707, 10.3390/molecules28020707 | |
3. | Eva María Ramírez, Manuel Brenes, Concepción Romero, Eduardo Medina, Olive Leaf Processing for Infusion Purposes, 2023, 12, 2304-8158, 591, 10.3390/foods12030591 | |
4. | Salah Chaji, Walid Zenasni, Valerie Tomao, Anne-Sylvie Fabiano-Tixier, El Amine Ajal, Hafida Hanine, Aadil Bajoub, Exploring the potential of advanced eco-friendly extraction techniques for a rapid recovery of oleuropein-rich extracts from “Picholine Marocaine” olive tree leaves, 2023, 36, 23525541, 101248, 10.1016/j.scp.2023.101248 | |
5. | Gulshan Kumar, Jayasree Joshi T, Pavuluri Srinivasa Rao, Padmavati Manchikanti, Effect of thin layer drying conditions on the retention of bioactive components in Malabar spinach (Basella alba) leaves, 2023, 3, 2772753X, 100419, 10.1016/j.focha.2023.100419 |
Cultivar | Fresh | Vacuum-dried | Oven-dried | Freeze-dried | Air-dried | p-value |
Total phenol content (mg GAE/g DW) | ||||||
'Arroniz' | 26.5 ± 1.3 Cc | 11.5 ± 0.8 Ba | 17.4 ± 1.0 Bb | 42.2 ± 2.6 CDd | 37.9 ± 3.2 ABd | 0.000 |
'Empeltre' | 26.8 ± 0.2 Cc | 6.0 ± 1.1 Aa | 15.3 ± 0.6 ABb | 25.6 ± 2.1 Bc | 39.8 ± 4.2 ABd | 0.000 |
'Arbosana' | 34.8 ± 0.1 Db | 35.4 ± 2.0 Db | 21.6 ± 0.3 Ca | 44.3 ± 1.2 Dc | 42.6 ± 1.7 Bc | 0.000 |
'Picual' | 22.6 ± 0.6 Bc | 10.0 ± 1.2 ABa | 13.2 ± 0.3 Aab | 16.0 ± 0.7 Ab | 37.8 ± 4.8 ABd | 0.000 |
Conventional 'Arbequina' | 15.0 ± 0.7 Aa | 28.9 ± 0.9 Cb | 33.0 ± 0.7 Db | 34.5 ± 5.4 Cb | 29.2 ± 1.0 Ab | 0.000 |
Ecological 'Arbequina' | 24.1 ± 1.0 Ba | 34.6 ± 3.8 Db | 37.0 ± 2.0 Ebc | 46.1 ± 2.8 Dc | 45.4 ± 6.6 Bc | 0.000 |
p-value | 0.000 | 0.000 | 0.000 | 0.000 | 0.007 | |
Total antioxidant capacity (µmol TE/g DW) | ||||||
'Arroniz' | 113 ± 15 BCb | 44 ± 5 Aa | 209 ± 10 Bc | 204 ± 29 ABCc | 276 ± 42 Bd | 0.000 |
'Empeltre' | 86 ± 17 ABab | 38 ± 5 Aa | 114 ± 37 Ab | 134 ± 27 ABb | 218 ± 34 ABc | 0.000 |
'Arbosana' | 160 ± 18 Cab | 120 ± 35 Bab | 89 ± 27 Aa | 201 ± 49 ABCb | 198 ± 10 ABb | 0.005 |
'Picual' | 109 ± 32 Bbc | 31 ± 2 Aa | 59 ± 6 Aab | 57 ± 6 Aa | 150 ± 27 Ac | 0.000 |
Conventional 'Arbequina' | 57 ± 1 Aa | 137 ± 39 Bab | 190 ± 32 Bab | 283 ± 112 Cb | 161 ± 10 Aab | 0.007 |
Ecological 'Arbequina' | 82 ± 4 ABa | 147 ± 20 Bab | 212 ± 10 Bb | 222 ± 31 BCb | 225 ± 53 ABb | 0.000 |
p-value | 0.000 | 0.000 | 0.000 | 0.004 | 0.006 | |
Hydroxytyrosol content (mg /g DW) | ||||||
'Arroniz' | 0.06 ± 0.01 Aa | 0.14 ± 0.00 Aa | 0.34 ± 0.01 Bb | 0.92 ± 0.06 Cd | 0.65 ± 0.09 Cc | 0.000 |
'Empeltre' | 1.37 ± 0.11 Dd | 0.15 ± 0.00 Aa | 0.36 ± 0.05 BCb | 0.62 ± 0.02 Bc | 0.51 ± 0.05 BCbc | 0.000 |
'Arbosana' | 1.53 ± 0.04 Dc | 0.80 ± 0.08 Cb | 0.44 ± 0.03 Ca | 0.82 ± 0.04 Cb | 0.41 ± 0.08 Ba | 0.000 |
'Picual' | 0.20 ± 0.02 ABab | 0.18 ± 0.00 Aa | 0.23 ± 0.01 Ab | 0.21 ± 0.01 Aab | 0.90 ± 0.02 Dc | 0.000 |
Conventional 'Arbequina' | 0.96 ± 0.02 Cb | 1.01 ± 0.04 Db | 1.31 ± 0.02 Dc | 0.93 ± 0.06 Cb | 0.13 ± 0.02 Aa | 0.000 |
Ecological 'Arbequina' | 0.27 ± 0.09 Ba | 0.45 ± 0.03 Bb | 1.34 ± 0.03 Dd | 1.44 ± 0.03 Dd | 0.96 ± 0.04 Dc | 0.000 |
p-value | 0.000 | 0.000 | 0.000 | 0.000 | 0.000 | |
Oleuropein content (mg/g DW) | ||||||
'Arroniz' | 0.3 ± 0.1 Ba | 1.8 ± 0.1 Aa | 0.6 ± 0.1 ABa | 20.1 ± 1.8 Cb | 36.8 ± 3.1 CDc | 0.000 |
'Empeltre' | < 0.05 Aa | 1.3 ± 0.0 Ab | 0.2 ± 0.0 Aab | 4.0 ± 0.1 Ac | 32.4 ± 1.0 Cd | 0.000 |
'Arbosana' | < 0.05 Aa | 30.4 ± 1.7 Cc | 1.3 ± 0.1 Ba | 20.4 ± 1.0 Cb | 23.5 ± 2.0 Bc | 0.000 |
'Picual' | 0.8 ± 0.1 Ca | 2.5 ± 0.2 Aab | 0.5 ± 0.1 ABa | 3.4 ± 0.0 Ab | 40.1 ± 2.1 Dc | 0.000 |
Conventional 'Arbequina' | < 0.05 Aa | 20.9 ± 0.6 Bc | 23.8 ± 0.5 Dd | 14.6 ± 1.8 Bb | 14.4 ± 1.2 Ab | 0.000 |
Ecological 'Arbequina' | < 0.05 Aa | 30.0 ± 2.2 Cd | 8.3 ± 0.5 Cb | 16.0 ± 0.2 Bc | 35.6 ± 1.5 CDe | 0.000 |
p-value | 0.000 | 0.000 | 0.000 | 0.000 | 0.000 | |
Values are the mean (n = 3) ± standard deviation. Distinct capital letters show significant differences between cultivars and distinct lower-case letters represent significant differences between drying methods (two-way ANOVA; Tukey test: p-value < 0.05). GAE: gallic acid equivalents; TE: Trolox equivalents; DW: dry weigh; SD: standard deviation. |
Cultivar | Fresh | Vacuum-dried | Oven-dried | Freeze-dried | Air-dried | p-value |
Total phenol content (mg GAE/g DW) | ||||||
'Arroniz' | 26.5 ± 1.3 Cc | 11.5 ± 0.8 Ba | 17.4 ± 1.0 Bb | 42.2 ± 2.6 CDd | 37.9 ± 3.2 ABd | 0.000 |
'Empeltre' | 26.8 ± 0.2 Cc | 6.0 ± 1.1 Aa | 15.3 ± 0.6 ABb | 25.6 ± 2.1 Bc | 39.8 ± 4.2 ABd | 0.000 |
'Arbosana' | 34.8 ± 0.1 Db | 35.4 ± 2.0 Db | 21.6 ± 0.3 Ca | 44.3 ± 1.2 Dc | 42.6 ± 1.7 Bc | 0.000 |
'Picual' | 22.6 ± 0.6 Bc | 10.0 ± 1.2 ABa | 13.2 ± 0.3 Aab | 16.0 ± 0.7 Ab | 37.8 ± 4.8 ABd | 0.000 |
Conventional 'Arbequina' | 15.0 ± 0.7 Aa | 28.9 ± 0.9 Cb | 33.0 ± 0.7 Db | 34.5 ± 5.4 Cb | 29.2 ± 1.0 Ab | 0.000 |
Ecological 'Arbequina' | 24.1 ± 1.0 Ba | 34.6 ± 3.8 Db | 37.0 ± 2.0 Ebc | 46.1 ± 2.8 Dc | 45.4 ± 6.6 Bc | 0.000 |
p-value | 0.000 | 0.000 | 0.000 | 0.000 | 0.007 | |
Total antioxidant capacity (µmol TE/g DW) | ||||||
'Arroniz' | 113 ± 15 BCb | 44 ± 5 Aa | 209 ± 10 Bc | 204 ± 29 ABCc | 276 ± 42 Bd | 0.000 |
'Empeltre' | 86 ± 17 ABab | 38 ± 5 Aa | 114 ± 37 Ab | 134 ± 27 ABb | 218 ± 34 ABc | 0.000 |
'Arbosana' | 160 ± 18 Cab | 120 ± 35 Bab | 89 ± 27 Aa | 201 ± 49 ABCb | 198 ± 10 ABb | 0.005 |
'Picual' | 109 ± 32 Bbc | 31 ± 2 Aa | 59 ± 6 Aab | 57 ± 6 Aa | 150 ± 27 Ac | 0.000 |
Conventional 'Arbequina' | 57 ± 1 Aa | 137 ± 39 Bab | 190 ± 32 Bab | 283 ± 112 Cb | 161 ± 10 Aab | 0.007 |
Ecological 'Arbequina' | 82 ± 4 ABa | 147 ± 20 Bab | 212 ± 10 Bb | 222 ± 31 BCb | 225 ± 53 ABb | 0.000 |
p-value | 0.000 | 0.000 | 0.000 | 0.004 | 0.006 | |
Hydroxytyrosol content (mg /g DW) | ||||||
'Arroniz' | 0.06 ± 0.01 Aa | 0.14 ± 0.00 Aa | 0.34 ± 0.01 Bb | 0.92 ± 0.06 Cd | 0.65 ± 0.09 Cc | 0.000 |
'Empeltre' | 1.37 ± 0.11 Dd | 0.15 ± 0.00 Aa | 0.36 ± 0.05 BCb | 0.62 ± 0.02 Bc | 0.51 ± 0.05 BCbc | 0.000 |
'Arbosana' | 1.53 ± 0.04 Dc | 0.80 ± 0.08 Cb | 0.44 ± 0.03 Ca | 0.82 ± 0.04 Cb | 0.41 ± 0.08 Ba | 0.000 |
'Picual' | 0.20 ± 0.02 ABab | 0.18 ± 0.00 Aa | 0.23 ± 0.01 Ab | 0.21 ± 0.01 Aab | 0.90 ± 0.02 Dc | 0.000 |
Conventional 'Arbequina' | 0.96 ± 0.02 Cb | 1.01 ± 0.04 Db | 1.31 ± 0.02 Dc | 0.93 ± 0.06 Cb | 0.13 ± 0.02 Aa | 0.000 |
Ecological 'Arbequina' | 0.27 ± 0.09 Ba | 0.45 ± 0.03 Bb | 1.34 ± 0.03 Dd | 1.44 ± 0.03 Dd | 0.96 ± 0.04 Dc | 0.000 |
p-value | 0.000 | 0.000 | 0.000 | 0.000 | 0.000 | |
Oleuropein content (mg/g DW) | ||||||
'Arroniz' | 0.3 ± 0.1 Ba | 1.8 ± 0.1 Aa | 0.6 ± 0.1 ABa | 20.1 ± 1.8 Cb | 36.8 ± 3.1 CDc | 0.000 |
'Empeltre' | < 0.05 Aa | 1.3 ± 0.0 Ab | 0.2 ± 0.0 Aab | 4.0 ± 0.1 Ac | 32.4 ± 1.0 Cd | 0.000 |
'Arbosana' | < 0.05 Aa | 30.4 ± 1.7 Cc | 1.3 ± 0.1 Ba | 20.4 ± 1.0 Cb | 23.5 ± 2.0 Bc | 0.000 |
'Picual' | 0.8 ± 0.1 Ca | 2.5 ± 0.2 Aab | 0.5 ± 0.1 ABa | 3.4 ± 0.0 Ab | 40.1 ± 2.1 Dc | 0.000 |
Conventional 'Arbequina' | < 0.05 Aa | 20.9 ± 0.6 Bc | 23.8 ± 0.5 Dd | 14.6 ± 1.8 Bb | 14.4 ± 1.2 Ab | 0.000 |
Ecological 'Arbequina' | < 0.05 Aa | 30.0 ± 2.2 Cd | 8.3 ± 0.5 Cb | 16.0 ± 0.2 Bc | 35.6 ± 1.5 CDe | 0.000 |
p-value | 0.000 | 0.000 | 0.000 | 0.000 | 0.000 | |
Values are the mean (n = 3) ± standard deviation. Distinct capital letters show significant differences between cultivars and distinct lower-case letters represent significant differences between drying methods (two-way ANOVA; Tukey test: p-value < 0.05). GAE: gallic acid equivalents; TE: Trolox equivalents; DW: dry weigh; SD: standard deviation. |