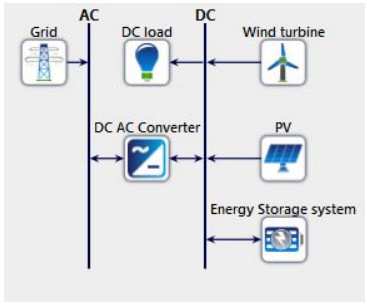
Citation: Hannah P Priyanka, Rahul S Nair. Neuroimmunomodulation by estrogen in health and disease[J]. AIMS Neuroscience, 2020, 7(4): 401-417. doi: 10.3934/Neuroscience.2020025
[1] | He Wang, Yu Liu . Laguerre BV spaces, Laguerre perimeter and their applications. Communications in Analysis and Mechanics, 2023, 15(2): 189-213. doi: 10.3934/cam.2023011 |
[2] | Ho-Sik Lee, Youchan Kim . Boundary Riesz potential estimates for parabolic equations with measurable nonlinearities. Communications in Analysis and Mechanics, 2025, 17(1): 61-99. doi: 10.3934/cam.2025004 |
[3] | Efstratios Stratoglou, Alexandre Anahory Simoes, Leonardo J. Colombo . Reduction in optimal control with broken symmetry for collision and obstacle avoidance of multi-agent system on Lie groups. Communications in Analysis and Mechanics, 2023, 15(2): 1-23. doi: 10.3934/cam.2023001 |
[4] | Heng Yang, Jiang Zhou . Some estimates of multilinear operators on tent spaces. Communications in Analysis and Mechanics, 2024, 16(4): 700-716. doi: 10.3934/cam.2024031 |
[5] | Maomao Wu, Haidong Liu . Multiple solutions for quasi-linear elliptic equations with Berestycki-Lions type nonlinearity. Communications in Analysis and Mechanics, 2024, 16(2): 334-344. doi: 10.3934/cam.2024016 |
[6] | Xiulan Wu, Yaxin Zhao, Xiaoxin Yang . On a singular parabolic $ p $-Laplacian equation with logarithmic nonlinearity. Communications in Analysis and Mechanics, 2024, 16(3): 528-553. doi: 10.3934/cam.2024025 |
[7] | Xiao Qing Huang, Jia Feng Liao . Existence and asymptotic behavior for ground state sign-changing solutions of fractional Schrödinger-Poisson system with steep potential well. Communications in Analysis and Mechanics, 2024, 16(2): 307-333. doi: 10.3934/cam.2024015 |
[8] | Farrukh Dekhkonov . On a boundary control problem for a pseudo-parabolic equation. Communications in Analysis and Mechanics, 2023, 15(2): 289-299. doi: 10.3934/cam.2023015 |
[9] | Lovelesh Sharma . Brezis Nirenberg type results for local non-local problems under mixed boundary conditions. Communications in Analysis and Mechanics, 2024, 16(4): 872-895. doi: 10.3934/cam.2024038 |
[10] | Hongxia Lin, Sabana, Qing Sun, Ruiqi You, Xiaochuan Guo . The stability and decay of 2D incompressible Boussinesq equation with partial vertical dissipation. Communications in Analysis and Mechanics, 2025, 17(1): 100-127. doi: 10.3934/cam.2025005 |
The concept of microgrid was proposed several years ago due to the integration of DERs [1]. In recent years, the DC microgrid has become more attractive compared to the AC microgrid for two reasons. Firstly, most residential and office loads are based on electronic DC loads such as televisions, computers, printers, laptops, phones, tablets, and LED lighting. Secondly, DERs such as photovoltaics, batteries, and small wind power have DC coupling. Therefore, using DC-DC converters in DC microgrids is a more efficient technique for connecting the DERs to the load directly instead of indirectly integrating the DERs to the load through an AC microgrid that utilizes AC-DC and DC-AC transformations [2,3,4,5]. The typical architecture of a DC microgrid is shown in Figure 1 [6]. Because the DERs are decentralized and connected to the point of common coupling in DC microgrids, several control issues of interfacing parallel-connected DC-DC converters with maximum power point tracking, voltage control, and load current sharing are encountered. Although utilizing parallel-connected converters in DC microgrids has several advantages such as power expandability, system reliability, efficiency, and ease of maintenance, the voltage regulation at the point of common coupling and load current sharing present challenging control issues [7,8].
Several control methods have been presented in the literature on current sharing between parallel-connected converters in a DC microgrid [9,10,11,12,13,14,15,16,17,18,19,20]. These methods can be classified into three types based on the circuit theoretic viewpoint. The first type is based on the structure of parallel-connected converters in which all parallel converters are represented by their Thevenin sources with their control based on the droop method.
In the second type, all parallel-connected converters are represented by one Thevenin source connected in parallel with Norton sources. The Thevenin source regulates the output voltage and generates a current reference for the current mode operation of the Norton sources. The second type is commonly known as master-slave current-sharing method. The third type has a structure in which Norton current sources represent the parallel-connected converters. In this structure, all converters follow a reference current signal, which is derived from an output voltage feedback loop [9]. In general, the first type is called a passive control method, while the second and third types are called active control methods. The second and third types need a communication link to achieve precise current sharing. A typical active current sharing method for controlling parallel-connected DC-DC converter, which uses a radio-frequency (RF) communication interface in a master-slave arrangement, is presented in [10,11]. One of the parallel modules, which is the master module, uses a voltage control loop to produce the reference current for all parallel converters. The reference current is sent through RF transmission. The delay due to the RF transmission on the stability is investigated to determine a satisfactory operation region. Most of the active current sharing methods for controlling parallel-connected DC-DC converters are based on a high-bandwidth communication network and are utilized in central control [12]. However, because the distributed energy resources in a DC microgrid are connected to the point of common coupling, it is better in terms of investment cost and data reliability to decentralize the primary control of voltage and current sharing. Therefore, the droop method has received more attention in DC microgrid due to the elimination of communication networks.
Although the conventional droop method decentralizes the parallel modules' control, its voltage regulation and current sharing are the main drawbacks [13]. Therefore, several improved droop methods have been presented in the literature to enhance the load current sharing and voltage regulation [14,15,16,17,18,19,20,21]. The most effective approach presented in the literature is based on updating the parallel-connected converters' droop gain. This approach generally is called adaptive droop control [14]. The adaptive droop control method requires knowledge of converter currents that are shared between the parallel-modules by a low bandwidth communication scheme.
Furthermore, low bandwidth communication is used as well to exchange information between parallel converters to improve load sharing and restore the voltage of the common DC bus [15]. The required voltage and current data are sent to the other control system of the other converters to achieve a precise load sharing current and voltage restoration. Virtual resistance (VR) droop methods have been presented in [16,17,18,19,20]. These methods are based on a communication network to determine the virtual droop gain, which improves load sharing and voltage regulation. A tertiary optimization control for parallel-connected DC-DC converter has been proposed in the literature to enhance the virtual resistance droop method's efficiency [16]. By varying the virtual resistance, adjustments in load current sharing between the converter can be made. Stability analysis is studied to demonstrate the effectiveness of varying VR on the dynamic performance of the system. The tertiary optimization control which is based on a hierarchical control system (centralized control) needs a communication network. Another virtual resistance method is presented in [17]. The virtual resistance method is based on the droop index algorithm to minimize the circulating current and improve the load current sharing differences between parallel-connected converters. For the droop index algorithm, a virtual resistance value is calculated based on the output voltage mismatch at the output of the converters and normalized current sharing. Minimization of circulating current is achieved, and the load current is improved during the transient and steady-state conditions. While the droop index is calculated instantaneously using the measurements of the output currents and voltage for each converter, a communication network is required. In [18], a low bandwidth communication network is used in a three-level hierarchical control algorithm. The three-level hierarchical control algorithm is used to minimize the mismatch in the output voltage of the parallel-connected converters due to the droop method. However, the effect of the cable resistance connecting the outputs of the converters to the DC bus is not considered. A decentralized controller is presented in [19], which is based on the droop controller. The voltage in DC microgrid varies due to changes in the load. Thus, achieving current sharing in the converters is challenging. The problem can be alleviated by using another control loop, which uses low bandwidth digital communication between the converters. Another virtual droop gain which is based on an adaptive droop control method is presented in [20]. The method uses instantaneous virtual resistance to eliminate the voltage difference and minimize the current sharing difference when a different cable resistance is presented. However, the instantaneous virtual droop gain is calculated using the normalized current sharing differences and the losses in the converters' output side, which are a function of the individual output voltage and current for both converters. This approach requires a communication link between parallel-connected converters. An offline approach based on optimal values of droop resistances is obtained from minimizing the total current sharing error using the particle swarm optimization (PSO) technique [21]. However, the approach is associated with a heavy mathematical burden and takes a long time to find the optimal values.
Approaches that use modified droop control methods without communication to control active and reactive power in AC microgrids are presented in [22,23,24,25]. However, an alternative droop without communication proposed in this paper is for DC microgrids. The proposed method is utilized to overcome the drawbacks of using communication network and offline optimal droop gain computation in DC microgrid. The alternative droop method overcomes the issues of current sharing and voltage regulation. The proposed method's implementation is based on developing the modified droop method, including the cable resistance and the control algorithm technique. The unequal current sharing, which is due to the cable resistance mismatches, is dynamically improved by implementing a virtual droop gain. The virtual droop gain compensates for the cable resistance mismatches, which connects the two converters to the point of common coupling. A proper load sharing in both transient and steady-state conditions is achieved. The common DC bus voltage is restored using an adaptive voltage gain that estimates the voltage at the common DC bus and shifts the droop gain up or down depending on the load condition to ensure a constant voltage at the load bus. The proposed method is compared with the modified droop method and the control algorithm technique. The comparative results show that the improvement in the system's dynamic responses and restore the voltage at the point of common coupling is achieved by the proposed method. Furthermore, precise load current sharing for the proposed method is achieved with a better dynamic response in comparison to the modified droop method and control algorithm technique. The dynamic performance of the proposed method is tested by using MATLAB simulation and validated using experimental results.
The load regulation characteristics of parallel-connected converters in a DC microgrid affect the voltage deviation of the point of common coupling and the load current sharing. To illustrate, a standalone DC microgrid is constructed by two parallel-connected converters, two cable resistances, and a load resistor, as shown in Figure 2. The two converters are simplified by Thevenin equivalent. They are connected to the common DC bus through cable resistances. The output voltage for each converter, which is based on the droop control method, is given by
Vdcn=VNLn−Kn∗In | (1) |
where Vdcn is the output voltage of convertern, VNLn is the no-load voltage of converter n, Kn is the droop gain of converter n, and In is the output current of converter n.
The voltage at the common DC bus based on Figure 2 can be derived as
VL=VNL1−(K1+Rc1)∗I1 | (2) |
VL=VNL2−(K2+Rc2)∗I2 | (3) |
VL=(I1+I2)∗Rload | (4) |
It can be observed from Figure 3(a) that a small mismatch in the parameters of the parallel-connected converters causes unequal load current sharing between parallel modules. The mismatch in the parameters of the parallel module could result from manufacturing tolerance. Therefore, if the voltage at the common DC bus is Vbus, the current sharing for converter Ⅰ and converter Ⅱ are I1 and I2 respectively, which are unequal. Furthermore, the increase in loading changes the operating point from Vbus to V‘bus, and the load current sharing I‘1 and I‘2 are still unequal. Moreover, if the cable resistances that connect the parallel modules to the common DC bus are different, the load current sharing is degraded, as shown in Figure 3(b). If the cable resistance Rc2 is greater than the cable resistance Rc1, the load current sharing for converter Ⅰ and converter Ⅱ are degraded from I1 and I2 to I‘1 and I‘2 respectively. In other words, the differences in cable resistance worsen the load current sharing according to the load regulation characteristic of each converter. Furthermore, the third limitation of the droop method is the voltage deviation. According to (1)-(3), the droop gains for converter Ⅰ and Ⅱ cause the output voltage to decrease when the load current increases. Figure 3(c) shows the load regulation characteristics of two parallel modules with different droop gain K1 and K2. However, the voltage deviation increases when the droop gain is higher, as shown in Figure 3(c). Therefore, the droop gains K‘1 and K‘2, which are higher than K1and K2 for converter Ⅰ and converter Ⅱ, respectively, increase the voltage deviation for the same load currents.
The main purpose of the droop control method is to control parallel-connected converters for achieving equal load current sharing and maintaining acceptable voltage at the point of common coupling. In DC microgrid, the point of common coupling makes the droop control method more attractive compared to the active current sharing methods. In this section, an overview of the modified droop method, including the cable resistance and control algorithm technique, is summarized [26,27]. Since the alternative droop strategy utilizes the modified droop method and control algorithm technique, it is presented and explained accordingly in this section.
The modified droop method with cable resistance implementation is reported in [26]. The method is used to estimate the voltage set point for each controller according to the converters' droop characteristics. The first step for estimating the voltage set point uses the measurements of voltage and current at the common DC bus to determine the load resistance as
Rload=VLIL | (5) |
The modified droop method including cable resistances estimates the load current sharing, which determined by solving Eqs (2), (3), and (4) as
I1=V2NL/Rload−V1NL/Rload2∗(K2+RC2+Rload)1−1/Rload2∗(K1+RC1+Rload)∗(K2+RC2+Rload) | (6) |
I2=V1NL/Rload−V2NL/Rload2∗(K1+RC1+Rload)1−1/Rload2∗(K1+RC1+Rload)∗(K2+RC2+Rload) | (7) |
The voltage set-points for the parallel-connected converters are determined based on the estimated current sharing values and the common DC bus voltage. The set-point of the output voltage of each converter is determined locally without transmitting voltage and current data from one converter to the other as
Vsetponit_1=VL+Rc1∗I1 | (8) |
Vsetpoint_2=VL+Rc2∗I2 | (9) |
The block diagram and flowchart for estimating current sharing and the output voltage set-point of the modified droop with cable resistance are shown in Figure 4 and Figure 5, respectively.
Another loop control that is added to the modified droop method is reported in [27]. The addition loop control is responsible for ensuring a precise current sharing when there is a mismatch in parallel modules' parameters. It compares the current sharing for each converter with the load current. If the percentage of current sharing for converter Ⅰ is lower than 50%, an additional ΔV is added to the converter's set point to increase its current sharing to the load current, and vice versa. A small value ΔV is chosen to prevent an oscillatory output current. Figure 6 shows the block diagram of the modified droop method along with the additional control algorithm.
In the proposed approach, two gains are added to the modified droop control method. These gains are a virtual droop gain VDG, and an adaptive voltage control gain AVCG. The virtual droop gain can improve the system's dynamic response for load current sharing between parallel modules and the adaptive voltage control gain can regulate the voltage. First of all, the virtual droop gain can be added to the droop Eqs (2) and (3) as
VL=VNL1−(K1+Rc1+Kv1)∗I1 | (10) |
VL=VNL2−(K2+Rc2+Kv1)∗I2 | (11) |
where Kv1 and Kv2 are the virtual droop gain for converter Ⅰ and Ⅱ, respectively. The virtual gains can be selected based on the differences in cable resistances. To illustrate, Figure 7(a) shows the load regulation characteristics for two parallel-connected converters. The cable resistances Rc1 and Rc2 connect converter Ⅰ and Ⅱ to the common DC bus, and Rc2 is greater than Rc1. Thus, according to the droop method, converter Ⅰ experiences higher current compared to converter Ⅱ.
Since the current sharing between the two parallel-connected converters is unequal, the values of Kv1 and Kv2 can be chosen based on the cable resistance compensation. To retrieve the original load regulation characteristics, the virtually gains Kv1 and Kv2 can compensate for Rc2 and Rc1 respectively as shown in Figure 7(b).
Secondly, the adaptive voltage control gain is determined based on the estimated voltage at the common DC bus, which is determined from the modified droop method and compared with its rated value. Thus, The AVCG can be determined as
AVCG=VCC−Vrated | (12) |
For various load conditions, the voltage at the point of common coupling PCC is regulated by using its estimated value, as shown in Figure 8.
The steps that are used to regulate the voltage at the common DC bus by utilizing the AVCG are:
1-Measure the voltage at the PCC (VL) and the total load current (IL). The load resistor can be determined as given in Eq (5)
2-Based on the modified droop method with cable resistance implementation, the estimated output current for converter Ⅰ and converter Ⅱ can be determined by using Eqs (6) and (7).
3- The estimated voltage (VCC) at the common DC bus can be obtained as
VCC=VNL1−(R1+K1)∗I1 | (13) |
VCC=VNL2−(R2+K2)∗I2 | (14) |
The AVCG can be determined by using Eq (12). Thus, if the AVCG has a negative value, the droop method's load characteristic regulation is shifted up to a newer load characteristics regulation, as shown in Figure 8. Since the measurements are at the point of common coupling point, the AVCG has the same value for the two boost converters' local controller. Thus, the newer load characteristics regulations for both converters are shifted from the load regulation characteristics synchronously. The voltage position ensures that the voltage at the point of common coupling is at the rated value. However, suppose the AVCG has a positive value. In that case, the droop method's load characteristic regulations, for both converters, are shifted down to a newer load characteristics regulation, as shown in Figure 8. Therefore, the newer load characteristic regulation adjusts the voltage at the point of common coupling synchronously to the required value, which is the rated voltage for the DC microgrid. The block diagram for implementing the proposed approach, which improves the dynamic response of the unequal current sharing due to different cable resistances and voltage deviation, is shown in Figure 9.
The Matlab/Simulink environment is used to demonstrate the effectiveness of the alternative droop method. The parameters of the system are given in Table 1. The boost converter parameters are determined based on the continuous current conduction mode of operation [28].
Parameters | DC-DC Boost Converter Ⅰ | DC-DC Boost Converter Ⅱ |
Switching frequency fS | 25 kHz | 25 kHz |
Inductance L | 9.136 mH | 10.200 mH |
Capacitance C | 452 µF | 430 µF |
Voltage V | 6–12 V | 6–12 V |
Furthermore, the voltage and current PI controllers in Figure 4, Figure 6, and Figure 9 are designed based on the state space averaging technique [29]. The SISOTOOL in MATLAB is used to determine the parameters of the PI controller, which are given in Table 2 [30].
Parameters | Voltage control loop | Current control loop |
Proportional Gain kP | 0.182 | 0.114 |
Integral Gain kI | 23.364 | 19 |
The droop gains for converter Ⅰ and converter Ⅱ are determined based on two operating points, which are the no-load and full-load voltage and current [31,32]. The values for the droop gains K1 and K2, which are used in the simulated and experimental modules, are 0.8133 and 0.8182, respectively. In this paper, different cable resistances are selected: the values of Rc1 and Rc2 are equal to 0.2 Ω and 0.1 Ω, respectively to verify the effectiveness of the proposed method. Thus, the virtual gains are selected to compensate for the differences in the cable resistances Rc1 and Rc2. The values of virtual gains Kv1 and Kv2 are 0.1 and 0.2 respectively. Furthermore, two different values of load resistances are used to compare the performance of the proposed method compared to the modified droop method. However, in the Matlab simulation study, three cases are tested to demonstrate the feasibility of the proposed method. Case-1 presents the results of the modified droop method based on the block diagram of Figure 4. Case-2 presents the modified droop method with a control algorithm technique shown in Figure 6. Case-3 presents the results of the proposed method shown in Figure 9.
In Case-1, the voltage and current sharing responses between the two parallel-connected converters are tested with an increase in the load from 15.51 Ω and 13.804 Ω. The transient responses of the current sharing for converter Ⅰ and converter Ⅱ and the total load current are shown in Figure 10. The step increase in load occurs at 3 seconds. It can be noticed that the load current for the two parallel-connected boost converters is not shared equally.
Furthermore, the transient responses of the output voltage for converter Ⅰ and converter Ⅱ and the load voltage for the step increase in the load are shown in Figure 11. It can be observed that when the step increase in the load is placed, the voltage at the load or common DC bus decreases, which is the main drawback of the conventional droop method.
The steady-state values for the output voltage of converter Ⅰ and converter Ⅱ, the voltage at the common DC bus, load current sharing, and the percentage deviation of the current sharing for case-1 are shown in Table 3.
Time | 1-3 second | 3-5 second |
I1 (A) | 0.346 | 0.39 |
I2 (A) | 0.425 | 0.475 |
IL (A) | 0.771 | 0.864 |
Vdc1 (V) | 12.04 | 12 |
Vdc2 (V) | 12.01 | 11.975 |
VL (V) | 11.97 | 11.925 |
ΔI (%) current sharing differences | 10.25 | 9.84 |
It can be observed from Table 3 that the voltage drops from 11.97 V to 11.925 V at the common DC bus when a step increase in the load is applied. Furthermore, the load current sharing is unequal due to the mismatches in the converters' parameters and the differences in cable resistances.
In Case-2, the control algorithm is implemented with the modified droop method. The block diagram in Figure 6 is simulated using MATLAB/Simulink environment. For the same load condition in Case-1, the output currents transient response for converter I, converter Ⅱ and the total load current are shown in Figure 12.
Although the load current is shared equally between the parallel-connected boost converters, as shown in Figure 12, the common DC bus's output voltage is dropped, as shown in Figure 13.
It can be observed from Figure 13, and the load voltage drops from 11.96 V to 11.92 V. The steady-state values for the output voltages and currents for converter Ⅰ and converter Ⅱ and common DC bus's voltage are given in Table 4.
Time | 1-3 second | 3-5 second |
I1 (A) | 0.385 | 0.43 |
I2 (A) | 0.385 | 0.43 |
IL (A) | 0.77 | 0.86 |
Vdc1 (V) | 12.04 | 12.01 |
Vdc2 (V) | 12.01 | 11.96 |
VL (V) | 11.96 | 11.92 |
ΔI (%) current sharing differences | 0 | 0 |
From Table 4, the steady-state values of the output currents for converter Ⅰ and converter Ⅱ are equal, but the voltage at the common DC bus (load voltage) drops from 11.96 V to 11.92 V due to the droop action. Thus, including the control algorithm with the modified droop method can enhance the load sharing, but it does not restore the voltage at the common DC bus to its rated value (12 V).
To compare the proposed method with the modified droop control method and the modified droop method with control algorithm, the block diagram of the proposed method in Figure 9 is simulated using MATLAB/Simulink environment. The results are based on the same loading condition and cable resistances in Case-1 and Case-2. In Case-3, the cable resistances that connect each converter to the common DC bus are considered as 0.2 Ω and 0.1 Ω for Rc1 and Rc2 respectively. The values of the virtual droop gain are set at 0.1 and 0.2 for Kv1 and Kv2 respectively. Different load resistances are used to test the performance of the proposed method. The transient responses for the current sharing accuracy enhancement of converter I and converter II along with the load current are shown in Figure 14, where 15.51 Ω and 13.804 Ω load resistances are applied, respectively.
From Figure 14, it can be observed that the output currents of the parallel-connected converter are equal for different loading conditions. Furthermore, the proposed method restores the voltage at the common DC bus. The response of the output voltage for converter Ⅰ and converter Ⅱ and the common DC bus voltage are shown in Figure 15. The voltage at the common DC bus is restored when a step increase in the load demand is applied.
The steady-state values of the simulated results for the proposed method given in Table 5 show that the current sharing accuracy is enhanced, and the voltage at the common DC bus is restored to its rated value.
Time | 1-3 second | 3-5 second |
I1 (A) | 0.387 | 0.435 |
I2 (A) | 0.387 | 0.435 |
IL (A) | 0.774 | 0.87 |
Vdc1 (V) | 12.075 | 12.085 |
Vdc2 (V) | 12.036 | 12.04 |
VL (V) | 12 | 12 |
ΔI (%) current sharing differences | 0 | 0 |
The comparison between the steady-state values of the load current sharing and the voltage at the common DC bus for the three cases indicates that the proposed alternative droop control method shows an improved performance over the modified droop method and the modified droop method with control algorithm. Although the modified droop method with control algorithm provides a precise load current sharing, it has poor voltage regulation at the common DC bus. The performance of the modified droop method with control algorithm degrades as the mismatches of the cable resistance increase. To illustrate, the block diagram of the modified droop method with the control algorithm, as shown in Figure 6 is simulated using MATLAB/Simulink environment for larger differences in the cable resistances compared to the used values of the previous cases. The cable resistance values of Rc1=0.4Ω and Rc2=0.1Ω are used to connect converter I and converter II respectively to the common DC bus. The results are compared with the proposed alternative droop method, which uses virtual droop gain of Kv1=0.1 and Kv2=0.4. The comparison considers the same load condition and the same value of ΔV for regulating the common DC bus. Figure 16 shows the dynamic response for the load current sharing of the control algorithm in comparison to the alternative droop method.
From Figure 16, it can be observed that the dynamic response of the alternative droop method is better than the modified droop with control algorithm. The required time to reach a precise load current sharing for the alternative droop method is 0.2 seconds compared to 2.3 seconds for the modified droop method with control algorithm. After a step increase in the load, the alternative droop method also tracks the operating point faster than the modified droop method with control algorithm. The main reason for improving the dynamic response of the proposed is the utilization of the virtual droop gains, which compensate for the large mismatches of the cable resistance. The virtual droop gain shortens the time to reach the desired operating point, which enhances the dynamic response of the parallel-connected converter system. From Figure 16, the output currents of converter Ⅰ and converter Ⅱ for the proposed method are slightly higher than the output currents of the modified droop method with control algorithm because the proposed method restores the voltage at the common DC bus to its rated value of 12 V.
To validate the proposed alternative droop method, a laboratory prototype of two boost converter is implemented, as shown in Figure 17. In real-time control, the simulated model is compiled by dSPACE 1104. The parameters of the two boost converters that are given in Table 1 are used. Furthermore, to test the proposed control method, different cable resistance of 0.2 Ω and 0.1 Ω are used to connect converter Ⅰ and Ⅱ respectively to the common DC bus. Two different load resistance of 15.51 Ω and 13.804 Ω are employed respectively, to compare the simulated MATLAB module with real-time implementation.
Since the signal applied to DSPACE 1104 A/D channel is limited to ± 10 V, the voltage and current sensors' measurements have a conversion ratio of 30-10 V and 10-1 V, respectively, and software protection in Matlab/Simulink is used to prevent signals greater than ±10V. Moreover, because the D/A channel of the dSPACE 1104 is limited to ±10 V, a gate driver is used to provide PWM of 15 V and isolate the dSPACE 1104 from the actual hardware. Initially, the load is set to 15.51 Ω. The output current for converter Ⅰ and converter Ⅱ and the load current is shown in Figure 18. When a step increase in the load from 15.51 Ω to 13.804 Ω is applied, the transient response for the proposed method validates the accuracy of current sharing between the two converters, as shown in Figure 18.
The output voltage waveforms of converter Ⅰ and converter Ⅱ and at the common DC bus are shown in Figure 19. It can be observed that the voltage at the common DC bus is kept at a rated value of 12 V during the step change in the load.
For the two load resistance values of 15.51 Ω to 13.804 Ω, the steady-state values of the load current sharing and the voltage at the common DC bus along with the estimated value of the voltage by the proposed method are given in Table 6. The percentages of current deviation that is carried out from the real-time implementation validate the proposed method.
Load resistance | 15.51 Ω | 13.804 Ω |
I1 (A) | 0.388 | 0.4355 |
I2 (A) | 0.388 | 0.4355 |
IL (A) | 0.777 | 0.871 |
Vestimated (V) | 11.951 | 11.905 |
Furthermore, the estimated voltage by the proposed method is observed as shown in Table 6. The value is used to adjust the regulation characteristics of the droop method. Therefore, the voltage at the common DC bus or load is maintained at its rated value during different load conditions.
The alternative droop method for controlling parallel-connected converters is presented. The proposed method enhances current sharing by implementing the virtual droop gain, which modifies the regulation characteristics of the droop method. Furthermore, the adaptive voltage control gain restores the voltage at the common DC bus at its rated value. The adaptive voltage control gain used the estimated voltage at the common DC bus to shift the droop method's regulation characteristics. The alternative droop method is compared with the modified droop method and the modified droop method with control algorithm. The results show that the alternative droop method is better than the modified droop method in terms of voltage restoration and precise load current sharing. The proposed method's performance is also better than the modified droop method with control algorithm in terms of the voltage restoration and the dynamic response of reaching precise load current sharing. The alternative droop method is verified using MATLAB/Simulink, and the experimental validation of the simulated method is proved.
The authors would like to thank the Libyan government for funding this research.
All authors declare no conflicts of interest in this paper.
[1] |
Meites J (1988) Neuroendocrine biomarkers of aging in the rat. Exp Gerontol 23: 349-358. doi: 10.1016/0531-5565(88)90037-X
![]() |
[2] | Bellinger DL, Lorton D, Lubahn C, et al. (2001) Innervation of lymphoid organs—Association of nerves with cells of the immune system and their implications in disease. Psychoneuroimmunology San Diego: Academic Press, 5-111. |
[3] |
Downs JL, Wise PM (2009) The role of the brain in female reproductive aging. Mol Cell Endocrinol 299: 32-38. doi: 10.1016/j.mce.2008.11.012
![]() |
[4] |
Banks WA (2015) The blood-brain barrier in neuroimmunology: tales of separation and assimilation. Brain Behav Immun 44: 1-8. doi: 10.1016/j.bbi.2014.08.007
![]() |
[5] |
Bellinger DL, Millar BA, Perez S, et al. (2008) Sympathetic modulation of immunity: relevance to disease. Cell Immunol 252: 27-56. doi: 10.1016/j.cellimm.2007.09.005
![]() |
[6] | ThyagaRajan S, Priyanka HP (2012) Bidirectional communication between the neuroendocrine system and the immune system: relevance to health and diseases. Ann Neurosci 19: 40-46. |
[7] | Pratap U, Hima L, Kannan T, et al. (2020) Sex-Based Differences in the Cytokine Production and Intracellular Signaling Pathways in Patients With Rheumatoid Arthritis. Arch Rheumatol 35: i-xiii. |
[8] |
Hima L, Patel MN, Kannan T, et al. (2020) Age-associated decline in neural, endocrine, and immune responses in men and women: Involvement of intracellular signaling pathways. J Neuroimmunol 345: 577290. doi: 10.1016/j.jneuroim.2020.577290
![]() |
[9] |
ThyagaRajan S, Hima L, Pratap UP, et al. (2019) Estrogen-induced neuroimmunomodulation as facilitator of and barrier to reproductive aging in brain and lymphoid organs. J Chem Neuroanat 95: 6-12. doi: 10.1016/j.jchemneu.2018.02.008
![]() |
[10] |
Randolph JF, Sowers M, Bondarenko IV, et al. (2004) Change in estradiol and follicle-stimulating hormone across the early menopausal transition: effects of ethnicity and age. J Clin Endocrinol Metab 89: 1555-1561. doi: 10.1210/jc.2003-031183
![]() |
[11] |
Kermath BA, Gore AC (2012) Neuroendocrine control of the transition to reproductive senescence: lessons learned from the female rodent model. Neuroendocrinol 96: 1-12. doi: 10.1159/000335994
![]() |
[12] |
Harlow SD, Mitchell ES, Crawford S, et al. (2008) The ReSTAGE Collaboration: defining optimal bleeding criteria for onset of early menopausal transition. Fertil Steril 89: 129-40. doi: 10.1016/j.fertnstert.2007.02.015
![]() |
[13] |
Wang Y, Mishra A, Brinton RD (2020) Transitions in metabolic and immune systems from pre-menopause to post-menopause: implications for age-associated neurodegenerative diseases. F1000 Res 9: 68. doi: 10.12688/f1000research.21599.1
![]() |
[14] |
Finkelstein JS, Brockwell SE, Mehta V, et al. (2008) Bone mineral density changes during the menopause transition in a multiethnic cohort of women. J Clin Endocrinol Metab 93: 861-868. doi: 10.1210/jc.2007-1876
![]() |
[15] |
Brann DW, Mahesh VB (2005) The aging reproductive neuroendocrine axis. Steroids 70: 273-283. doi: 10.1016/j.steroids.2004.12.008
![]() |
[16] |
Wise PM (2005) Estrogens and cerebrovascular stroke: what do animal models teach us? Ann N Y Acad Sci 1052: 225-232. doi: 10.1196/annals.1347.017
![]() |
[17] |
Wise PM, Scarbrough K, Lloyd J, et al. (1994) Neuroendocrine concomitants of reproductive aging. Exp Gerontol 29: 275-283. doi: 10.1016/0531-5565(94)90007-8
![]() |
[18] |
MohanKumar PS, ThyagaRajan S, Quadri SK (1995) Cyclic and age-related changes in norepinephrine concentrations in the medial preoptic area and arcuate nucleus. Brain Res Bull 38: 561-564. doi: 10.1016/0361-9230(95)02031-4
![]() |
[19] |
MohanKumar PS, ThyagaRajan S, Quadri SK (1997) Tyrosine hydroxylase and DOPA decarboxylase activities in the medial preoptic area and arcuate nucleus during the estrus cycle: effects of aging. Brain Res Bull 42: 265-271. doi: 10.1016/S0361-9230(96)00210-9
![]() |
[20] |
Wise PM (1982) Norepinephrine and dopamine activity in micro dissected brain areas of the middle-aged and young rat on proestrus. Biol Reprod 27: 562-574. doi: 10.1095/biolreprod27.3.562
![]() |
[21] |
Wise PM (1984) Estradiol-induced daily luteinizing hormone and prolactin surges in young and middle-aged rats: correlations with age-related changes in pituitary responsiveness and catecholamine turnover rates in micro dissected brain areas. Endocrinology 115: 801-809. doi: 10.1210/endo-115-2-801
![]() |
[22] |
ThyagaRajan S, Madden KS, Teruya B, et al. (2011) Age-associated alterations in sympathetic noradrenergic innervation of primary and secondary lymphoid organs in female Fischer 344 rats. J Neuroimmunol 233: 54-64. doi: 10.1016/j.jneuroim.2010.11.012
![]() |
[23] |
Chakrabarti M, Haque A, Banik NL, et al. (2014) Estrogen receptor agonists for attenuation of neuroinflammation and neurodegeneration. Brain Res Bull 109: 22-31. doi: 10.1016/j.brainresbull.2014.09.004
![]() |
[24] |
Ravichandran KA, Karrunanithi S, Hima L, et al. (2017) Estrogen differentially regulates the expression of tyrosine hydroxylase and nerve growth factor through free radical generation in the thymus and mesenteric lymph nodes of middle-aged ovariectomized female Sprague-Dawley rats. Clin Exp Neuroimmunol 8: 341-350. doi: 10.1111/cen3.12415
![]() |
[25] |
Priyanka HP, Sharma U, Gopinath S, et al. (2013) Menstrual cycle and reproductive aging alters immune reactivity, NGF expression, antioxidant enzyme activities, and intracellular signalling pathways in the peripheral blood mononuclear cells of healthy women. Brain Behav Immun 32: 131-143. doi: 10.1016/j.bbi.2013.03.008
![]() |
[26] |
Wise PM, Smith MJ, Dubal DB, et al. (2002) Neuroendocrine modulation and repercussions of female reproductive aging. Recent Prog Horm Res 57: 235-256. doi: 10.1210/rp.57.1.235
![]() |
[27] |
Murray RW (2001) Estrogen, prolactin, and autoimmunity: actions and interactions. Int Immunopharmacol 1: 995-1008. doi: 10.1016/S1567-5769(01)00045-5
![]() |
[28] |
Salem ML (2004) Estrogen, a double-edged sword: modulation of TH1- and TH2-mediated inflammations by differential regulation of TH1/TH2 cytokine production. Curr Drug Targets Inflamm Allergy 3: 97-104. doi: 10.2174/1568010043483944
![]() |
[29] |
Lang TJ (2004) Estrogen as an immunomodulator. Clin Immunol 113: 224-230. doi: 10.1016/j.clim.2004.05.011
![]() |
[30] | Krzych U, Strausser HR, Bressler JP, et al. (1978) Quantitative differences in immune responses during the various stages of the estrus cycle in female BALB/c mice. J Immunol 121: 1603-1605. |
[31] |
Straub RH (2007) The complex role of estrogens in inflammation. Endocr Rev 28: 521-574. doi: 10.1210/er.2007-0001
![]() |
[32] |
Lasarte S, Elsner D, Sanchez-Elsner T, et al. (2013) Estradiol down regulates NF-κB translocation by Ikbkg transcriptional repression in dendritic cells. Genes Immun 14: 462-469. doi: 10.1038/gene.2013.35
![]() |
[33] |
Maret A, Coudert JD, Garidou L, et al. (2003) Estradiol enhances primary antigen-specific CD4 T cell responses and Th1 development in vivo. Essential role of estrogen receptor alpha expression in hematopoietic cells. Eur J Immunol 33: 512-521. doi: 10.1002/immu.200310027
![]() |
[34] |
Priyanka HP, Krishnan HC, Singh RV, et al. (2013) Estrogen modulates in vitro T cell responses in a concentration- and receptor-dependent manner: effects on intracellular molecular targets and antioxidant enzymes. Mol Immunol 56: 328-339. doi: 10.1016/j.molimm.2013.05.226
![]() |
[35] |
Maglione A, Rolla S, Mercanti SF, et al. (2019) The Adaptive Immune System in Multiple Sclerosis: An Estrogen-Mediated Point of View. Cells 8: 1280. doi: 10.3390/cells8101280
![]() |
[36] |
Ysrraelit MC, Correale J (2019) Impact of sex hormones on immune function and multiple sclerosis development. Immunology 156: 9-22. doi: 10.1111/imm.13004
![]() |
[37] |
Cutolo M, Sulli A, Capellino S, et al. (2004) Sex hormones influence on the immune system: basic and clinical aspects in autoimmunity. Lupus 13: 635-638. doi: 10.1191/0961203304lu1094oa
![]() |
[38] |
Chakrabarti M, Haque A, Banik NL, et al. (2014) Estrogen receptor agonists for attenuation of neuroinflammation and neurodegeneration. Brain Res Bull 109: 22-31. doi: 10.1016/j.brainresbull.2014.09.004
![]() |
[39] |
Babayan AH, Kramár EA (2013) Rapid effects of oestrogen on synaptic plasticity: interactions with actin and its signalling proteins. J Neuroendocrinol 25: 1163-1172. doi: 10.1111/jne.12108
![]() |
[40] |
Mónica Brauer M, Smith PG (2015) Estrogen and female reproductive tract innervation: cellular and molecular mechanisms of autonomic neuroplasticity. Auton Neurosci 187: 1-17. doi: 10.1016/j.autneu.2014.11.009
![]() |
[41] |
Priyanka HP, ThyagaRajan S (2013) Selective modulation of lymphoproliferation and cytokine production via intracellular signalling targets by α1- and α2-adrenoceptors and estrogen in splenocytes. Int Immunopharmacol 17: 774-784. doi: 10.1016/j.intimp.2013.08.020
![]() |
[42] |
Priyanka HP, Pratap UP, Singh RV, et al. (2014) Estrogen modulates β2-adrenoceptor-induced cell-mediated and inflammatory immune responses through ER-α involving distinct intracellular signaling pathways, antioxidant enzymes, and nitric oxide. Cell Immunol 292: 1-8. doi: 10.1016/j.cellimm.2014.08.001
![]() |
[43] |
Scanzano A, Schembri L, Rasini E, et al. (2015) Adrenergic modulation of migration, CD11b and CD18 expression, ROS and interleukin-8 production by human polymorphonuclear leukocytes. Inflamm Res 64: 127-135. doi: 10.1007/s00011-014-0791-8
![]() |
[44] |
Prey S, Leaute-Labreze C, Pain C, et al. (2014) Mast cells as possible targets of propranolol therapy: an immunohistological study of beta-adrenergic receptors in infantile haemangiomas. Histopathology 65: 436-439. doi: 10.1111/his.12421
![]() |
[45] |
Du Y, Yan L, Du H, et al. (2012) β1-adrenergic receptor autoantibodies from heart failure patients enhanced TNF-α secretion in RAW264.7 macrophages in a largely PKA-dependent fashion. J Cell Biochem 113: 3218-3228. doi: 10.1002/jcb.24198
![]() |
[46] |
Yang H, Du RZ, Qiu JP, et al. (2013) Bisoprolol reverses epinephrine-mediated inhibition of cell emigration through increases in the expression of β-arrestin 2 and CCR7 and PI3K phosphorylation, in dendritic cells loaded with cholesterol. Thromb Res 131: 230-237. doi: 10.1016/j.thromres.2012.12.009
![]() |
[47] |
Markus T, Hansson SR, Cronberg T, et al. (2010) β-Adrenoceptor activation depresses brain inflammation and is neuroprotective in lipopolysaccharide-induced sensitization to oxygen-glucose deprivation in organotypic hippocampal slices. J Neuroinflammation 7: 94. doi: 10.1186/1742-2094-7-94
![]() |
[48] |
Cunningham M, Gilkeson G (2011) Estrogen receptors in immunity and autoimmunity. Clin Rev Allergy Immunol 40: 66-73. doi: 10.1007/s12016-010-8203-5
![]() |
[49] |
Geserick C, Meyer HA, Haendler B (2005) The role of DNA response elements as allosteric modulators of steroid receptor function. Mol Cell Endocrinol 236: 1-7. doi: 10.1016/j.mce.2005.03.007
![]() |
[50] |
Li J, McMurray RW (2006) Effects of estrogen receptor subtype-selective agonists on immune functions in ovariectomized mice. Int Immunopharmacol 6: 1413-1423. doi: 10.1016/j.intimp.2006.04.019
![]() |
[51] |
Li J, McMurray RW (2010) Effects of cyclic versus sustained estrogen administration on peripheral immune functions in ovariectomized mice. Am J Reprod Immunol 63: 274-281. doi: 10.1111/j.1600-0897.2009.00784.x
![]() |
[52] |
Delpy L, Douin-Echinard V, Garidou L, et al. (2005) Estrogen enhances susceptibility to experimental autoimmune myasthenia gravis by promoting type 1-polarized immune responses. J Immunol 175: 5050-5057. doi: 10.4049/jimmunol.175.8.5050
![]() |
[53] |
Murphy AJ, Guyre PM, Pioli PA (2010) Estradiol suppresses NF-kappa B activation through coordinated regulation of let-7a and miR-125b in primary human macrophages. J Immunol 184: 5029-5037. doi: 10.4049/jimmunol.0903463
![]() |
[54] |
Tiwari-Woodruff S, Voskuhl RR (2009) Neuroprotective and anti-inflammatory effects of estrogen receptor ligand treatment in mice. J Neurol Sci 286: 81-85. doi: 10.1016/j.jns.2009.04.023
![]() |
[55] |
Hildebrand F, Hubbard WJ, Choudhry MA, et al. (2006) Are the protective effects of 17 beta-estradiol on splenic macrophages and splenocytes after trauma-haemorrhage mediated via estrogen-receptor (ER)-alpha or ER-beta? J Leukoc Biol 79: 1173-1180. doi: 10.1189/jlb.0106029
![]() |
[56] |
Kawasaki T, Suzuki T, Choudhry MA, et al. (2010) Salutary effects of 17 beta-estradiol on Peyer's patch T cell functions following trauma-haemorrhage. Cytokine 51: 166-172. doi: 10.1016/j.cyto.2010.03.016
![]() |
[57] |
Suzuki T, Yu HP, Hsieh YC, et al. (2008) Mitogen activated protein kinase (MAPK) mediates non-genomic pathway of estrogen on T cell cytokine production following trauma-haemorrhage. Cytokine 42: 32-38. doi: 10.1016/j.cyto.2008.02.002
![]() |
[58] |
Liao ZH, Huang T, Xiao JW, et al. (2019) Estrogen signaling effects on muscle-specific immune responses through controlling the recruitment and function of macrophages and T cells. Skeletal Muscle 9: 20. doi: 10.1186/s13395-019-0205-2
![]() |
[59] | Khan D, Ansar Ahmed S (2015) The Immune System Is a Natural Target for Estrogen Action: Opposing Effects of Estrogen in Two Prototypical Autoimmune Diseases. Front Immunol 6: 635. |
[60] | Spengler RN, Allen RM, Remick DG, et al. (1990) Stimulation of alpha-adrenergic receptor augments the production of macrophage-derived tumour necrosis factor. J Immunol 145: 1430-1434. |
[61] |
Prossnitz ER, Barton M (2011) The G-protein-coupled estrogen receptor GPER in health and disease. Nat Rev Endocrinol 7: 715-726. doi: 10.1038/nrendo.2011.122
![]() |
[62] |
Bourque M, Dluzen DE, Di Paolo T (2012) Signalling pathways mediating the neuroprotective effects of sex steroids and SERMs in Parkinson's disease. Front Neuroendocrinol 33: 169-178. doi: 10.1016/j.yfrne.2012.02.003
![]() |
[63] |
Lebesgue D, Chevaleyre V, Zukin RS, et al. (2009) Estradiol rescues neurons from global ischemia-induced cell death: multiple cellular pathways of neuroprotection. Steroids 74: 555-561. doi: 10.1016/j.steroids.2009.01.003
![]() |
[64] |
Thomas W, Coen N, Faherty S, et al. (2006) Estrogen induces phospholipase A2 activation through ERK1/2 to mobilize intracellular calcium in MCF-7 cells. Steroids 71: 256-265. doi: 10.1016/j.steroids.2005.10.010
![]() |
[65] |
Titolo D, Mayer CM, Dhillon SS, et al. (2008) Estrogen facilitates both phosphatidylinositol 3-kinase/Akt and ERK1/2 mitogen-activated protein kinase membrane signalling required for long-term neuropeptide Y transcriptional regulation in clonal, immortalized neurons. J Neurosci 28: 6473-6482. doi: 10.1523/JNEUROSCI.0514-08.2008
![]() |
[66] |
Milette S, Hashimoto M, Perrino S, et al. (2019) Sexual dimorphism and the role of estrogen in the immune microenvironment of liver metastases. Nat Commun 10: 5745. doi: 10.1038/s41467-019-13571-x
![]() |
[67] |
Wade CB, Dorsa DM (2003) Estrogen activation of cyclic adenosine 5′-monophosphate response element mediated transcription requires the extracellularly regulated kinase/mitogen-activated protein kinase pathway. Endocrinology 144: 832-838. doi: 10.1210/en.2002-220899
![]() |
[68] |
Fernandez SM, Lewis MC, Pechenino AS, et al. (2008) Estradiol-induced enhancement of object memory consolidation involves hippocampal ERK activation and membrane-bound estrogen receptors. J Neurosci 28: 8660-8667. doi: 10.1523/JNEUROSCI.1968-08.2008
![]() |
[69] |
Carlstrom L, Ke ZJ, Unnerstall JR, et al. (2001) Estrogen modulation of the cyclic AMP response element-binding protein pathway. Effects of long-term and acute treatments. Neuroendocrinology 74: 227-243. doi: 10.1159/000054690
![]() |
[70] |
Grove-Strawser D, Boulware MI, Mermelstein PG (2010) Membrane estrogen receptors activate the metabotropic glutamate receptors mGluR5 and mGluR3 to bidirectionally regulate CREB phosphorylation in female rat striatal neurons. Neuroscience 170: 1045-1055. doi: 10.1016/j.neuroscience.2010.08.012
![]() |
[71] |
Sanchez MG, Morissette M, Di Paolo T (2012) Effect of a chronic treatment with 17 β-estradiol on striatal dopamine neurotransmission and the Akt/GSK3 signalling pathway in the brain of ovariectomized monkeys. Psychoneuroendocrinology 37: 280-291. doi: 10.1016/j.psyneuen.2011.06.012
![]() |
[72] |
Zhang QG, Wang R, Tang H, et al. (2014) Brain-derived estrogen exerts anti-inflammatory and neuroprotective actions in the rat hippocampus. Mol Cell Endocrinol 389: 84-91. doi: 10.1016/j.mce.2013.12.019
![]() |
[73] |
Pratap UP, Patil A, Sharma HR, et al. (2016) Estrogen-induced neuroprotective and anti-inflammatory effects are dependent on the brain areas of middle-aged female rats. Brain Res Bull 124: 238-253. doi: 10.1016/j.brainresbull.2016.05.015
![]() |
[74] |
Maharjan S, Serova LI, Sabban EL (2010) Membrane-initiated estradiol signalling increases tyrosine hydroxylase promoter activity with ER alpha in PC12 cells. J Neurochem 112: 42-55. doi: 10.1111/j.1471-4159.2009.06430.x
![]() |
[75] |
Pendergast JS, Tuesta LM, Bethea JR (2008) Oestrogen receptor beta contributes to the transient sex difference in tyrosine hydroxylase expression in the mouse locus coeruleus. J Neuroendocrinol 20: 1155-1164. doi: 10.1111/j.1365-2826.2008.01776.x
![]() |
[76] |
Thanky NR, Son JH, Herbison AE (2002) Sex differences in the regulation of tyrosine hydroxylase gene transcription by estrogen in the locus coeruleus of TH9-LacZ transgenic mice. Brain Res Mol Brain Res 104: 220-226. doi: 10.1016/S0169-328X(02)00383-2
![]() |
[77] |
Yamaguchi N, Yuri K (2014) Estrogen-dependent changes in estrogen receptor-β mRNA expression in middle-aged female rat brain. Brain Res 1543: 49-57. doi: 10.1016/j.brainres.2013.11.010
![]() |
[78] |
Foster TC (2012) Role of estrogen receptor alpha and beta expression and signalling on cognitive function during aging. Hippocampus 22: 656-669. doi: 10.1002/hipo.20935
![]() |
[79] | Coyoy-Salgado A, Segura-Uribe JJ, Manuel Gallardo J, et al. (2020) Tibolone regulates systemic metabolism and the expression of sex hormone receptors in the central nervous system of ovariectomised rats fed with high-fat and high-fructose diet. Brain Res 6: 1748. |
[80] | Zoubina EV, Mize AL, Alper RH, et al. (2001) Acute and chronic estrogen supplementation decreases uterine sympathetic innervation in ovariectomized adult virgin rats. Histol Histopathol 16: 989-996. |
[81] |
Chisholm NC, Packard AR, Koss WA, et al. (2012) The Effects of Long-Term Treatment with Estradiol and Medroxyprogesterone Acetate on Tyrosine Hydroxylase Fibers and Neuron Number in the Medial Prefrontal Cortex of Aged Female Rats. Endocrinology 153: 4874-4882. doi: 10.1210/en.2012-1412
![]() |
[82] |
Kale P, Mohanty A, Patil A, et al. (2014) Estrogen modulates neural-immune interactions through intracellular signalling pathways and antioxidant enzyme activity in the spleen of middle-aged ovariectomized female rats. J Neuroimmunol 267: 7-15. doi: 10.1016/j.jneuroim.2013.11.003
![]() |
[83] |
Tang MX, Jacobs D, Stern Y, et al. (1996) Effect of oestrogen during menopause on risk and age at onset of Alzheimer's disease. Lancet 348: 429-432. doi: 10.1016/S0140-6736(96)03356-9
![]() |
[84] |
Jacome LF, Gautreaux C, Inagaki T, et al. (2010) Estradiol and ERβ agonists enhance recognition memory, and DPN, an ERβ agonist, alters brain monoamines. Neurobiol Learn Mem 94: 488-498. doi: 10.1016/j.nlm.2010.08.016
![]() |
[85] |
Gibbs RB (2010) Estrogen therapy and cognition: a review of the cholinergic hypothesis. Endocr Rev 31: 224-253. doi: 10.1210/er.2009-0036
![]() |
[86] |
Norbury R, Cutter WJ, Compton J, et al. (2003) The neuroprotective effects of estrogen on the aging brain. Exp Gerontol 38: 109-117. doi: 10.1016/S0531-5565(02)00166-3
![]() |
[87] | Paul V, Ekambaram P (2011) Involvement of nitric oxide in learning & memory processes. Indian J Med Res 133: 471-478. |
[88] |
Kopf SR, Benton RS, Kalfin R, et al. (2001) NO synthesis inhibition decreases cortical ACH release and impairs retention of conditioned response. Brain Res 894: 141-144. doi: 10.1016/S0006-8993(00)03148-6
![]() |
[89] |
Ghisletti S, Meda C, Maggi A, et al. (2005) 17 Beta-estradiol inhibits inflammatory gene expression by controlling NF-kappa B intracellular localization. Mol Cell Biol 25: 2957-2968. doi: 10.1128/MCB.25.8.2957-2968.2005
![]() |
[90] |
Walf AA, Paris JJ, Rhodes ME, et al. (2011) Divergent mechanisms for trophic actions of estrogens in the brain and peripheral tissues. Brain Res 1379: 119-136. doi: 10.1016/j.brainres.2010.11.081
![]() |
[91] |
Hasan W, Smith HJ, Ting AY, et al. (2005) Estrogen alters trkA and p75 neurotrophin receptor expression within sympathetic neurons. J Neurobiol 65: 192-204. doi: 10.1002/neu.20183
![]() |
[92] |
Arbogast LA, Hyde JF (2000) Estradiol attenuates the forskolin-induced increase in hypothalamic tyrosine hydroxylase activity. Neuroendocrinology 71: 219-227. doi: 10.1159/000054539
![]() |
[93] |
Kritzer MF, Kohama SG (1998) Ovarian hormones influence the morphology, distribution, and density of tyrosine hydroxylase immunoreactive axons in the dorsolateral prefrontal cortex of adult rhesus monkeys. J Comp Neurol 395: 1-17. doi: 10.1002/(SICI)1096-9861(19980525)395:1<1::AID-CNE1>3.0.CO;2-4
![]() |
[94] |
Babu GN, Vijayan E (1984) Hypothalamic tyrosine hydroxylase activity and plasma gonadotropin and prolactin levels in ovariectomized-steroid treated rats. Brain Res Bull 12: 555-558. doi: 10.1016/0361-9230(84)90171-0
![]() |
[95] |
Chisholm NC, Packard AR, Koss WA, et al. (2012) The effects of long-Term treatment with estradiol and medroxyprogesterone acetate on tyrosine hydroxylase fibers and neuron number in the medial prefrontal cortex of aged female rats. Endocrinology 153: 4874-4882. doi: 10.1210/en.2012-1412
![]() |
[96] |
Turcano P, Savica R (2020) Sex differences in movement disorders. Handb Clin Neurol 175: 275-282. doi: 10.1016/B978-0-444-64123-6.00019-9
![]() |
[97] |
Esterbauer H, Schaur RJ, Zollner H (1991) Chemistry and biochemistry of 4-hydroxynonenal, malonaldehyde and related aldehydes. Free Radic Biol Med 11: 81-128. doi: 10.1016/0891-5849(91)90192-6
![]() |
[98] |
Viveros MP, Arranz L, Hernanz A, et al. (2007) A model of premature aging in mice based on altered stress-related behavioural response and immunosenescence. Neuroimmunomodulation 14: 157-162. doi: 10.1159/000110640
![]() |
[99] |
Tian L, Cai Q, Bowen R, et al. (1995) Effects of caloric restriction on age-related oxidative modifications of macromolecules and lymphocyte proliferation in rats. Free Radic Biol Med 19: 859-865. doi: 10.1016/0891-5849(95)00090-K
![]() |
[100] |
Baeza I, Alvarado C, Alvarez P, et al. (2009) Improvement of leucocyte functions in ovariectomised aged rats after treatment with growth hormone, melatonin, oestrogens or phyto-oestrogens. J Reprod Immunol 80: 70-79. doi: 10.1016/j.jri.2009.02.002
![]() |
[101] |
Baeza I, De Castro NM, Giménez-Llort L, et al. (2010) Ovariectomy, a model of menopause in rodents, causes a premature aging of the nervous and immune systems. J Neuroimmunol 219: 90-99. doi: 10.1016/j.jneuroim.2009.12.008
![]() |
[102] |
Baeza I, Fdez-Tresguerres J, Ariznavarreta C, et al. (2010) Effects of growth hormone, melatonin, oestrogens and phytoestrogens on the oxidized glutathione (GSSG)/reduced glutathione (GSH) ratio and lipid peroxidation in aged ovariectomized rats. Biogerontology 11: 687-701. doi: 10.1007/s10522-010-9282-7
![]() |
[103] |
Vina J, Gambini J, Lopez-Grueso R, et al. (2011) Females live longer than males: role of oxidative stress. Curr Pharm Des 17: 3959-3965. doi: 10.2174/138161211798764942
![]() |
[104] |
Germain D (2016) Sirtuins and the Estrogen Receptor as Regulators of the Mammalian Mitochondrial UPR in Cancer and Aging. Adv Cancer Res 130: 211-256. doi: 10.1016/bs.acr.2016.01.004
![]() |
[105] |
Brann D, Raz L, Wang R, et al. (2012) Oestrogen signalling and neuroprotection in cerebral ischaemia. J Neuroendocrinol 24: 34-47. doi: 10.1111/j.1365-2826.2011.02185.x
![]() |
[106] |
Simpkins JW, Green PS, Gridley KE, et al. (1997) Role of estrogen replacement therapy in memory enhancement and the prevention of neuronal loss associated with Alzheimer's disease. Am J Med 103: 19S-25S. doi: 10.1016/S0002-9343(97)00260-X
![]() |
[107] | Shulman GI, Barrett EJ, Sherwin RS (2003) Integrated fuel metabolism. Ellenberg & Rifkin's diabetes mellitus NewYork: McGraw-Hill, 1-13. |
[108] | Shi J, Simpkins JW (1997) 17 beta-Estradiol modulation of glucose transporter 1 expression in blood-brain barrier. Am J Physiol 272: E1016-E1022. |
[109] |
Kostanyan A, Nazaryan A (1992) Rat brain glycolysis regulation by estradiol-17 beta. Biochim Biophys Acta 1133: 301-306. doi: 10.1016/0167-4889(92)90051-C
![]() |
[110] | Magistretti P (2008) Brain energy metabolism. Fundamental neuroscience San Diego: Academic, 271-296. |
[111] |
Hernández-R J (1992) Na+/K(+)-ATPase regulation by neurotransmitters. Neurochem Int 20: 1-10. doi: 10.1016/0197-0186(92)90119-C
![]() |
[112] |
Brinton RD (2008) Estrogen regulation of glucose metabolism and mitochondrial function: therapeutic implications for prevention of Alzheimer's disease. Adv Drug Deliv Rev 60: 1504-1511. doi: 10.1016/j.addr.2008.06.003
![]() |
[113] |
Kostanyan A, Nazaryan A (1992) Rat brain glycolysis regulation by estradiol-17 beta. Biochim Biophys Acta 1133: 301-306. doi: 10.1016/0167-4889(92)90051-C
![]() |
[114] |
Moorthy K, Yadav UC, Siddiqui MR, et al. (2004) Effect of estradiol and progesterone treatment on carbohydrate metabolizing enzymes in tissues of aging female rats. Biogerontology 5: 249-259. doi: 10.1023/B:BGEN.0000038026.89337.02
![]() |
[115] |
Rasgon NL, Silverman D, Siddarth P, et al. (2005) Estrogen use and brain metabolic change in postmenopausal women. Neurobiol Aging 26: 229-235. doi: 10.1016/j.neurobiolaging.2004.03.003
![]() |
[116] |
Monteiro R, Teixeira D, Calhau C (2014) Estrogen signaling in metabolic inflammation. Mediators Inflamm 2014: 615917. doi: 10.1155/2014/615917
![]() |
[117] |
Villa A, Rizzi N, Vegeto E, et al. (2015) Estrogen accelerates the resolution of inflammation in macrophagic cells. Sci Rep 5: 15224. doi: 10.1038/srep15224
![]() |
[118] |
Bagger YZ, Tankó LB, Alexandersen P, et al. (2005) Early postmenopausal hormone therapy may prevent cognitive impairment later in life. Menopause 12: 12-7. doi: 10.1097/00042192-200512010-00005
![]() |
[119] |
Maki PM (2006) Hormone therapy and cognitive function: is there a critical period for benefit? Neuroscience 138: 1027-1030. doi: 10.1016/j.neuroscience.2006.01.001
![]() |
[120] |
Keller JN, Germeyer A, Begley JG, et al. (1997) 17 Beta-estradiol attenuates oxidative impairment of synaptic Na+/K+-ATPase activity, glucose transport, and glutamate transport induced by amyloid beta-peptide and iron. J Neurosci Res 50: 522-530. doi: 10.1002/(SICI)1097-4547(19971115)50:4<522::AID-JNR3>3.0.CO;2-G
![]() |
[121] |
Kumar P, Kale RK, McLean P, et al. (2011) Protective effects of 17 β-estradiol on altered age related neuronal parameters in female rat brain. Neurosci Lett 502: 56-60. doi: 10.1016/j.neulet.2011.07.024
![]() |
[122] |
Shi C, Xu J (2008) Increased vulnerability of brain to estrogen withdrawal-induced mitochondrial dysfunction with aging. J Bioenerg Biomembr 40: 625-630. doi: 10.1007/s10863-008-9195-1
![]() |
[123] |
Wong-Riley MT (1989) Cytochrome oxidase: an endogenous metabolic marker for neuronal activity. Trends Neurosci 12: 94-101. doi: 10.1016/0166-2236(89)90165-3
![]() |
[124] |
Bettini E, Maggi A (1992) Estrogen induction of cytochrome c oxidase subunit III in rat hippocampus. J Neurochem 58: 1923-1929. doi: 10.1111/j.1471-4159.1992.tb10070.x
![]() |
[125] |
Soane L, Kahraman S, Kristian T, et al. (2007) Mechanisms of impaired mitochondrial energy metabolism in acute and chronic neurodegenerative disorders. J Neurosci Res 85: 3407-3415. doi: 10.1002/jnr.21498
![]() |
[126] |
Atamna H, Frey WH (2007) Mechanisms of mitochondrial dysfunction and energy deficiency in Alzheimer's disease. Mitochondrion 7: 297-310. doi: 10.1016/j.mito.2007.06.001
![]() |
Parameters | DC-DC Boost Converter Ⅰ | DC-DC Boost Converter Ⅱ |
Switching frequency fS | 25 kHz | 25 kHz |
Inductance L | 9.136 mH | 10.200 mH |
Capacitance C | 452 µF | 430 µF |
Voltage V | 6–12 V | 6–12 V |
Parameters | Voltage control loop | Current control loop |
Proportional Gain kP | 0.182 | 0.114 |
Integral Gain kI | 23.364 | 19 |
Time | 1-3 second | 3-5 second |
I1 (A) | 0.346 | 0.39 |
I2 (A) | 0.425 | 0.475 |
IL (A) | 0.771 | 0.864 |
Vdc1 (V) | 12.04 | 12 |
Vdc2 (V) | 12.01 | 11.975 |
VL (V) | 11.97 | 11.925 |
ΔI (%) current sharing differences | 10.25 | 9.84 |
Time | 1-3 second | 3-5 second |
I1 (A) | 0.385 | 0.43 |
I2 (A) | 0.385 | 0.43 |
IL (A) | 0.77 | 0.86 |
Vdc1 (V) | 12.04 | 12.01 |
Vdc2 (V) | 12.01 | 11.96 |
VL (V) | 11.96 | 11.92 |
ΔI (%) current sharing differences | 0 | 0 |
Time | 1-3 second | 3-5 second |
I1 (A) | 0.387 | 0.435 |
I2 (A) | 0.387 | 0.435 |
IL (A) | 0.774 | 0.87 |
Vdc1 (V) | 12.075 | 12.085 |
Vdc2 (V) | 12.036 | 12.04 |
VL (V) | 12 | 12 |
ΔI (%) current sharing differences | 0 | 0 |
Load resistance | 15.51 Ω | 13.804 Ω |
I1 (A) | 0.388 | 0.4355 |
I2 (A) | 0.388 | 0.4355 |
IL (A) | 0.777 | 0.871 |
Vestimated (V) | 11.951 | 11.905 |
Parameters | DC-DC Boost Converter Ⅰ | DC-DC Boost Converter Ⅱ |
Switching frequency fS | 25 kHz | 25 kHz |
Inductance L | 9.136 mH | 10.200 mH |
Capacitance C | 452 µF | 430 µF |
Voltage V | 6–12 V | 6–12 V |
Parameters | Voltage control loop | Current control loop |
Proportional Gain kP | 0.182 | 0.114 |
Integral Gain kI | 23.364 | 19 |
Time | 1-3 second | 3-5 second |
I1 (A) | 0.346 | 0.39 |
I2 (A) | 0.425 | 0.475 |
IL (A) | 0.771 | 0.864 |
Vdc1 (V) | 12.04 | 12 |
Vdc2 (V) | 12.01 | 11.975 |
VL (V) | 11.97 | 11.925 |
ΔI (%) current sharing differences | 10.25 | 9.84 |
Time | 1-3 second | 3-5 second |
I1 (A) | 0.385 | 0.43 |
I2 (A) | 0.385 | 0.43 |
IL (A) | 0.77 | 0.86 |
Vdc1 (V) | 12.04 | 12.01 |
Vdc2 (V) | 12.01 | 11.96 |
VL (V) | 11.96 | 11.92 |
ΔI (%) current sharing differences | 0 | 0 |
Time | 1-3 second | 3-5 second |
I1 (A) | 0.387 | 0.435 |
I2 (A) | 0.387 | 0.435 |
IL (A) | 0.774 | 0.87 |
Vdc1 (V) | 12.075 | 12.085 |
Vdc2 (V) | 12.036 | 12.04 |
VL (V) | 12 | 12 |
ΔI (%) current sharing differences | 0 | 0 |
Load resistance | 15.51 Ω | 13.804 Ω |
I1 (A) | 0.388 | 0.4355 |
I2 (A) | 0.388 | 0.4355 |
IL (A) | 0.777 | 0.871 |
Vestimated (V) | 11.951 | 11.905 |