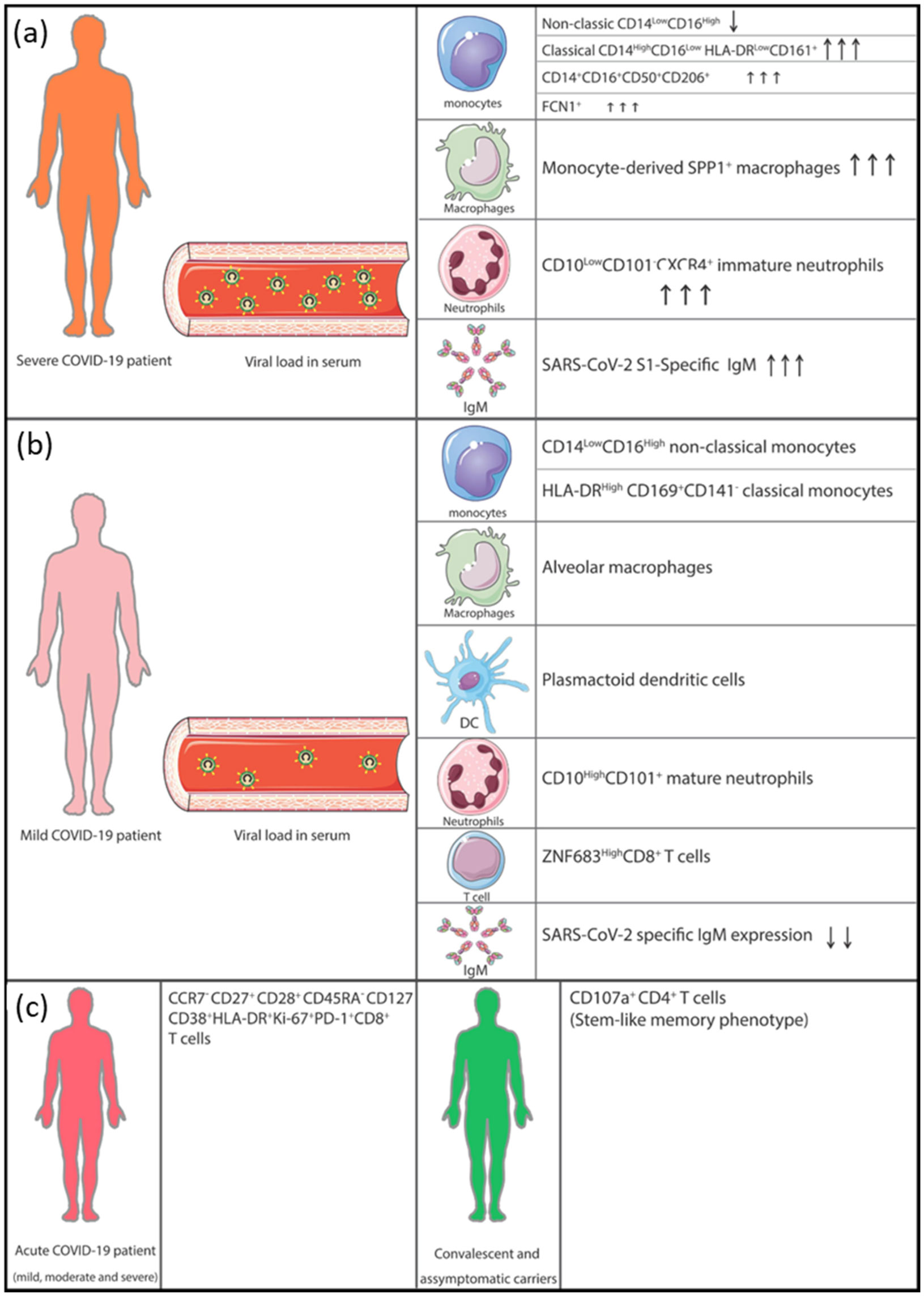
The ravaging pandemic caused by SAR-CoV-2, a member of β-coronaviruses, marks the end of the year 2019. Despite being identified and classified at the earliest stage, the virus records worldwide soaring transmissibility, morbidity, and mortality. Global data have shown the infection with SARS-CoV-2 to be severe among at least 15% of the infected; the aged and those with premorbid conditions like cancer, cardiovascular, and respiratory diseases. The highest prevalence and mortality are seen in the Americas, with African countries least affected. Severe respiratory distress and multiorgan failure are the usual findings in severe cases. A hyperinflammatory, fulminant, hypercytokinemia that is often further complicated by hypercoagulopathy and multiorgan failure has been reported extensively among severely infected patients. Scientists describe hyper-activated immune response mediated by macrophages secreting copious amounts of interleukin (IL)-6 forming the epicenter of cytokine storm (CS), thereby perpetuating signaling cascade through JAK/Kinase pathway that yields a hypercytokinemia. Researchers globally are exploring JAK/kinase inhibitors, immunomodulatory (immunosuppressive) therapy, cytokines, and cytokine receptor blockers for CS management. In which interestingly some of these agents possess antiviral activity. Here, we reviewed published studies with their respective outcome. However, a lot needs to be done to address the CS of COVID-19 to avert its fatal outcome.
Citation: Mansur Aliyu, Sayed-Hamidreza Mozhgani, Omid Kohandel Gargari, Mustapha Ahmed Yusuf, Ali Akbar Saboor-Yaraghi. The host immune responses to SARS-CoV-2 and therapeutic strategies in the treatment of COVID-19 cytokine storm[J]. AIMS Allergy and Immunology, 2021, 5(4): 240-258. doi: 10.3934/Allergy.2021018
[1] | Yu. A. Desheva, A. S. Mamontov, P. G. Nazarov . Contribution of antibody-dependent enhancement to the pathogenesis of coronavirus infections. AIMS Allergy and Immunology, 2020, 4(3): 50-59. doi: 10.3934/Allergy.2020005 |
[2] | Darrell O. Ricke . Antibodies and infected monocytes and macrophages in COVID-19 patients. AIMS Allergy and Immunology, 2022, 6(2): 64-70. doi: 10.3934/Allergy.2022007 |
[3] | Kamyar Nasiri, Zohreh Mortezania, Sanaz Oftadehbalani, Mohammad Khosousi Sani, Amin Daemi, Seyyedeh Touran Hosseini, Yusuf Döğüş, Zafer Yönden . Parasitology and Covid-19: epidemiological, immunological, pathological, and therapeutic aspects. AIMS Allergy and Immunology, 2023, 7(1): 92-103. doi: 10.3934/Allergy.2023007 |
[4] | DharmaSaranya Gurusamy, Vasuki Selvamurugesan, Swaminathan Kalyanasundaram, Shantaraman Kalyanaraman . Is COVID 19, a beginning of new entity called chronic viral systemic inflammatory syndrome?. AIMS Allergy and Immunology, 2021, 5(2): 127-134. doi: 10.3934/Allergy.2021010 |
[5] | Daniel Danladi Gaiya, Jonathan Danladi Gaiya, Richard Auta, Aliyu Muhammad, Bege Jonathan, Stella Kuyet Udu, Ekpa Emmanuel, Amina Shehu Bature . The journey so far with SARS-CoV-2 variants: Pathogenesis, immunity and treatments. AIMS Allergy and Immunology, 2023, 7(4): 222-250. doi: 10.3934/Allergy.2023016 |
[6] | Lia Tsverava, Nazibrola Chitadze, Gvantsa Chanturia, Merab Kekelidze, David Dzneladze, Paata Imnadze, Amiran Gamkrelidze, Vincenzo Lagani, Zaza Khuchua, Revaz Solomonia . Antibody profiling reveals gender differences in response to SARS-COVID-2 infection. AIMS Allergy and Immunology, 2022, 6(1): 6-13. doi: 10.3934/Allergy.2022002 |
[7] | Zinia Pervin, Anika Tasnim, Hasib Ahamed, Md Al Hasibuzzaman . Epigenetic regulation of the COVID-19 pathogenesis: its impact on the host immune response and disease progression. AIMS Allergy and Immunology, 2023, 7(1): 60-81. doi: 10.3934/Allergy.2023005 |
[8] | Ahmed Yaqinuddin, Ayesha Rahman Ambia, Tasnim Atef Elgazzar . Case fatalities due to COVID-19: Why there is a difference between the East and West?. AIMS Allergy and Immunology, 2021, 5(1): 56-63. doi: 10.3934/Allergy.2021005 |
[9] | Ryuta Muromoto, Kenji Oritani, Tadashi Matsuda . Tyk2-mediated homeostatic control by regulating the PGE2-PKA-IL-10 axis. AIMS Allergy and Immunology, 2021, 5(3): 175-183. doi: 10.3934/Allergy.2021013 |
[10] | Swathi Mukurala, Jahanavi Bandla, Swetha kappala . Angioedema-post COVID symptoms. AIMS Allergy and Immunology, 2023, 7(4): 273-280. doi: 10.3934/Allergy.2023018 |
The ravaging pandemic caused by SAR-CoV-2, a member of β-coronaviruses, marks the end of the year 2019. Despite being identified and classified at the earliest stage, the virus records worldwide soaring transmissibility, morbidity, and mortality. Global data have shown the infection with SARS-CoV-2 to be severe among at least 15% of the infected; the aged and those with premorbid conditions like cancer, cardiovascular, and respiratory diseases. The highest prevalence and mortality are seen in the Americas, with African countries least affected. Severe respiratory distress and multiorgan failure are the usual findings in severe cases. A hyperinflammatory, fulminant, hypercytokinemia that is often further complicated by hypercoagulopathy and multiorgan failure has been reported extensively among severely infected patients. Scientists describe hyper-activated immune response mediated by macrophages secreting copious amounts of interleukin (IL)-6 forming the epicenter of cytokine storm (CS), thereby perpetuating signaling cascade through JAK/Kinase pathway that yields a hypercytokinemia. Researchers globally are exploring JAK/kinase inhibitors, immunomodulatory (immunosuppressive) therapy, cytokines, and cytokine receptor blockers for CS management. In which interestingly some of these agents possess antiviral activity. Here, we reviewed published studies with their respective outcome. However, a lot needs to be done to address the CS of COVID-19 to avert its fatal outcome.
cytokine storm;
noble coronavirus pneumonia disease 2019;
severe acute respiratory syndrome coronavirus 2;
angiotensin-converting enzyme receptor 2;
transmembrane serine protease 2;
numb-associated kinases;
Janus kinase-signal transducer and activator of transcription;
nuclear factor-kappa beta;
interferon gamma;
tumor necrosis factor alpha;;
NLR (nod-like receptor) family pyrin domain containing 3
In December 2019, Wu and coworkers reported an index case of SARS-CoV-2 [1]. The World Health Organization (WHO) flag the noble coronavirus pneumonia disease 2019 (COVID-19) as a pandemic in the first quarter of 2020 [2]. The SARS-CoV-2 is an acute infection, transmitted from person to person through respiratory droplets [3]. Lymphopenia and ground-glass opacities on complete blood count and computed tomography (CT) respectively are the usual findings [4]. Morphologically, the SARS-CoV-2 is pleomorphic [5], enveloped, single-stranded RNA virus [6].
The SARS-CoV-2 has spikes (S, S1, S2, and receptor binding domain/RBD), envelope (E), membrane (M) and nucleocapsid (N) structural proteins encoded by RNA sequence. Two overlapping open reading frames (OFRs) encode its sixteen non-structural proteins (OFR1a and OFR1b) encoded by replicase/transcriptase genes [7],[8]. The S protein located on the surface is utilized in the viral entry; highly immunogenic and stands tall for vaccine design. However, biochemical mimicry with human proteins was reported in all the thirty-seven protein vaccine targets of SARS-CoV-2; this may explain autoimmunity as a compounding factor in COVID-19 [9].
Since the advent of COVID-19 clinicians manage cases using different classes of drugs which include antiviral agents, convalescent plasma, , anti-inflammatory agents, immunomodulatory drugs, monoclonal antibodies, corticosteroids, chloroquine and hydroxychloroquine to mention but a few [10]. These drugs were employed in different combinations with varying results. The infectivity and mortality of COVID-19 infection put global health care at distress, shutting down economies and wellbeing, never known before. However, the prospects of pharmacotherapy against SARS-COV2 are not within the scope of this paper.
The COVID-19 represents the worst disaster that hit the human race in recent times. The morbidity, mortality and financial crises know no bounds worldwide. The work of scientists and clinicians continues to be stretched through treatment uncertainties, vaccine development complexities and advancement of cytokine storm (CS) fatalities. In this review, we discussed the overview of the spectrum of immune responses, innate and specific to SARS-CoV-2, the pathophysiology and therapeutic strategies in the treatment of COVID-19 CS, which form the backbone of this paper, with references to ongoing clinical trials globally.
The SARS-CoV-2 viruses utilize their trimeric spike glycoprotein to bind the peptidase domain of angiotensin-converting enzyme receptor 2 (ACE2) avidly for onward viral attachment to gain entry into the host cells [11]. In addition to ACE2, SARS-CoV-2 S protein require priming by transmembrane serine protease 2 (TMPRSS2). An inhibitor of TMPRSS2 blocks SARS-CoV-2 infection of the pneumocytes [12]. SAR-CoV shares 88.6% homology of its receptor-binding domain with SARS-CoV-2 [8], but recorded low cross-reactivity of monoclonal antibodies of SAR-CoV against SARS-CoV-2. Similarly, anti-SAR-CoV monoclonal antibodies do not inhibit SARS-CoV-2 viral entry using the ACE2 [11].
Scientists report similar and varied findings regarding immune responses to COVID-19, which fit in the puzzle to provide a larger picture of the cumulative evidence of this discovered infectious disease. The role of non-specific immunity to SARS-CoV-2 is not clear-cut. Some researchers believe that BCG vaccination offers a protective role to COVID-19; globally, different countries enroll in clinical studies to verify BCG's putative effect. However, countries like Brazil prove the contrary, having a national BCG program for over half a century yet ranked among the worst countries hit by the COVID-19 [13].
Pathogenesis of SARS-CoV-2, like other respiratory viruses upon successful infection, specialized cell surface and cytosolic pattern-recognition factors of professional and non-professional antigen-presenting cells recognize SARS-CoV-2 specific antigens and activate adaptor proteins that subsequently activate transcription factors to facilitate the production of cytokines and chemokines, which aid recruitment of immune effector cells. The SARS-CoV-2 commonly infects alveolar macrophages, type I and II pneumocytes and induced secretion of CXCL1, CXCL5, interferon gamma-induced protein 10 (IP10/CXCL10), interleukin (IL)-6 and monocyte chemoattractant protein-1 (MCP-1/CCL2) [14],[15].
Hadjadj and coworkers reported low interferon (IFN)-α, deficient IFN-β expression with associated increase viral load in the peripheral blood of patients with severe COVID-19 [16]. Similarly, in another study macrophages in the bronchoalveolar lavage fluid of patients with severe COVID-19 lack expression of IFN-α [17]. Studies found a distinct immune cell population between mild and severe cases of COVID-19. In a single-cell RNA sequencing and viral track analysis on bronchoalveolar lavage fluid from mild and severe cases of COVID-19, researchers reported a good population of alveolar macrophages, plasmacytoid dendritic cells, ZNF683hi CD8+ T cells among the mild cases. However, NK cells, neutrophils, FCN1+ monocytes, and monocyte-derived SPP1+ macrophages, and naïve CCR7+ CD4+ T cells were prevalent among severe COVID-19 cases [17].
Investigators stage COVID-19 based on innate immune features they studied using flow cytometry and single-cell RNA sequencing of blood samples from COVID-19 cases. Severe COVID-19 is characterized by a low level of non-classic CD14LowCD16High monocytes and accumulation of the classical CD14HighCD16LowHLA-DRLowCD161+ monocytes, besides a vast number of CD10LowCD101−CXCR4+ immature neutrophils and copious secretion of calprotectin (S100A8/S100A9). Mild cases of COVID-19 recorded preservation of CD14lowCD16high non-classical monocytes, CD10highCD101+ mature neutrophils, and HLA-DRhigh CD169+ CD141− classical monocytes. They classify the high level of calprotectin, neutrophilia, and loss of non-classical monocytes as poor prognostic factors for COVID-19 [18].
Humeral and cell-mediated immunity to SARS-CoV-2 was documented in patients with COVID-19. Both anti-SARS-CoV-2 T cells and IgG are more pronounced against the S protein of the SARS-CoV-2 rather than membrane or nucleoprotein. Besides, anti-S IgG has displayed neutralizing activity against the SARS-CoV-2. Furthermore, antibody titers in convalescent patients vary significantly over a wide range. Some patients are devoid of immunoglobulin class switching and deficient in viral-neutralization; despite having T cell-specific immunity to SARS-CoV-2 S protein [19]. In a nutshell, the existence of the severe disease in the presence of active humoral and cell-mediated immunity entails that the immune system actively participates in the pathogenesis of COVID-19.
Humeral immune response forms a cardinal pillar in defense against viral diseases and forms a basis for diagnosis. Hence, antibody response in SARS-CoV-2 cannot be an exception. IgM and IgA specific to pathogens are detected in the second week of infection, while antigen-specific IgG appeared in the serum after the second week. The circulating S-specific CD4+ T cell polarization was found to be skewed towards T follicular helper cells, signifying their role in the humoral immune response [20]. Recently, scientists find the sensitivity of IgA and IgG enzyme-linked immunosorbent assays (ELISA) as 87.9% and 84.8%, respectively, among patients with polymerase chain reaction (PCR), confirm COVID-19. In the above-mentioned study, patients with severe COVID-19 express significantly more S1 antigen-specific antibodies than patients with moderate disease. All patients with moderate and severe COVID-19 attain seroconversion for both IgA and IgG after the second week of infection [21].
In another study, researchers employ ELISA, chemiluminescence microparticle immunoassays (CMIA), and colloidal-gold lateral-flow immunoassays (LFIA) to study SARS-CoV-2 S1 specific total antibody, IgM, and IgG in the serum of PCR confirm COVID-19 patients. Humeral immune response was detected from 6 days post-onset succeeded by a decrease in serum viral load. Sensitivities of the antigen-specific antibodies peak after two weeks post-onset. The researchers found no difference in sensitivity or specificity between the assay types used to detect SARS-CoV-2 S1 among the studied population for each specific antibody [22]. However, scientists reported persistent negative serological tests in PCR confirmed COVID-19 patients up to six weeks at the time of reporting. COVID-19 patients have a median seroconversion for total antibodies, IgM, and IgG as 11, 12, and 14 days respectively [23].
SARS-CoV-2 viral shedding among the mild patients is restricted to the respiratory system, and viral RNA disappears from their serum ten days post-onset; they also have lower antigen-specific IgM expression. In contrast, patients with severe COVID-19 have higher IgM expression and disseminated viral shedding in tissues for three to six weeks post-onset. IgM appears after the ninth-day post-onset and remains detectable in most patients. Antibodies to SARS-CoV cross-react with SARS-CoV-2 but not MERS. Patients with severe COVID-19 express more neutralizing antibodies than the milder cases, yet the neutralizing antibodies appear from the tenth day of onset in both scenarios. Convalescent sera from SARS-CoV-2 were found to block viral entry but not from SARS-CoV or MERS [8].
Coordination of a detectable humoral immune response with distinct class switching to yield antigen-specific IgG signifies the existence of cell-mediated immunity coordinated by CD4+ helper T cells. Nevertheless, cytotoxic CD8+ T cells are essential to viral immunity. From the discussions above, it is glaring that both arms of cell-mediated immunity are functional in COVID-19. A study describes the presence of viral-specific CD4+ and CD8+ T cells in COVID-19 patients and links the decline of the CD8+ subset with a poor prognosis. Besides rebound CD8+ T cell ratio after treatment correlates with better disease outcome, this phenomenon is deficient in patients that lack treatment response [24].
Scientists reported the existence of multifunctional SARS-CoV-2 specific CD107a+CD4+ T cells with stem-like memory phenotype at convalescence among mild and asymptomatic patients, also among close contacts with no detectable anti-SARS-CoV-2. In contrast, the acute phase SARS-CoV-2 CD38+HLA-DR+Ki-67+PD-1+CD8+ T cells are proactive cytotoxic lymphocytes also expressing CCR7−CD27+CD28+CD45RA−CD127−, found among acute, moderate, and severe COVID-19 patients [20].
Another study describes the presence of SARS-CoV-2 specific CD8+ T cells in all patients triage for intensive care admission but found SARS-CoV-2 specific CD4+ T cells in 80% of the same group of patients. They describe the presence of SARS-CoV-2 reactive T cells in 20% of the healthy population, possibly demonstrating cross-reactivity with other common coronaviruses. The T cell response is predominantly to the S protein of the SARS-CoV-2, mostly in the form of Th1, then Th2, and Th17 to a lesser extent [25]. Figure 1 describes the different immune cells that are peculiar to the clinical status of the COVID-19 patient's status.
Remy and colleagues analyzed peripheral blood mononuclear cells expression of cytokines using ELISpot of patients with PCR confirm COVID-19, while septic patients and other medical and surgical patients in the ICU that are not positive for SARS-CoV-2 serve as their control. They show that COVID-19 patients have remarkable immunosuppression from a reduced number of immune cells and functionally defective T cells and monocytes, which will explain the fatal outcome of COVID-19 rather than the acclaimed CS. They theorize the role of immunostimulatory therapy like IL-7 to possess a potent ameliorative effect in COVID-19 patients. Local cytokine assay within the lung tissue may discard this conclusion [26]. Another study reported normal monocyte counts among ICU patients with COVID-19 [4].
In Meta and colleagues' correspondence, they likened a late severe presentation of COVID-19 patients with secondary hemophagocytic lymphohistiocytosis (sHLH), a hyperinflammatory syndrome associated with hypercytokinemia and multiorgan failure usually initiated by viral infections [27]. CS is an unregulated and disseminated hyperinflammatory response (immune dysregulation) to pathogens from persistent activation of lymphocytes, monocytes, and macrophages, thereby yielding an endothelial dysfunction, vascular damage and metabolic dysregulation orchestrated by hypercytokinemia (pro-inflammatory)—establishing systemic inflammation setting in motion multiple organ failure that is often fatal [28],[29].
Zhang et al. outlined two CS pathogenesis mechanisms. First, patients prone to CS have genetic mutations in proteins responsible for clearing perforins released from cytotoxic cells (CD8+ T cells and NK cells). Hence, clearance of checkpoints by the cytotoxic target cells ensured, thereby favoring positive feedback on cytokines release by macrophages and T cells giving rise to CS. The loss of negative feedback mechanism on the release of the inflammatory response to infection, cancer, or drugs leads to CS in the second proposed mechanism, but what causes the feedback mechanism failure is unknown [30].
Yang and co-workers describe another pathway, therein proinflammatory cytokines like tumor necrosis factor-alpha (TNF-α), IFN-γ, IL-6 and IL-2 secreted by macrophages and T cells in response to SARS-CoV-2 infection activate NF-κβ and Janus kinase-signal transducer and activator of transcription (JAK/STAT) signaling pathways, thereby establishing a positive feedback mechanism of proinflammatory cytokines secretion and the subsequent establishment of CS [31].
IL-6 activations of JAK-STAT inflammatory pathway stands as the vital coordinator of the CS [28]. The binding of IL-6 to secretory (soluble) IL-6 receptor activates the trans-signaling of the IL-6 signaling pathway, which initiates a pro-inflammatory response. In contrast, IL-6 binding to membrane-bound IL-6r activates classical signaling that produced an anti-inflammatory response. Both classical and trans-signaling of IL-6 ensure the phosphorylation of tyrosine residues in the cytoplasmic part of the gp130-associated Janus kinases of the IL-6r, which binds transcription factor signal transducer and activator of transcription 3 (STAT3). STATs attached to the receptor subsequently translocate into the nucleus as STAT3-dimers being products of Janus kinases phosphorylation to effect STAT-specific gene expression [32]. STAT-3 induced the expression of IL-6, IL-17A, IL-17F, IL-22, IL-26, MCP-1, IL-10 and TGF-β, but downregulate the expression of IL-12, IFN-γ and TNF-α [33].
Studies conducted by Wang and colleagues pointed to the accumulation of SARS-CoV-2 infected macrophages hypersecreting IL-6 in the lung tissue as the pivotal cells behind the CS in COVID-19 [34],[35]. Zhang and coworkers use flow cytometry to describe a large population of monocytes with CD14+CD16+CD80+CD206+ phenotype in patients' peripheral blood with severe COVID-19 in the ICU, secreting copious amounts of IL-6, IL-10, and TNF-α. They hypothesize that these monocytes are the potent initiators of CS that usually resulted in acute respiratory distress syndrome (ARDS), coagulopathy, and death among COVID-19 patients [36].
Huang and coworkers use real-time PCR and next-generation sequencing to analyze patients' cytokines profile with confirmed COVID-19. They discover a cytokines profile for patients in the ICU to possess a high level of TNF-α, IL-2, IL-7, IL-10, granulocyte-macrophage colony-stimulating factor (GM-CSF/CSF2), CXCL10, MCP-1, and macrophage inflammatory protein 1 alpha (MIP-1α/CCL3) [37], a profile similar to that reported for sHLH [27]. Another study reported a direct correlation between COVID-19 disease severity with CXCL10, CCL7, and IL-1ra cytokine levels. Besides, CXCL10 and CCL7 increased levels correlate well with disease progression [38]. Severe cases present with an elevated level of C-reactive protein (CRP) and IL-6 [39].
A study of admitted patients with COVID-19 shows that patients with severe disease had markedly raised CRP and procalcitonin levels on admission but develop raised serum levels of IL-6, ferritin, and fibrin degradation product (FDP/D-dimer) while hospitalized. Interestingly, no COVID-19 predilection was reported among infants and children with immunosuppression, unlike obesity [40]. The indifference of COVID-19 among immunosuppressed and healthy children to attain a severe outcome signify that active immunity is not enough to curtail the infection.
Caricchio and coworkers have shown that COVID-19 CS is likened to monocyte activation syndrome (MAS) and HLH, but failed short of certifying the criteria for either of the two. Therefore, for diagnosis of CS in COVID-19 patients, scientists rely on laboratory findings that indicate inflammation, cell death, tissue damage, and prerenal electrolyte imbalance—above measured within twenty-four hours of the first week of admission before attaining CS. These criteria have a sensitivity of 85% and specificity of 80%; it helps the researchers to predict potential severe cases and those with the mortal outcome [35].
CS severely complicates SARS-CoV-2 infection; its diagnosis, prevention, and treatment form a vital pillar in treating COVID-19 patients. Many immunopharmacological therapies are on trial globally, including Janus kinase inhibitors, IL-6 inhibitors, IL-1r antagonists, and colchicine, which will be highlighted in this review. The pathogenesis of COVID-19, along with different immunopharmacological targets for CS, are depicted in Figure 2 below. Other immunotherapies on the lookout not covered in this review include corticosteroids, sphingo-1-phosphate receptor agonists, and type I/II IFN inhibitors [7]. However, despite the call for TNF-α inhibitors clinical study against COVID-19 CS, there are paucity of such clinical trials on clinicaltrials.gov. Nevertheless, phase 2 clinical trial of infliximab (TNF blocker) on severe COVID-19 patients had commenced in June 2020 in the USA [41].
A huge body of literature is piling up regarding clinical trials using monoclonal antibodies to treat SARS-CoV-2 associated CS. The majority of the clinical trials are taking place in the USA, France, Italy, Spain, China, Russia, Belgium, Greece, Denmark and others in that order [42]. As reviewed by Patel et al. a total of 65 clinical trials target IL-6 (inhibitors), which represent close to 50% of all monoclonal antibody clinical trials for COVID-19 CS, followed by clinical trials on non-monoclonal antibody inhibitors especially, Anakinra (IL-1ra inhibitor) with eight ongoing clinical trials and GM-CSF inhibitors having a record of seven trials. Other monoclonal antibody clinical trials target other proinflammatory cytokines, chemokines, receptors, adhesion molecules, complement products and growth factors [42]. Tocilizumab and sarilumab are among the monoclonal antibodies used in targeting IL-6 in COVID-19 CS [43].
Tocilizumab is a humanized monoclonal antibody that binds and inhibits both secreted and membrane-bound IL-6, also inhibits JAK-STAT or MAPK/NF-κβ-IL-6 signaling pathway. FDA licensed tocilizumab for stage II clinical trials of COVID-19 [44]. Syam and colleagues reported a case of a diagnosed patient of COVID-19 with an elevated level of IL-6 and hyperferritinemia which was treated effectively with a course of tocilizumab [45]. Suresh and coworkers reported on case series in Pennsylvania of three middle-aged females; two out of the three women were intubated. All the three have a clinical and laboratory diagnosis of COVID-19 CS, thereof relieved from CS after receiving two doses of intravenous tocilizumab [46].
In another case report, a 58-year old patient with ulcerative colitis, diagnosed with clinical, laboratory and radiological diagnosis of COVID-19 did well on standard care and remdesivir, which clear the viral load. Nevertheless, later deteriorated with elevated IL-6 level, raised CRP and fever. However, upon the institution of tocilizumab and other supportive therapy, the patient recorded an improvement in lung function and general stabilization [47]. Other case reports documented promising results with tocilizumab on COVID-19 CS therapy [48],[49].
In a small sample size study report, patients with severe COVID-19 were treated with two doses of tocilizumab in addition to standard-of-care—hydroxychloroquine, oseltamivir, and azithromycin or moxifloxacin. Patients show remarkable improvement in clinical and laboratory parameters (CS amelioration) [50]. A retrospective study of severe COVID-19 patients placed on tocilizumab and intubation did not report a superior outcome compared to those who received an interleukin-1 inhibitor (anakinra), probably compounded by the critical nature of the patients [51]. Ramiro and colleagues study the effect of intravenous tocilizumab with or without methylprednisolone among severe COVID-19 patients with CS in the Netherlands. Patients on tocilizumab, methylprednisolone and standard-of-care reported significantly less invasive mechanical ventilation and recorded less mortality than the control group [52].
As stated above the SARS-CoV-2 CS is characterized by hypercytokinemia and fulminant inflammation in which the JAK-STAT signaling pathway plays a pivotal role. Hence, scientists view JAK-STAT signaling inhibitors like baricitinib, ruxolitinib, and fedratinib as essential biologics in managing CS. Among the three JAK/STAT inhibitors mentioned, baricitinib has better dosing and milder side effects [53]. The entrance of SARS-CoV-2 needs numb-associated kinases (NAK): adaptor complex protein 2 (AP2)-associated protein kinase (AAK1) and cyclin G-associated kinase (GAK), which regulated the receptor-mediated cell entry of the SARS-CoV-2. Besides, ruxolitinib blocks AAK1 and baricitinib blocks, both AAK1 and GAK [53].
Recently, Cantini and coworkers undertake a pilot study on patients with clinical and laboratory-confirmed diagnoses of mild to moderate COVID-19 in Alessandria, Italy. Patients received baricitinib with lopinavir, ritonavir, and hydroxychloroquine. At the same time, the control group received all treatments except baricitinib. At the end of two weeks, the baricitinib-treated group shows significant improvement in clinical and laboratory parameters being considered, with no adverse effect reported [54]. The study excludes severe cases, the category that experiences CS. However, the ability of baricitinib to inhibit JAK1/JAK2 non-selectively will be beneficial to the excluded group. Another more robust baricitinib randomized control trials commence May 7, 2020 in the United Kingdom [55], we hope to see a desirable outcomes from this study in the future.
Ruxolitinib is another JAK1/JAK2 inhibitor licensed for psoriasis, myelofibrosis, and rheumatoid arthritis by the Food and Drug Administration (FDA). Likewise, ruxolitinib inhibits NAK, which the SARS-CoV-2 uses for endocytosis and replication [56]. A multicenter, single blind, randomized controlled trial was undertaken in China among adult patients with a confirmed severe COVID-19 diagnosis. The treatment group received ruxolitinib with standard-of-care for 14 days, while the control group received standard-of-care with vitamin C. The treatment group shows rapid clinical improvement, and the majority shows chest CT improvement, lymphopenia recovery, with no mortality. The control group recorded 14.3% mortality; forty-four out of the forty-eight analyzed cytokines declined except CCL3, G-CSF, IFN-α2, and IL-1α. However, all cytokines expression were downregulated among the treatment group. Moreover, the levels of IL-6, nerve growth factor (NGF)-β, IL-12 (p40), macrophage migration inhibitory factor (MIF), CCL3, CCL4, and vascular endothelial growth factor (VEGF) were significantly lowered among the treatment group than the control group [57].
As discussed above, pro-inflammatory cytokines drive the pathogenesis of CS; hence IL-1r blockade is thoughtful. The Anakinra is a recombinant IL-1r antagonist that down-regulates pro-inflammatory cytokines like IL-1α and IL-1β through the blockade of the Interleukin-1 receptor [58]. A retrospective cohort study among moderate and severe COVID-19 patients outside ICU with CS syndrome was conducted in Milan, Italy, to assess anakinra's effect. The above patients received hydroxychloroquine, lopinavir, and ritonavir as standard-of-care, while the study group received additional high dose anakinra. They reported a decline of CRP and ferritin, a decreased likelihood to use invasive ventilation, and increase survival among the high-dose anakinra study group. No significant difference was observed for bacteremia between the study and control group. Overall low-dose anakinra has no associated health benefits in this study [59].
The robust immunomodulatory, anti-inflammatory and antiviral properties of colchicine in COVID-19 have been described. Besides, FDA licensed colchicine for the treatment of gout [60]. Presently, clinicaltrials.gov registered twenty-four clinical trials of colchicine therapy in SARS-CoV-2 infection [61]. A matched cohort study of confirmed severe COVID-19 patients was conducted in the USA. The study group received colchicine in addition to standard of care, while the control group received standard of care, which consists of hydroxychloroquine, azithromycin, remdesivir, and tocilizumab—for those with a diagnosis of the CS. At two weeks, no significant difference in outcome was observed between the study and control groups. At the end of the fourth week, patients in the study group were five times more likely to be discharged from the hospital than controls, and three mortalities occur in the study group compared to eleven among the control group [62]. Tocilizumab might have an additive effect when combining with colchicine, but extensive studies are needed to make such a conclusion.
Mesenchymal stem cells (MSCs), being adult stem cells, are sourced from bone marrow, umbilical code tissue, or fat; they are self-renewing capable of differentiating into different cell types. MSCs are immunomodulatory and anti-inflammatory capable of dampening activated T cells and macrophages with resultant induction of regulatory phenotypes. MSCs also have a vital role in controlling CS through the inhibition of pro-inflammatory cytokines secretions [7]. Of note, MSCs inhibit T cell proliferation and differentiation, induce T cells apoptosis and induce production of IL-10, which cumulatively block proinflammatory response. While MSCs when co-cultured with macrophages they induce their differentiation to M2 phenotypes that express a huge amount of IL-10. Together, the above immunomodulatory, mechanisms were harnessed for the treatment of COVID-19 CS targeting T cells and macrophages, which are the key cells behind the storm. Hence, FDA has approved the utilization of MSCs in the treatment of COVID-19 CS [63]. Reports from experimental and phase 1 clinical trials have shown promising results with MSCs therapy in COVID-19 CS [64]. At present, 78 clinical trials are registered at clinicaltrials.gov with three trials already at phase III [65].
Corticosteroids are ubiquitous agents discovered almost a century ago and have been widely used, their immunosuppressive properties are especially put to use in so many disease conditions, however, it is important to always bear in mind the spectrum of their adverse effects, which are not discussed here [66]. Hydrocortisone, prednisolone and dexamethasone are readily available as over-the-counter glucocorticoids in most countries globally. Dexamethasone in addition to standard-of-care was shown to reduce mortality by 33% in the UK among COVID-19 patients on ventilators when compared to patients that receive standard-of-care only [67]. As Ramiro and co-workers reported in their prospective study, patients on parenteral methylprednisolone with or without tocilizumab in addition to standard-of-care were shown to have significantly lower mechanical ventilator use and lower hospital death compared to controls who are on the standard-of-care [68]. Quite a handful of clinical trials were at various stages in developed countries consistently reporting significant result on glucocorticoid's role in the management of COVID-19 CS [69]. Despite the promising results of glucocorticoids in COVID-19 management, alarming complications are being reported from secondary mucormycosis infection that is fatal [70]–[73].
Intravenous immunoglobulins (IVIg) were initially employed empirically in the treatment of moderate to severe COVID-19. The IVIg is composed primarily of IgG pooled from thousands of healthy donor's plasma [74]. The immunoglobulins here function by inhibiting activation of proinflammatory cells; blocking the expression of proinflammatory cytokines and complement, facilitating the expression of anti-inflammatory cytokines, tilting Th1/Th2 balance in favor of Th2. The IVIg have recorded some promising results in the outbreak of severe viral diseases recently, also COVID-19 [75]. Therefore, IVIg here is principally employed to shut down hyper-inflammatory CS [74],[76]. In a retrospective multi-center study in China, early administration of IVIg was shown to improve the outcome of the severely infected COVID-19 patients, in which CS provide the target for the IVIg [77].
The classes of drugs employed in the treatment of COVID-19 associated CS each has their limitations, which needed to be taken into consideration when using it. Another major limitation is in the presentation of CS, which is present among severe cases of COVID-19, if compounded by premorbid conditions among which mortality is high, knowing for real the efficacy of CS treatment will be impossible. As such, one will not understand the actual outcome. We presented the overview of CS management and the huge number of clinical trials going on globally, we expect these trials to translate into effective management of COVID-19 CS, especially with promising preliminary results obtained. A combination of these treatment modalities may likely provide a better outcome, as lower doses may have lower adverse effect while yielding combine additive beneficial effect. Calabrese has reviewed the prospects of immunotherapy in COVID-19 CS [78].
Patients with severe COVID-19 infection from SARS-CoV-2 are prone to cytokine storm, a fulminant hypercytokinemia that yields hyperinflammatory systemic response resulting in multiorgan failure. Several immunomodulatory anti-inflammatory pharmacological agents are under clinical trial globally to curtail this menace. However, early diagnosis and treatment of cases are of paramount significance.
[1] |
Wu F, Zhao S, Yu B, et al. (2020) A new coronavirus associated with human respiratory disease in China. Nature 579: 265-269. doi: 10.1038/s41586-020-2008-3
![]() |
[2] | WHO Rolling Updates on Coronavirus Disease (COVID-19). WHO, 2020 Available from: https://www.who.int/emergencies/diseases/novel-coronavirus-2019/events-as-they-happen. |
[3] |
Li Q, Guan X, Wu P, et al. (2020) Early transmission dynamics in Wuhan, China, of novel coronavirus-infected pneumonia. N Engl J Med 382: 1199-1207. doi: 10.1056/NEJMoa2001316
![]() |
[4] |
Wang D, Hu B, Hu C, et al. (2020) Clinical characteristics of 138 hospitalized patients with 2019 novel coronavirus-infected pneumonia in Wuhan, China. JAMA 323: 1061-1069. doi: 10.1001/jama.2020.1585
![]() |
[5] |
Caly L, Druce J, Roberts J, et al. (2020) Isolation and rapid sharing of the 2019 novel coronavirus (SARS-CoV-2) from the first patient diagnosed with COVID-19 in Australia. Med J Australia 212: 459-462. doi: 10.5694/mja2.50569
![]() |
[6] |
Chan JFW, Kok KH, Zhu Z, et al. (2020) Genomic characterization of the 2019 novel human-pathogenic coronavirus isolated from a patient with atypical pneumonia after visiting Wuhan. Emerg Microbes Infect 9: 221-236. doi: 10.1080/22221751.2020.1719902
![]() |
[7] |
Ye Q, Wang B, Mao J (2020) The pathogenesis and treatment of the “Cytokine Storm” in COVID-19. J Infection 80: 607-613. doi: 10.1016/j.jinf.2020.03.037
![]() |
[8] |
Wang Y, Zhang L, Sang L, et al. (2020) Kinetics of viral load and antibody response in relation to COVID-19 severity. J Clin Invest 130: 5235-5244. doi: 10.1172/JCI138759
![]() |
[9] |
Lyons-Weiler J (2020) Pathogenic priming likely contributes to serious and critical illness and mortality in COVID-19 via autoimmunity. J Transl Autoimmun 3: 100051-100051. doi: 10.1016/j.jtauto.2020.100051
![]() |
[10] |
Stasi C, Fallani S, Voller F, et al. (2020) Treatment for COVID-19: An overview. Eur J Pharmacol 889: 173644. doi: 10.1016/j.ejphar.2020.173644
![]() |
[11] |
Wrapp D, Wang N, Corbett KS, et al. (2020) Cryo-EM structure of the 2019-nCoV spike in the prefusion conformation. Science 367: 1260-1263. doi: 10.1126/science.abb2507
![]() |
[12] |
Hoffmann M, Kleine-Weber H, Schroeder S, et al. (2020) SARS-CoV-2 cell entry depends on ACE2 and TMPRSS2 and is blocked by a clinically proven protease inhibitor. Cell 181: 271-280. doi: 10.1016/j.cell.2020.02.052
![]() |
[13] | Charoenlap S, Piromsopa K, Charoenlap C (2020) Potential role of Bacillus Calmette-Guérin (BCG) vaccination in COVID-19 pandemic mortality: Epidemiological and Immunological aspects. Asian Pac J Allergy 38: 150-161. |
[14] |
García LF (2020) Immune response, inflammation, and the clinical spectrum of COVID-19. Front Immunol 11: 1441. doi: 10.3389/fimmu.2020.01441
![]() |
[15] |
Mortaz E, Tabarsi P, Varahram M, et al. (2020) The immune response and immunopathology of COVID-19. Front Immunol 11: 2037. doi: 10.3389/fimmu.2020.02037
![]() |
[16] |
Hadjadj J, Yatim N, Barnabei L, et al. (2020) Impaired type I interferon activity and inflammatory responses in severe COVID-19 patients. Science 369: 718-724. doi: 10.1126/science.abc6027
![]() |
[17] |
Bost P, Giladi A, Liu Y, et al. (2020) Host-viral infection maps reveal signatures of severe COVID-19 patients. Cell 181: 1475-1488. doi: 10.1016/j.cell.2020.05.006
![]() |
[18] |
Silvin A, Chapuis N, Dunsmore G, et al. (2020) Elevated calprotectin and abnormal myeloid cell subsets discriminate severe from mild COVID-19. Cell 182: 1401-1418. doi: 10.1016/j.cell.2020.08.002
![]() |
[19] |
Ni L, Ye F, Cheng ML, et al. (2020) Detection of SARS-CoV-2-specific humoral and cellular immunity in COVID-19 convalescent individuals. Immunity 52: 971-977. doi: 10.1016/j.immuni.2020.04.023
![]() |
[20] |
Sekine T, Perez-Potti A, Rivera-Ballesteros O, et al. (2020) Robust T cell immunity in convalescent individuals with asymptomatic or mild COVID-19. Cell 183: 158-168. doi: 10.1016/j.cell.2020.08.017
![]() |
[21] |
Kowitdamrong E, Puthanakit T, Jantarabenjakul W, et al. (2020) Antibody responses to SARS-CoV-2 in patients with differing severities of coronavirus disease 2019. PloS One 15: e0240502. doi: 10.1371/journal.pone.0240502
![]() |
[22] |
Lou B, Li TD, Zheng SF, et al. (2020) Serology characteristics of SARS-CoV-2 infection since exposure and post symptom onset. Eur Respir J 56: 2000763. doi: 10.1183/13993003.00763-2020
![]() |
[23] |
Zhao J, Yuan Q, Wang H, et al. (2020) Antibody responses to SARS-CoV-2 in patients of novel coronavirus disease 2019. Clin Infect Dis 71: 2027-2034. doi: 10.1093/cid/ciaa344
![]() |
[24] |
Wang F, Nie J, Wang H, et al. (2020) Characteristics of peripheral lymphocyte subset alteration in COVID-19 pneumonia. J Infect Dis 221: 1762-1769. doi: 10.1093/infdis/jiaa150
![]() |
[25] |
Weiskopf D, Schmitz KS, Raadsen MP, et al. (2020) Phenotype and kinetics of SARS-CoV-2-specific T cells in COVID-19 patients with acute respiratory distress syndrome. Sci Immunol 5: eabd2071. doi: 10.1126/sciimmunol.abd2071
![]() |
[26] |
Remy KE, Mazer M, Striker DA, et al. (2020) Severe immunosuppression and not a cytokine storm characterizes COVID-19 infections. JCI Insight 5: e140329. doi: 10.1172/jci.insight.140329
![]() |
[27] |
Mehta P, McAuley DF, Brown M, et al. (2020) COVID-19: consider cytokine storm syndromes and immunosuppression. Lancet 395: 1033-1034. doi: 10.1016/S0140-6736(20)30628-0
![]() |
[28] |
Bhaskar S, Sinha A, Banach M, et al. (2020) Cytokine storm in COVID-19-immunopathological mechanisms, clinical considerations, and therapeutic approaches: The reprogram consortium position paper. Front Immunol 11: 1648. doi: 10.3389/fimmu.2020.01648
![]() |
[29] |
Chen LYC, Hoiland RL, Stukas S, et al. (2020) Confronting the controversy: interleukin-6 and the COVID-19 cytokine storm syndrome. Eur Respir J 56: 2003006. doi: 10.1183/13993003.03006-2020
![]() |
[30] | Zhang D, Chen S (2020) Cytokine storms caused by novel coronavirus 2019 and treatment for cardiac injury. Eur Rev Med Pharmacol Sci 24: 12527-12535. |
[31] |
Yang L, Xie X, Tu Z, et al. (2021) The signal pathways and treatment of cytokine storm in COVID-19. Signal Transduct Target Ther 6: 255. doi: 10.1038/s41392-021-00679-0
![]() |
[32] |
Reeh H, Rudolph N, Billing U, et al. (2019) Response to IL-6 trans- and IL-6 classic signalling is determined by the ratio of the IL-6 receptor α to gp130 expression: fusing experimental insights and dynamic modelling. Cell Commun Signal 17: 46. doi: 10.1186/s12964-019-0356-0
![]() |
[33] |
Mogensen TH (2019) IRF and STAT transcription factors—From basic biology to roles in infection, protective immunity, and primary immunodeficiencies. Front Immunol 9: 3047. doi: 10.3389/fimmu.2018.03047
![]() |
[34] |
Wang C, Xie J, Zhao L, et al. (2020) Alveolar macrophage dysfunction and cytokine storm in the pathogenesis of two severe COVID-19 patients. EBioMedicine 57: 102833. doi: 10.1016/j.ebiom.2020.102833
![]() |
[35] |
Caricchio R, Gallucci M, Dass C, et al. (2020) Preliminary predictive criteria for COVID-19 cytokine storm. Ann Rheum Dis 80: 88-95. doi: 10.1136/annrheumdis-2020-218323
![]() |
[36] | Zhang D, Guo R, Lei L, et al. (2020) COVID-19 infection induces readily detectable morphologic and inflammation-related phenotypic changes in peripheral blood monocytes. J Leukocyte Biol 2020: 1-10. |
[37] |
Huang C, Wang Y, Li X, et al. (2020) Clinical features of patients infected with 2019 novel coronavirus in Wuhan, China. Lancet 395: 497-506. doi: 10.1016/S0140-6736(20)30183-5
![]() |
[38] |
Yang Y, Shen C, Li J, et al. (2020) Plasma IP-10 and MCP-3 levels are highly associated with disease severity and predict the progression of COVID-19. J Allergy Clin Immun 146: 119-127. doi: 10.1016/j.jaci.2020.04.027
![]() |
[39] |
Quartuccio L, Sonaglia A, McGonagle D, et al. (2020) Profiling COVID-19 pneumonia progressing into the cytokine storm syndrome: Results from a single Italian Centre study on tocilizumab versus standard of care. J Clin Virol 129: 104444. doi: 10.1016/j.jcv.2020.104444
![]() |
[40] |
Zachariah P, Johnson CL, Halabi KC, et al. (2020) Epidemiology, clinical features, and disease severity in patients with coronavirus disease 2019 (COVID-19) in a children's hospital in New York City, New York. JAMA Pediatr 174: e202430-e202430. doi: 10.1001/jamapediatrics.2020.2430
![]() |
[41] | Clinicaltrials.gov (2020) A Phase 2 Trial of Infliximab in Coronavirus Disease 2019 (COVID-19) United States National Library of Medicine, Available from: https://clinicaltrials.gov/ct2/show/NCT04425538?term=TNF-%CE%B1+inhibitors&cond=COVID&draw=2&rank=1. |
[42] |
Patel S, Saxena B, Mehta P (2021) Recent updates in the clinical trials of therapeutic monoclonal antibodies targeting cytokine storm for the management of COVID-19. Heliyon 7: e06158. doi: 10.1016/j.heliyon.2021.e06158
![]() |
[43] |
Sinha P, Matthay MA, Calfee CS (2020) Is a “cytokine storm” relevant to COVID-19? JAMA Intern Med 180: 1152-1154. doi: 10.1001/jamainternmed.2020.3313
![]() |
[44] |
Saha A, Sharma AR, Bhattacharya M, et al. (2020) Tocilizumab: A therapeutic option for the treatment of cytokine storm syndrome in COVID-19. Arch Med Res 51: 595-597. doi: 10.1016/j.arcmed.2020.05.009
![]() |
[45] | Syam AF, Pitoyo CW, Suhendro S, et al. (2021) Tocilizumab as a treatment for “cytokine storm syndrome” in COVID-19: A case report. Acta Med Indones 53: 194-201. |
[46] | Suresh K, Figart M, Formeck S, et al. (2021) Tocilizumab for the treatment of COVID-19-induced cytokine storm and acute respiratory distress syndrome: A case series from a rural level 1 Trauma Center in Western Pennsylvania. J Investig Med High Impact Case Rep 9: 1-5. |
[47] |
Kornitzky FW, Langen HJ, Held M (2021) Treatment of a patient with a pronounced cytokine storm in severe COVID-19 pneumonia using a hemoadsorption in combination with the administration of tocilizumab. Pneumologie 75: 644-650. doi: 10.1055/a-1458-4080
![]() |
[48] |
Farooqi F, Dhawan N, Morgan R, et al. (2020) Treatment of severe COVID-19 with tocilizumab mitigates cytokine storm and averts mechanical ventilation during acute respiratory distress: A case report and literature review. Trop Med Infect Dis 5: 112. doi: 10.3390/tropicalmed5030112
![]() |
[49] |
Chitturi KR, Thacker S, Al-Saadi MA, et al. (2020) Successful treatment of acute heart failure in COVID-19-induced cytokine storm with tocilizumab: a case report. Eur Heart J Case Rep 4: 1-6. doi: 10.1093/ehjcr/ytaa188
![]() |
[50] |
Borku Uysal B, Ikitimur H, Yavuzer S, et al. (2020) Tocilizumab challenge: A series of cytokine storm therapy experiences in hospitalized COVID-19 pneumonia patients. J Med Virol 92: 2648-2656. doi: 10.1002/jmv.26111
![]() |
[51] |
Langer-Gould A, Smith JB, Gonzales EG, et al. (2020) Early identification of COVID-19 cytokine storm and treatment with anakinra or tocilizumab. Int J Infect Dis 99: 291-297. doi: 10.1016/j.ijid.2020.07.081
![]() |
[52] |
Ramiro S, Mostard RLM, Magro-Checa C, et al. (2020) Historically controlled comparison of glucocorticoids with or without tocilizumab versus supportive care only in patients with COVID-19-associated cytokine storm syndrome: results of the CHIC study. Ann Rheum Dis 79: 1143-1151. doi: 10.1136/annrheumdis-2020-218479
![]() |
[53] |
Stebbing J, Phelan A, Griffin I, et al. (2020) COVID-19: combining antiviral and anti-inflammatory treatments. Lancet Infect Dis 20: 400-402. doi: 10.1016/S1473-3099(20)30132-8
![]() |
[54] |
Cantini F, Niccoli L, Matarrese D, et al. (2020) Baricitinib therapy in COVID-19: A pilot study on safety and clinical impact. J Infection 81: 318-356. doi: 10.1016/j.jinf.2020.04.017
![]() |
[55] |
Kulkarni S, Fisk M, Kostapanos M, et al. (2020) Repurposed immunomodulatory drugs for Covid-19 in pre-ICu patients—mulTi-Arm therapeutic study in pre-ICu patients admitted with Covid-19—repurposed drugs (TACTIC-R): A structured summary of a study protocol for a randomised controlled trial. Trials 21: 626. doi: 10.1186/s13063-020-04535-4
![]() |
[56] |
Convertino I, Tuccori M, Ferraro S, et al. (2020) Exploring pharmacological approaches for managing cytokine storm associated with pneumonia and acute respiratory distress syndrome in COVID-19 patients. Crit Care 24: 331. doi: 10.1186/s13054-020-03020-3
![]() |
[57] |
Cao Y, Wei J, Zou L, et al. (2020) Ruxolitinib in treatment of severe coronavirus disease 2019 (COVID-19): A multicenter, single-blind, randomized controlled trial. J Allergy Clin Immun 146: 137-146. doi: 10.1016/j.jaci.2020.05.019
![]() |
[58] | Cavalli G, Dinarello CA (2015) Treating rheumatological diseases and co-morbidities with interleukin-1 blocking therapies. Rheumatology 54: 2134-2144. |
[59] |
Cavalli G, De Luca G, Campochiaro C, et al. (2020) Interleukin-1 blockade with high-dose anakinra in patients with COVID-19, acute respiratory distress syndrome, and hyperinflammation: a retrospective cohort study. Lancet Rheumatol 2: e325-e331. doi: 10.1016/S2665-9913(20)30127-2
![]() |
[60] |
Schlesinger N, Firestein BL, Brunetti L (2020) Colchicine in COVID-19: an old drug, new use. Curr Pharmacol Rep 6: 137-145. doi: 10.1007/s40495-020-00225-6
![]() |
[61] | Clinicaltrials.gov (2020) Colchicine and COVID-19 Clinical Trials Search United States National Library of Medicine, Available from: https://clinicaltrials.gov/ct2/results?cond=COVID&term=colchicine&cntry=&state=&city=&dist=. |
[62] |
Brunetti L, Diawara O, Tsai A, et al. (2020) Colchicine to weather the cytokine storm in hospitalized patients with COVID-19. J Clin Med 9: 2961. doi: 10.3390/jcm9092961
![]() |
[63] |
Jeyaraman M, John A, Koshy S, et al. (2021) Fostering mesenchymal stem cell therapy to halt cytokine storm in COVID-19. BBA-Mol Basis Diss 1867: 166014-166014. doi: 10.1016/j.bbadis.2020.166014
![]() |
[64] |
Farkhad NK, Reihani H, Moghadam AA, et al. (2021) Are mesenchymal stem cells able to manage cytokine storm in COVID-19 patients? A review of recent studies. Regen Ther 18: 152-160. doi: 10.1016/j.reth.2021.05.007
![]() |
[65] | Clinicaltrials.gov (2020) Stem Cells and COVID-19 Clinical Trials Search United States National Library of Medicine, Available from: https://clinicaltrials.gov/ct2/results?cond=COVID-19&term=stem+cell&cntry=&state=&city=&dist=. |
[66] |
Kapugi M, Cunningham K (2019) Corticosteroids. Orthop Nurs 38: 336-339. doi: 10.1097/NOR.0000000000000595
![]() |
[67] |
Deng CX (2020) Glucocorticoids save lives in COVID-19 patients. Int J Biol Sci 16: 2477-2478. doi: 10.7150/ijbs.49125
![]() |
[68] |
Ramiro S, Mostard RLM, Magro-Checa C, et al. (2020) Historically controlled comparison of glucocorticoids with or without tocilizumab versus supportive care only in patients with COVID-19-associated cytokine storm syndrome: results of the CHIC study. Ann Rheum Dis 79: 1143-1151. doi: 10.1136/annrheumdis-2020-218479
![]() |
[69] |
Sharun K, Tiwari R, Dhama J, et al. (2020) Dexamethasone to combat cytokine storm in COVID-19: Clinical trials and preliminary evidence. Int J Surg 82: 179-181. doi: 10.1016/j.ijsu.2020.08.038
![]() |
[70] |
Ahmadikia K, Hashemi SJ, Khodavaisy S, et al. (2021) The double-edged sword of systemic corticosteroid therapy in viral pneumonia: A case report and comparative review of influenza-associated mucormycosis versus COVID-19 associated mucormycosis. Mycoses 64: 798-808. doi: 10.1111/myc.13256
![]() |
[71] |
Pakdel F, Ahmadikia K, Salehi M, et al. (2021) Mucormycosis in patients with COVID-19: A cross-sectional descriptive multicentre study from Iran. Mycoses 64: 1238-1252. doi: 10.1111/myc.13334
![]() |
[72] | Pal R, Singh B, Bhadada SK, et al. (2021) COVID-19-associated mucormycosis: An updated systematic review of literature. Mycoses In press. |
[73] | Rodriguez-Morales AJ, Sah R, Millan-Oñate J, et al. (2021) COVID-19 associated mucormycosis: the urgent need to reconsider the indiscriminate use of immunosuppressive drugs. Ther Adv Infect Dis 8: 1-5. |
[74] |
Tzilas V, Manali E, Papiris S, et al. (2020) Intravenous immunoglobulin for the treatment of COVID-19: a promising tool. Respiration 99: 1085. doi: 10.1159/000506650
![]() |
[75] |
Bongomin F, Asio LG, Ssebambulidde K, et al. (2021) Adjunctive intravenous immunoglobulins (IVIg) for moderate-severe COVID-19: emerging therapeutic roles. Curr Med Res Opin 37: 903-905. doi: 10.1080/03007995.2021.1903849
![]() |
[76] |
Kohler H, Kaveri S (2021) How IvIg can mitigate Covid-19 disease: A symmetrical immune network model. Monoclon Antibodies Immunodiagn Immunother 40: 17-20. doi: 10.1089/mab.2020.0041
![]() |
[77] |
Galeotti C, Kaveri SV, Bayry J (2020) Intravenous immunoglobulin immunotherapy for coronavirus disease-19 (COVID-19). Clin Transl Immunol 9: e1198. doi: 10.1002/cti2.1198
![]() |
[78] |
Calabrese LH (2020) Cytokine storm and the prospects for immunotherapy with COVID-19. Clevel Clin J Med 87: 389-393. doi: 10.3949/ccjm.87a.ccc008
![]() |
1. | Mansur Aliyu, Fatema Tuz Zohora, Abubakar Umar Anka, Kashif Ali, Shayan Maleknia, Mohammad Saffarioun, Gholamreza Azizi, Interleukin-6 cytokine: An overview of the immune regulation, immune dysregulation, and therapeutic approach, 2022, 111, 15675769, 109130, 10.1016/j.intimp.2022.109130 |