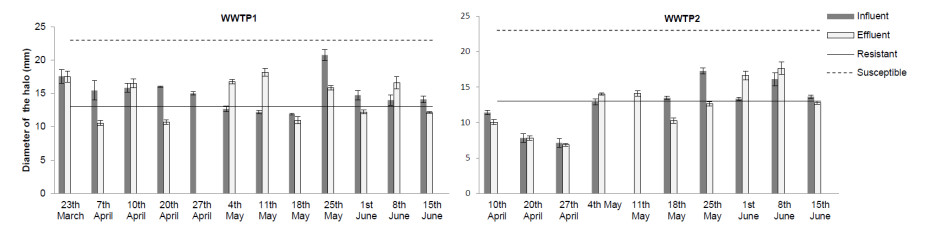
The antibiotic resistant bacteria (ARB) and antibiotic resistance genes (ARGs) are considered new classes of water contaminants due to their potential adverse effects on aquatic ecosystems and human health. This paper describes the susceptibility evaluation of Escherichia coli, isolated from the influent and effluent of the biological reactors in two wastewater treatment plants (WWTP1 and WWTP2), and the evaluation of the disinfection efficiency of the UV radiation system in WWTP2.
The exposure to different families of antibiotics was evaluated, namely, macrolides (erythromycin, azithromycin, clarithromycin), quinolones (ofloxacin, ciprofloxacin), nitroimidazoles (metronidazole), sulfanilamides (sulfamethoxazole) and trimethoprim, the latter is used in combination with sulfamethoxazole due to its synergistic effect.
The results of the antimicrobial susceptibility tests, using disc diffusion (Kirby–Bauer) method, showed the occurrence of E. coli strains resistance. The analysis performed indicated an overall resistance (considering both influents and effluents) in WWTP1 and WWTP2, of respectively: 33.3% and 37.5% to erythromycin; 0.0% and 4.0% to azithromycin; 25.0% and 29.2% to clarithromycin; 12.5% and 4.2% to ofloxacin; 16.7% and 4.2% to ciprofloxacin; 29.2% and 12.5% to trimethoprim; 41.7% and 12.5% to sulfamethoxazole. A variability of resistance was observed along the studied period, from WWTP1 and WWTP2, and from influent to effluent.
Disinfection by UV demonstrated good performance, achieving in some samples 100% removal of E. coli that has growth in TBX agar. However, a proper supervision is needed in order to achieve the allowed limits concerning the number of bacteria.
The results from this work contribute to a better awareness of ARB dissemination from wastewater treatment plants to the aquatic environment.
Citation: Vera Barbosa, Madalena Morais, Aurora Silva, Cristina Delerue-Matos, Sónia A. Figueiredo, Valentina F. Domingues. Comparison of antibiotic resistance in the influent and effluent of two wastewater treatment plants[J]. AIMS Environmental Science, 2021, 8(2): 101-116. doi: 10.3934/environsci.2021008
[1] | R. Destiani, M.R. Templeton . Chlorination and ultraviolet disinfection of antibiotic-resistant bacteria and antibiotic resistance genes in drinking water. AIMS Environmental Science, 2019, 6(3): 222-241. doi: 10.3934/environsci.2019.3.222 |
[2] | Riri Novita Sunarti, Sri Budiarti, Marieska Verawaty, Bayo Alhusaeri Siregar, Poedji Loekitowati Hariani . Diversity of Antibiotic-Resistant Escherichia coli from Rivers in Palembang City, South Sumatra, Indonesia. AIMS Environmental Science, 2022, 9(5): 721-734. doi: 10.3934/environsci.2022041 |
[3] | Carlos F. Amábile-Cuevas . Antibiotic resistance from, and to the environment. AIMS Environmental Science, 2021, 8(1): 18-35. doi: 10.3934/environsci.2021002 |
[4] | Jianfeng Wen, Yanjin Liu, Yunjie Tu and Mark W. LeChevallier . Energy and chemical efficient nitrogen removal at a full-scale MBR water reuse facility. AIMS Environmental Science, 2015, 2(1): 42-55. doi: 10.3934/environsci.2015.1.42 |
[5] | Melanie Voigt, Alexander Wirtz, Kerstin Hoffmann-Jacobsen, Martin Jaeger . Prior art for the development of a fourth purification stage in wastewater treatment plant for the elimination of anthropogenic micropollutants-a short-review. AIMS Environmental Science, 2020, 7(1): 69-98. doi: 10.3934/environsci.2020005 |
[6] | Carmen Gutiérrez-Bouzán, Valentina Buscio . Combining electrochemistry and UV for the simultaneous wastewater decolorization and reduction of salinity. AIMS Environmental Science, 2018, 5(2): 96-104. doi: 10.3934/environsci.2018.2.96 |
[7] | Xiaojun Liu, Ikbel Souli, Mohamad-Amr Chamaa, Thomas Lendormi, Claire Sabourin, Yves Lemée, Virginie Boy, Nizar Chaira, Ali Ferchichi, Pascal Morançais, Jean-Louis Lanoisellé . Effect of thermal pretreatment at 70 °C for one hour (EU hygienization conditions) of various organic wastes on methane production under mesophilic anaerobic digestion. AIMS Environmental Science, 2018, 5(2): 117-129. doi: 10.3934/environsci.2018.2.117 |
[8] | Joyce Cristale, Silvia Lacorte . PBDEs versus NBFR in wastewater treatment plants: occurrence and partitioning in water and sludge. AIMS Environmental Science, 2015, 2(3): 533-546. doi: 10.3934/environsci.2015.3.533 |
[9] | Karolina Nowocień, Barbara Sokołowska . Bacillus spp. as a new direction in biocontrol and deodorization of organic fertilizers. AIMS Environmental Science, 2022, 9(2): 95-105. doi: 10.3934/environsci.2022007 |
[10] | Abigail W. Porter, Sarah J. Wolfson, Lily. Young . Pharmaceutical transforming microbes from wastewater and natural environments can colonize microplastics. AIMS Environmental Science, 2020, 7(1): 99-116. doi: 10.3934/environsci.2020006 |
The antibiotic resistant bacteria (ARB) and antibiotic resistance genes (ARGs) are considered new classes of water contaminants due to their potential adverse effects on aquatic ecosystems and human health. This paper describes the susceptibility evaluation of Escherichia coli, isolated from the influent and effluent of the biological reactors in two wastewater treatment plants (WWTP1 and WWTP2), and the evaluation of the disinfection efficiency of the UV radiation system in WWTP2.
The exposure to different families of antibiotics was evaluated, namely, macrolides (erythromycin, azithromycin, clarithromycin), quinolones (ofloxacin, ciprofloxacin), nitroimidazoles (metronidazole), sulfanilamides (sulfamethoxazole) and trimethoprim, the latter is used in combination with sulfamethoxazole due to its synergistic effect.
The results of the antimicrobial susceptibility tests, using disc diffusion (Kirby–Bauer) method, showed the occurrence of E. coli strains resistance. The analysis performed indicated an overall resistance (considering both influents and effluents) in WWTP1 and WWTP2, of respectively: 33.3% and 37.5% to erythromycin; 0.0% and 4.0% to azithromycin; 25.0% and 29.2% to clarithromycin; 12.5% and 4.2% to ofloxacin; 16.7% and 4.2% to ciprofloxacin; 29.2% and 12.5% to trimethoprim; 41.7% and 12.5% to sulfamethoxazole. A variability of resistance was observed along the studied period, from WWTP1 and WWTP2, and from influent to effluent.
Disinfection by UV demonstrated good performance, achieving in some samples 100% removal of E. coli that has growth in TBX agar. However, a proper supervision is needed in order to achieve the allowed limits concerning the number of bacteria.
The results from this work contribute to a better awareness of ARB dissemination from wastewater treatment plants to the aquatic environment.
Antibiotics play a crucial role in the treatment of numerous types of infectious diseases caused by pathogenic microbes. According to the European Centre for Disease Prevention and Control (ECDC), in 2017, the average utilization of antibiotics in the European Union was 22 defined daily doses (DDD) per 1000 habitants and ranged from 10 DDD in Netherlands to 34 DDD in Cyprus [1]. Due to the high and continuous consumption of antibiotics they have been found in many aquatic systems, including in surface waters [2] and wastewaters [3]. Such occurrence has been accompanied by the proliferation of antibiotic resistance genes (ARG) and the appearance of resistant strains in the environment due to the increased selective pressure in bacterial population [4]. Today, the emergence and spread of antibiotic resistant bacteria (ARB) is one of the greatest threats to public health, seeing that this compromises the effectiveness of antimicrobial therapy, leading to more infections and causing higher morbidity and mortality [5,6].
The acquisition of genes that encode the resistance to antibiotics occur mainly via transfer of ARG between bacterial cells through plasmids and other mobile genetic elements, such as transposons or integrons [5]. Apart from horizontal gene transfer (HGT), the resistance of microbes to antibiotics may also arise through mutation [4,7]. Although the antibiotic resistance is an inevitable evolutionary consequence due to the natural selection process, the rate at which these processes occur and the number of resistant strains have greatly increased over the past years due to the selective pressure derived from anthropogenic activities [4].
Wastewater treatment plants (WWTPs) are important storage and emission sources of antibiotics and ARGs to the environment. Although WWTPs are useful for removing antibiotics and ARGs, they are not specifically designed for this task [8]. The effluents from municipal wastewater treatment plants (WWTPs) have been identified as the most significant anthropogenic sources for the dissemination of antibiotic residues, ARB and ARG, into the environment [7]. Since the antibiotics consumed are not completely metabolized they are excreted in urine and faeces, not only as metabolites but also as parent compounds into the wastewater [9,10]. Among the multiple pathways of antibiotic resistance dissemination, hospital effluents discharged into WWTPs represent a relevant source of ARG and ARB. Studies have demonstrated that hospital effluents contain higher prevalence of ARG, like blaTEM and vanA genes [11,12], higher concentrations of antibiotic residues [13], and higher loads of ARB than municipal effluents [14]. The high resistance rates of Enterococcus spp. to the antibiotics vancomycin, erythromycin and ciprofloxacin in Portugal were found in cities with a high number of hospital units [15]. The same phenomena has been observed in several countries [16,17]. Thus, there is an increasing concern about the environmental risk posed by the wastewater discharge on receivers, namely due to the dissemination of antimicrobial resistance.
Conventional WWTPs assemble three sequential steps, preliminary, primary and secondary treatments, which have been designed to remove bulk solids, sand, floating fat, grease and biodegradable compounds from wastewater [18]. Considering that WWTPs have a limited capacity of removing micropollutants, such as antibiotics, these components end up in the aquatic environment creating selective pressure for the proliferation of ARB and ARGs in aquatic ecosystems [18,19,20,21]. In fact, WWTPs have been recognized as reservoirs for the spread of antibiotic resistance [22]. The microbial communities present in the activated sludge process, one of the most used secondary treatments, are highly concentrated. The presence in the biological reactor inflow of high nutrient content, under subinhibitory concentrations of antibiotics, fungicides and metals, create a favorable environment for the spread of ARGs [23]. The proximity between bacterial cells promotes genetic exchange through HGT of ARB, and the high nutrient content create the ideal conditions for survival and growth of ARB [7,20].
To minimize the risk of spreading microorganisms [3] disinfection processes have been implemented in some WWTPs before the discharge to receiving waters. Chlorine, ultraviolet (UV) radiation and ozonation are the most common disinfection technologies currently applied in WWTPs worldwide [18,24,25]
Due to the low cost of chlorine, it is the most common method of chemical disinfection. This method demands a high quality treated water, low levels of suspended solids, organic matter and ammoniacal nitrogen in order to guarantee its efficiency and reduce the formation of oxidation by-products, such as organochlorinated compounds that are toxic and carcinogenic [25].
Both UV and ozone are well suited disinfection agents concerning the objective of degradation and deactivation of ARGs. UV radiation causes photochemical damage to RNA and DNA, preventing the reproduction of cells [25]. It is directly absorbed by pyrimidine and purine nucleobases causing DNA mutations which then impairs the synthesis of critical proteins and DNA replication. As a consequence, in the absence of repair mechanisms, there will be cell death and inactivation of ARGs [10,26,27]. Ozonation works on a different principle, ozone can penetrate the bacterial cells, breaks the cell walls, and also promotes multiple oxidation reactions of cellular constituents damaging cell structures and DNA [10,27].
In general, these disinfection processes contribute to the effective decrease of microbial loads, but it has been demonstrated that they might have potential to act selectively over some bacterial groups. Under the stressful conditions imposed by the disinfection processes some of the bacteria species will die, whilst others will enter a dormant state recovering when the stressors are removed [18]. In this way, disinfection treatment may compromise the bacterial diversity, which will contribute to the enrichment of ubiquitous bacteria associated with acquirement of ARGs [28]. On the other hand, even if the disinfection process has shown an effective reduction of ARB, intact remnants of ARGs may prevail and confer antibiotic resistance to bacterial populations [26,29]. Thus, the disinfection process should be capable of deactivating ARGs and inactivating the corresponding ARB. There is still much to learn about the efficiency of tertiary treatment technologies (disinfection processes) for removal of ARB. In this context, the aim of the present work was to study the occurrence of antibiotic resistance of Escherichia coli isolated from the inflow and outflow of the biological reactors (secondary treatment) from two typical municipal WWTPs in the North of Portugal. Furthermore, the efficiency of UV radiation to inactive microorganisms was also evaluated. The antibiotics used in this study were selected by taking into account their presence and persistence in the environment [21,30]. Sulfonamides, fluoroquinolones and macrolides are the most persistent antibiotic families; tetracyclines are less mobile, but can persist for relatively long periods in the absence of sunlight; and aminoglycosides and β-lactam antibiotics show less persistence [21]. In previous studies in WWTP effluents, sulfamethoxazole and quinolones (in particular, ciprofloxacin and ofloxacin) were the antibiotics present at higher concentrations [3,30].
Sampling campaigns were performed in two urban WWTPs, which receive only domestic wastewater.
The WWTP1 was designed for 25,000 population equivalent and an average flowrate of 14,000 m3/d. This treatment plant has preliminary treatment (screening and removal of grit, as well as oil and grease), primary treatment (sedimentation) and secondary treatment carried out into a biological reactor (activated sludge in extended aeration regime).
The WWTP2 was designed for 80,000 population equivalent and an average flow rate of 7,000 m3/d. It has preliminary treatment (screening and grit removal), primary treatment (sedimentation), secondary treatment performed into a biological reactor (activated sludge in extended aeration regime) and tertiary treatment (micro screening and disinfection by ultraviolet irradiation), according to the discharge license this last step is only mandatory during the Summer.
A total of 12 sampling campaigns were performed in two urban WWTPs (WWTP1 and WWTP2) from March to June (2015) in weekdays. The samples were collected by dipping of a sterile 1 L bottle in the upstream and downstream flow of the biological reactor in both WWTPs under study, and after the disinfection step of WWTP2. Their transportation to the laboratory (30 min) was made under controlled temperature conditions, and they were immediately processed. Manipulation of the samples was made under aseptic conditions.
The sample collection inside the biological reactors was performed by dipping of a vessel. As they are continuous stirred tank reactors, the composition inside the reactors is the same as in its outlet. The characterization of these samples concerning pH, temperature, and total suspended solids (TSS) and volatile suspended solids (VSS) was performed following standard methods [31].
The following procedure was adopted for the enumeration of E. coli through membrane filtration: 1.00 mL of sample was filtered through 0.45 μm nitrocellulose membrane; then it was placed on a plate containing TBX agar (Oxoid) and incubated at 37 ℃ for 24 h; finally, the number of positive (blue-green) colonies on the plate were counted. The incubation temperature was 37 ℃. The plates prepared with TBX agar were stored at 4 ℃ for 1–4 days before use.
All blue-green colonies were incubated in a Fluorocult broth at 37 ℃ for 24 h and the green tubes indol positive were considered as E. coli.
Antimicrobial susceptibility of the E. coli isolates was tested for eight antimicrobials using disc diffusion (Kirby–Bauer) method following the Clinical and Laboratory Standards Institute (CLSI, 2012) criteria. This technique consists in putting the disk containing the antibiotic in direct contact with the medium (Mueller-Hinton from Liofilchem) previously inoculated with the E. coli strains isolated as described in section 2.3. The disks used contained the following antimicrobials: erythromycin (15 μg); azithromycin (15 μg); clarithromycin (15 μg); ofloxacin (5 μg); ciprofloxacin (5 μg); sulfamethoxazole (50 μg); trimethoprim (5 μg); and metronidazole (50 μg).
After an overnight incubation period at 37 ℃, the inhibition halos were measured with a certified electronic digital caliper (Paget Trading Ltd., Woodrow London SE18 5DH, UK) and compared to standard halos, allowing the results to be categorized. For the E. coli/antibiotic combination there are two breakpoints (marked in Figures 1–7, for each antibiotic), which are the boundaries of the 3 categories of interpretation: susceptible (in vitro inhibition by an antibiotic concentration that is associated with a high likelihood of therapeutic success), intermediate (uncertain probability of successful treatment), resistant (high likelihood of therapeutic failure) [32]. The breakpoint system used was proposed by the European Committee of Antimicrobial Susceptibility Testing, and described by Tascini et al. [32]. The standard deviations of the sample replicates (n = 3) represented in Figures 1–7 were considered in the comparison with the breakpoints.
The values of operational parameters monitored inside the biological reactors of the WWTPs, pH, temperature, TSS and VSS (Table 1), indicate normal operational conditions in both WWTPs. Comparing these values with the typical values for operation of an activated sludge biological reactor operating under an extended aeration regime [25]: i) the pH is in the typical range 6–9, near a neutral pH, where optimal performance occurs; ii) temperature values are in the typical range of 10–30 ℃ for psychrophilic microorganisms, 18.8–23.9 ℃ and 15.0–21.0 ℃ respectively for WWTP1 and WWTP2, nevertheless occasionally it is outside the optimal range, 12–18 ℃; iii) TSS values should be in the typical range 2000–5000 mg/L but the values are lower than 2000 mg/L, however a healthy percentage for the ratio VSS/TSS (typically equal or greater than 75%) was observed, being higher than 87 and 85% respectively for WWTP1 and WWTP2 (VSS represents the live bacteria portion of solids) [25].
Date | pH | T (℃) | TSS (mg/L) | VSS (mg/L) | VSS/TSS (%) | |
Biological reactor of WWTP1 | 23/03/15 | 7.18 | 16.8 | 2030 | 1810 | 89.2 |
07/04/15 | 7.29 | 19.2 | 2080 | 1900 | 91.3 | |
10/04/15 | 7.22 | 18.2 | 2160 | 1960 | 90.7 | |
20/04/15 | 7.34 | 18.9 | 1950 | 1700 | 87.2 | |
27/04/15 | 7.05 | 18.7 | 1710 | 1550 | 90.6 | |
04/05/15 | 7.11 | 18.7 | 1310 | 1230 | 93.9 | |
11/05/15 | 7.09 | 21.0 | 1560 | 1470 | 94.2 | |
18/05/15 | 7.07 | 22.1 | 1240 | 1190 | 96.0 | |
25/05/15 | 7.16 | 22.8 | 1250 | 1170 | 93.6 | |
01/06/15 | 7.27 | 22.1 | 1420 | 1350 | 95.1 | |
08/06/15 | 7.33 | 23.9 | 1410 | 1300 | 92.2 | |
15/06/15 | 7.29 | 21.7 | 1660 | 1510 | 91.0 | |
Biological reactor of WWTP2 | 23/03/15 | 6.65 | 15.5 | 1040 | 1030 | 99.0 |
07/04/15 | 7.06 | 16.9 | 970 | 960 | 99.0 | |
10/04/15 | 6.85 | 18.5 | 980 | 870 | 88.8 | |
20/04/15 | 7.32 | 15.0 | 2170 | 1850 | 85.3 | |
27/04/15 | 6.98 | 16.4 | 1970 | 1680 | 85.3 | |
04/05/15 | 6.12 | 18.2 | 1720 | 1490 | 86.6 | |
11/05/15 | 6.51 | 19.1 | 1930 | 1710 | 88.6 | |
18/05/15 | 6.47 | 18.7 | 1550 | 1380 | 89.0 | |
25/05/15 | 6.52 | 20.7 | 1790 | 1560 | 87.2 | |
01/06/15 | 7.12 | 21.0 | 1840 | 1570 | 85.3 | |
08/06/15 | 7.24 | 20.2 | 1550 | 1360 | 87.7 | |
15/06/15 | 7.38 | 20.3 | 1930 | 1720 | 89.1 |
The macrolides, specifically erythromycin, azithromycin and clarithromycin, are the antibiotics most frequently detected in WWTPs [22]. It is noteworthy that erythromycin, clarithromycin and azithromycin are included in the EU first watch list in the Directive 2015/495/EU [33]. These class of antibiotics, bind to the 50S ribosomal subunit with a specific target in the 23S ribosomal RNA molecule and various ribosomal proteins [13]. However, the resistance profile of the E. coli to the three selected antibiotics of this class has some differences (Figures 1–3).
The overall picture of the E. coli in the two WWTPs shows several situations of resistance to erythromycin both in the influent and effluent of the biological reactor (Figure 1). The most critical case for erythromycin was on the 27th of April on WWTP1, for which no zone inhibition was evident from the effluent samples, although the strains from influent showed a halo with 15.0 mm ± 0.3. Moreover, it was also visible a significant decrease (from the influent to the effluent) of the inhibition zone below the resistant limits, on the 7th, the 20th and the 27th of April (Figure 1). The resistance to this macrolide in environmental samples can be justified by the ubiquitous presence of this antibiotic in the aquatic environment, from the Antarctic [34] to the Atlantic Ocean [35], and in wastewaters [31]. From March to June, 50% of the effluent samples analyzed, and released into the environment, have E. coli strains considered erythromycin resistant.
Concerning WWTP2, during April the E. coli strains from the influent and effluent showed halos lower than the value considered resistant. During these three months of campaign, 42% of the total effluent analysis indicate resistance to erythromycin.
Azithromycin has been described as more effective than erythromycin for many genera of the family Enterobacteriaceae [36]. This is in agreement with the results presented, where 100% of E. coli strains from the effluents of WWTP1 and WWTP2 are susceptible to azithromycin. The inhibition zones that resulted from the exposure to azithromycin discs are among the largest dimensions of this study, the inhibition zones ranged from 32.3 to 18.4 mm (Figure 2). On the 27th of April, in WWTP1 a noticeable decrease of the inhibition zone was identified, from 29.5 to 18.4 mm. The only concerning situation in WWTP2 has occurred on the11th of May, when the strains isolated from the influent of the biological reactor were considered as resistant (Figure 2). The results suggest that there was an entrance of bacteria azithromycin resistant, but they did not persist after the biological treatment of WWTP2.
About clarithromycin evaluation (Figure 3), 25% of influent and effluent analysis in WWTP1 were considered susceptible. In WWPP2 none of the influent samples was considered susceptible and only 8.3% of the effluent samples were considered susceptible (Figure 3).
In the same day (27th of April) antibiotic resistance occurs for both macrolides, clarithromycin and erythromycin, showing the same pattern, which suggests that a common resistance mechanism for these two antibiotics may occur. Also, on the 20th of April, in both WWTPs, and on the 27th in WWTP1, the strains from the effluent reveal no susceptibility to clarithromycin. This may suggest an acquired resistance during the biological treatment. The opposite occurs in WWTP2 on the 11th of May. The E. coli strains exhibit a higher resistance to erythromycin than to the other two tested macrolides, showing 33.3% of resistance in the analysis of WWTP1 and 37.5% in WWPT2. Several reports identified resistance to clarithromycin associated to mutations on the positions A2058 or A2059 of the 23S rRNA [37].
The quinolones act by converting their targets, gyrase and topoisomerase IV, into toxic enzymes that fragment the bacterial chromosome. The susceptibility of the E. coli isolated from the two WWTPs to quinolone antibiotics was also tested. Ofloxacin and ciprofloxacin, which are from the second generation of quinolones (with the introduction of a fluorine), displayed a considerably improved activity against gyrase, and enhanced pharmacokinetics and pharmacodynamics [38]. An oscillation of sensibility to ofloxacin (Figure 4) is observed before and after the biological treatment of WWTP2 in March and April, and in WWTP1, from April to May. In the end of May and June both WWTPs showed halos > 16 mm in the influent and effluent of the biological reactor, thus these strains were classified as susceptible. The most critical situations of resistance were identified on the 27th of April in WWTP1 (without halo at the entrance) and on the 18th of May in WWTP2, where the resistance deeply increases during the biological treatment (Figure 4).
Ciprofloxacin and ofloxacin are effective for treating infections caused by many different types of bacteria even for antibacterial prophylaxis [39]. Ciprofloxacin was the first quinolone that displayed significant activity outside of the urinary tract. After almost three decades in clinical use, ciprofloxacin remains one of the most commonly prescribed antimicrobial drugs, being listed by the World Health Organization (WHO) as an essential medicine and a critically important antibiotic [40]. So, these antibiotics are in the list of the main antibiotic compounds detected in the influent and effluent of WWTPs [22]. The removal of some antibiotics was investigated in six WWTPs in Italy during summer and winter. It was found that similar removal efficiencies were obtained for ciprofloxacin and ofloxacin in both seasons, 60% and 50%, respectively [41]. Varela et al. reported that the percentage of Enterococci isolates resistant to ciprofloxacin was 4.0% in the influent, while it decreased to 3.4% in the effluent [42].
In this study (Figure 5), a significant resistance increase is observed after the biological treatment in several situations: on the 23th March, and on the 1st June in WWTP1; and on the 10th April, on the 18th and on the 25th May in WWTP2.
Looking at the results obtained for ciprofloxacin and ofloxacin (Figures 4 and 5), E. coli resistant (to both) antibiotics was discharged into the environment only in two days in WWTP1 (23th March and 27th April) and in one day in WWTP2 (18th May). However, the spread of these resistant microorganisms may pose environmental and health risks. The acquisition mechanisms of quinolone resistance has been described as: i) chromosomal mutations in genes encoding the protein targets, or mutations causing reduced drug accumulation, either by a decreased uptake or by an increased efflux; or ii) plasmid‐located genes associated with quinolone resistance [43].
Trimethoprim and sulfamethoxazole are synergistic folate pathway inhibitors. The combination of trimethoprim and sulfamethoxazole is widely used not only for therapy but also for prevention of infections. The combination trimethoprim/sulfamethoxazole is recommended for use both in adults and children for several infections and as prophylactic. Trimethoprim affects the bacterial folic acid synthesis by the inhibition of dihydrofolate reductase (DHFR). Bacteria may become resistant to trimethoprim by several mechanisms: the development of permeability barriers, efflux pumps; the existence of naturally insensitive target DHFR enzymes; mutational and regulation changes in target enzymes; and the acquirement of drug-resistant target enzymes [44].
Although in the WWTP1 in 50% of the strains (isolated on the same day) from influent and from effluent were susceptible to trimethoprim (Figure 6), several critical situations were identified: on the 7th of April no halo was observed in both influent and effluent samples; a deep increase of resistance was noticed from the 10th to the 27th of April, when the E. coli strains from the influent were susceptible, while the strains from the effluent showed resistance (without halo). This suggests a resistance dissemination during the WWTP1 biological treatment. After the 4th of May, in the seven following campaigns until the 15th of June, the E. coli strains from effluent were susceptible to trimethoprim.
Regarding the WWTP2, the same pattern is observed, 50% of the strains (isolated on the same day) from influent and effluent were susceptible to trimethoprim (Figure 6). However, several critical situations (without halo) were observed, on the 20th of April and the 11th of May in the influent and, the most concerning situation occurred on the 10th of April when the strains isolated from the effluent do not exhibit any halo.
Sulfamethoxazole inhibits the production of dihydrofolate from para-aminobenzoic acid. Usually, the antimicrobial susceptibility tests are performed with trimethoprim or trimethoprim/sulfamethoxazole. According to the authors best knowledge, information is not available regarding the susceptibility halo of SMX for 50 µg disks.
In both WWTPs (Figure 7), the samples of E. coli strains isolated from the influent and effluent, in the same day, that showed susceptibility to sulfamethoxazole represent 50% of the samples analyzed. In WWTP1, the E. coli strains isolated from the influent and effluent demonstrated resistance (without halo) to sulfamethoxazole on the 7th, on the 27th of April, and the 8th of June (Figure 7). Between the 10th and the 27th of April, despite the influent strains showed susceptibility, resistance (without halo) was exhibited in the strains isolated from the effluent in these same days, which represents a critical increase of resistance. The same has occurred in the WWTP2 on the 10th of April (Figure 7), being considered the most critical situation.
Metronidazole is described to have some activity against coliforms in vivo, despite showing in vitro resistance [45]. According to the fact that both WWTPs own aerobic processes is not predictable the occurrence of resistant strains to metronidazole. However, E. coli strains with lesions in their DNA repair systems may show a decrease in minimal inhibitory concentration [44]. Therefore, it is pertinent to evaluate the sensibility of E. coli to this antibiotic. But, as expected, none of these strains exhibited resistance in the tests performed.
Different behaviors were observed during this study in both WWTPs, namely variability of resistance from antibiotic to antibiotic, along with time, from WWTP1 and WWTP2, and increase or decrease of resistance when comparing the E. coli isolated from influent and effluent. The different behaviors reported may be related with different factors that interact, which are described below.
Wastewater contains substances that may exert an array of effects on bacteria, being sometimes designated as stressors, of which are examples the antibiotic residues and metabolites thereof. These stressors may also shape the surviving community because different organisms, or groups of related organisms, have different degrees of tolerance, or defensive responses, against their adverse effects [18,46]. So, depending on the type of antibiotic that may be present in the WWTP it may cause different resistance behavior in influent and effluent.
The difference of resistance observed may also be related with the microbiota (mainly composed of human commensal bacteria) present at that time, which is mixed with bacteria from different origins that may be entering and colonizing the sewage treatment system [18]. In this environment, the fraction of ARB may reach more than 50% at least within a given group (e.g., enterobacteria or enterococci) [18,46].
In the secondary treatment the fraction of ARB & ARG gets in contact with the microorganisms present in the biomass suspension, which submitted to the potential selective pressure of antibiotics present in the inflow increase the potential of ARB & ARG's dissemination.
The sludge recirculation, from the secondary sedimentation tank to the biological activated sludge reactor, ensures enough cells to reduce the organic load of the wastewater. The microbiota (including ARB) arriving to the biological reactor is stimulated to compete with the activated sludge bacteria for the available organic matter. This intense metabolic activity creates an important bacterial community dynamics. The shifts occurring in the bacterial community, the fitness of ARB, and the success with which their ARGs are spread to other bacteria are crucial to the ARB & ARG's dissemination [18]. Since municipal WWTPs are the direct link to the aquatic environment, and they are not designed to eliminate ARGs and ARBs, they promote the spread of ARGs in allochthonous bacteria [47]. This study supports the need of advanced combined treatments to prevent the dissemination of ARG and ARB as recommend by the World Health Organization.
The revised standards of World Health Organization for unrestricted irrigation of treated wastewater were 1000 fecal coliforms/100 mL (monthly mean) although a more stringent guideline (200 fecal coliforms/100 mL) was considered more appropriate for public lawns with which the public may come into direct contact [48]. More recently the United States Environmental Protection Agency has included effluent limits for E. coli, rather than fecal coliforms, in order to protect the use of primary contact recreation in the receiving water: the monthly geometric mean concentration of E. coli must not exceed 126 organisms per 100 mL, further, no single sample may exceed 406 organisms per 100 mL (instantaneous maximum limit) [48]. However regulations for disinfection are site-specific, have seasonal standards, and are under continual review [25].
Disinfection is mandatory in WWTP2 because it discharges into a sensitive zone, but in this case only in the hot season and there is no legal limit imposed. Disinfection efficiency was evaluated through E. coli count before and after ultraviolet (UV) irradiation.
The efficiency of the disinfection treatment using UV radiation from the 25th of May to the 22nd of June is illustrated in Figure 8. The removal of bacteria from treated wastewater was complete (the counted values were lower than the limit of detection) on the 8th and the 15th of June, but it should be noted that the initial concentration was quite low. For the other tested days, the efficiency was close to 50% and the concentration after disinfection was much higher than 406 bacteria per 100 mL [48]. In fact the inability to achieve permit limitations is the most common problem related with UV disinfection, which may be due to: hydraulic problems related with the creation of density and/or eddy currents and/or dead volumes that cause short circuiting and therefore reduce the contact time leading to ineffective use of the UV system, which is difficult to detect and needs the help of studies of computational fluid dynamics; formation of biofilms on the exposed surfaces of the UV reactor, which demands frequent cleaning using a cleaning agent or disinfectant to remove them; presence of particles that disperse the radiation, that should be prior removed by filtration [25]. Although the disinfection system by UV radiation is efficient it has been demonstrated that it promotes the spread of ARGs mediated by conjugative transfer of plasmids between E. coli strains [49]. Therefore, special care should be taken in the use UV radiation for disinfection.
The results from this work contribute to the aware of ARB dissemination from WWTPs. The evaluation of the antibiotic susceptibility of E. coli strains isolated from the influent and effluent of the biological reactors in two WWTPs allowed the identification of several critical situations (e.g., erythromycin, clarithromycin, and trimethoprim): i) occurrence of bacteria resistant after the biological treatment, having as consequence the discharge in the aquatic environment of resistant strains; ii) the identification of extreme resistance (zero halo of antibiotic's diffusion), in the influent and/or effluent of the biological reactor, being the last the most critical situation; iii) and the prevalence of the resistance over time.
The analysis performed before and after the UV irradiation system revealed that the disinfection processes contributed to the decrease of bacteria number released in this WWTP. However, a reduction of the bacteria number released does not mean a reduction of ARB because UV irradiation may promote the conjugative transfer of plasmids between E. coli strains. Reuse of treated wastewater demands stringent requirements in order to avoid potential environmental and public health impacts. The use of other disinfection system, such as ozonation, would be a possible alternative to avoid the spread of ARB both in the aquatic environment and soil, when treated wastewater is reused, namely for irrigation, reducing the potential risks for environmental and public health.
This work was supported by the Associate Laboratory for Green Chemistry-LAQV which is financed by national funds from FCT/MCTES (UIDB/50006/2020). The authors would like to thank the EU and FCT/UEFISCDI/FORMAS for funding, in the frame of the collaborative international consortium REWATER financed under the ERA-NET Cofund WaterWorks2015 Call. This ERA-NET is an integral part of the 2016 Joint Activities developed by the Water Challenges for a Changing World Joint Programme Initiative (WaterJPI/0007/2016). The authors are greatly indebted to all financing sources.
All authors declare no conflicts of interest in this paper.
[1] | ECDC (2018) Annual Epidemiological Report for 2017 (Antimicrobial Consumption). |
[2] | Fernandes MJ, Paíga P, Silva A, et al. (2020) Antibiotics and antidepressants occurrence in surface waters and sediments collected in the north of Portugal. Chemosphere 239. |
[3] | Maia AS, Paíga P, Delerue-Matos C, et al. (2020) Quantification of fluoroquinolones in wastewaters by liquid chromatography-tandem mass spectrometry. Environ Pollut 259. |
[4] |
von Wintersdorff CJH, Penders J, van Niekerk JM, et al. (2016) Dissemination of Antimicrobial Resistance in Microbial Ecosystems through Horizontal Gene Transfer. Front Microbiol 7: 75–82. doi: 10.3389/fmicb.2016.00173
![]() |
[5] |
Korzeniewska E, Harnisz M (2018) Relationship between modification of activated sludge wastewater treatment and changes in antibiotic resistance of bacteria. Sci Total Environ 639: 304–315. doi: 10.1016/j.scitotenv.2018.05.165
![]() |
[6] | Rodriguez-mozaz S, Chamorro S, Marti E, et al. (2015) Occurrence of antibiotics and antibiotic resistance genes in hospital and urban wastewaters and their impact on the receiving river. Water Res 69. |
[7] |
McConnell MM, Truelstrup Hansen L, Jamieson RC, et al. (2018) Removal of antibiotic resistance genes in two tertiary level municipal wastewater treatment plants. Sci Total Environ 643: 292–300. doi: 10.1016/j.scitotenv.2018.06.212
![]() |
[8] |
Chen Y, Shen W, Wang B, et al. (2020) Occurrence and fate of antibiotics, antimicrobial resistance determinants and potential human pathogens in a wastewater treatment plant and their effects on receiving waters in Nanjing, China. Ecotoxicol Environ Saf 206: 111371. doi: 10.1016/j.ecoenv.2020.111371
![]() |
[9] |
Adefisoye MA, Okoh AI (2016) Identification and antimicrobial resistance prevalence of pathogenic Escherichia coli strains from treated wastewater effluents in Eastern Cape, South Africa. Microbiologyopen 5: 143–151. doi: 10.1002/mbo3.319
![]() |
[10] |
Lamba M, Ahammad SZ (2017) Performance comparison of secondary and tertiary treatment systems for treating antibiotic resistance. Water Res 127: 172–182. doi: 10.1016/j.watres.2017.10.025
![]() |
[11] |
Varela AR, André S, Nunes OC, et al. (2014) Insights into the relationship between antimicrobial residues and bacterial populations in a hospital-urban wastewater treatment plant system. Water Res 54: 327–336. doi: 10.1016/j.watres.2014.02.003
![]() |
[12] |
Narciso-da-Rocha C, Varela AR, Schwartz T, et al. (2014) blaTEM and vanA as indicator genes of antibiotic resistance contamination in a hospital–urban wastewater treatment plant system. J Glob Antimicrob Resist 2: 309–315. doi: 10.1016/j.jgar.2014.10.001
![]() |
[13] | Voigt AM, Skutlarek AD, Timm AC, et al. (2019) Liquid chromatography-tandem mass spectrometry as a fast and simple method for the determination of several antibiotics in different aqueous matrices. Enviromental Chem. |
[14] | Rita A, Ferro G, Vredenburg J, et al. (2013) Vancomycin resistant enterococci : From the hospital effluent to the urban wastewater treatment plant. Sci Total Environ 450–451: 155–161. |
[15] | Martins da Costa P, Vaz-Pires P, Bernardo F (2006) Antimicrobial resistance in Enterococcus spp. isolated in inflow, effluent and sludge from municipal sewage water treatment plants. Water Res 40: 1735–1740. |
[16] |
Girijan SK, Paul R, Rejish Kumar VJ, et al. (2020) Investigating the impact of hospital antibiotic usage on aquatic environment and aquaculture systems: A molecular study of quinolone resistance in Escherichia coli. Sci Total Environ 748: 141538. doi: 10.1016/j.scitotenv.2020.141538
![]() |
[17] | Sib E, Lenz-Plet F, Barabasch V, et al. (2020) Bacteria isolated from hospital, municipal and slaughterhouse wastewaters show characteristic, different resistance profiles. Sci Total Environ 746. |
[18] |
Manaia CM, Rocha J, Scaccia N, et al. (2018) Antibiotic resistance in wastewater treatment plants: Tackling the black box. Environ Int 115: 312–324. doi: 10.1016/j.envint.2018.03.044
![]() |
[19] | Sabri NA, Schmitt H, Van der Zaan B, et al. (2018) Prevalence of antibiotics and antibiotic resistance genes in a wastewater effluent-receiving river in the Netherlands. J Environ Chem Eng. |
[20] |
Karkman A, Do TT, Walsh F, et al. (2018) Antibiotic-Resistance Genes in Waste Water. Trends Microbiol 26: 220–228. doi: 10.1016/j.tim.2017.09.005
![]() |
[21] |
Brown KD, Kulis J, Thomson B, et al. (2006) Occurrence of antibiotics in hospital, residential, and dairy effluent, municipal wastewater, and the Rio Grande in New Mexico. Sci Total Environ 366: 772–783. doi: 10.1016/j.scitotenv.2005.10.007
![]() |
[22] |
Wang J, Chu L, Wojnárovits L, et al. (2020) Occurrence and fate of antibiotics, antibiotic resistant genes (ARGs) and antibiotic resistant bacteria (ARB) in municipal wastewater treatment plant: An overview. Sci Total Environ 744: 140997. doi: 10.1016/j.scitotenv.2020.140997
![]() |
[23] |
Ding H, Qiao M, Zhong J, et al. (2020) Characterization of antibiotic resistance genes and bacterial community in selected municipal and industrial sewage treatment plants beside Poyang Lake. Water Res 174: 115603. doi: 10.1016/j.watres.2020.115603
![]() |
[24] |
Silva I, Tacão M, Tavares RDS, et al. (2018) Fate of cefotaxime-resistant Enterobacteriaceae and ESBL-producers over a full-scale wastewater treatment process with UV disinfection. Sci Total Environ 639: 1028–1037. doi: 10.1016/j.scitotenv.2018.05.229
![]() |
[25] | Eddy M (2007) Wastewater Engineering, Mc Graw Hill. |
[26] |
Dodd MC (2012) Potential impacts of disinfection processes on elimination and deactivation of antibiotic resistance genes during water and wastewater treatment. J Environ Monit 14: 1754–1771. doi: 10.1039/c2em00006g
![]() |
[27] |
Sousa JM, Macedo G, Pedrosa M, et al. (2017) Ozonation and UV 254 nm radiation for the removal of microorganisms and antibiotic resistance genes from urban wastewater. J Hazard Mater 323: 434–441. doi: 10.1016/j.jhazmat.2016.03.096
![]() |
[28] |
Becerra-Castro C, Macedo G, Silva AMT, et al. (2016) Proteobacteria become predominant during regrowth after water disinfection. Sci Total Environ 573: 313–323. doi: 10.1016/j.scitotenv.2016.08.054
![]() |
[29] |
Li N, Sheng GP, Lu YZ, et al. (2017) Removal of antibiotic resistance genes from wastewater treatment plant effluent by coagulation. Water Res 111: 204–212. doi: 10.1016/j.watres.2017.01.010
![]() |
[30] |
Santos LHMLM, Gros M, Rodriguez-Mozaz S, et al. (2013) Contribution of hospital effluents to the load of pharmaceuticals in urban wastewaters: Identification of ecologically relevant pharmaceuticals. Sci Total Environ 461–462: 302–316. doi: 10.1016/j.scitotenv.2013.04.077
![]() |
[31] | Baird R, Laura B (2017) Standard methods for the examination of water and wastewater, Washington, D.C., American Public Health Association. |
[32] |
Tascini C, Sozio E, Viaggi B, et al. (2016) Reading and understanding an antibiogram. Ital J Med 10: 289–300. doi: 10.4081/itjm.2016.794
![]() |
[33] | European Commission (2018) Commission implementing decision establishing a watch list of substances for union-wide monitoring in the field of water policy pursuant to Directive 2008/105/EC of the European Parliament and of the Council. |
[34] |
Hernández F, Calısto-Ulloa N, Gómez-Fuentes C, et al. (2019) Occurrence of antibiotics and bacterial resistance in wastewater and sea water from the Antarctic. J Hazard Mater 363: 447–456. doi: 10.1016/j.jhazmat.2018.07.027
![]() |
[35] |
Barreto A, Luis LG, Pinto E, et al. (2019) Genotoxicity of gold nanoparticles in the gilthead seabream (Sparus aurata) after single exposure and combined with the pharmaceutical gemfibrozil. Chemosphere 220: 11–19. doi: 10.1016/j.chemosphere.2018.12.090
![]() |
[36] |
Girard AE, Girard D, English AR, et al. (1987) Pharmacokinetic and in vivo studies with azithromycin (CP-62,993), a new macrolide with an extended half-life and excellent tissue distribution. Antimicrob Agents Chemother 31: 1948–1954. doi: 10.1128/AAC.31.12.1948
![]() |
[37] |
Dinos GP (2017) The macrolide antibiotic renaissance. Br J Pharmacol 174: 2967–2983. doi: 10.1111/bph.13936
![]() |
[38] |
Aldred KJ, Kerns RJ, Osheroff N (2014) Mechanism of quinolone action and resistance. Biochemistry 53: 1565–1574. doi: 10.1021/bi5000564
![]() |
[39] |
Yeshurun M, Vaxman I, Shargian L, et al. (2018) Antibacterial prophylaxis with ciprofloxacin for patients with multiple myeloma and lymphoma undergoing autologous haematopoietic cell transplantation: a quasi-experimental single-centre before-after study. Clin Microbiol Infect 24: 749–754. doi: 10.1016/j.cmi.2017.11.019
![]() |
[40] | Correia S, Poeta P, Hébraud M, et al. (2017) Mechanisms of quinolone action and resistance: where do we stand? J Med Microbiol 66: 551–559. |
[41] |
Castiglioni S, Bagnati R, Fanelli R, et al. (2006) Removal of pharmaceuticals in sewage treatment plants in Italy. Environ Sci Technol 40: 357–363. doi: 10.1021/es050991m
![]() |
[42] |
Varela AR, Ferro G, Vredenburg J, et al. (2013) Vancomycin resistant enterococci: From the hospital effluent to the urban wastewater treatment plant. Sci Total Environ 450–451: 155–161. doi: 10.1016/j.scitotenv.2013.02.015
![]() |
[43] |
Fàbrega A, Madurga S, Giralt E, et al. (2009) Mechanism of action of and resistance to quinolones. Microb Biotechnol 2: 40–61. doi: 10.1111/j.1751-7915.2008.00063.x
![]() |
[44] |
Šeputiene V, Povilonis J, Ružauskas M, et al. (2010) Prevalence of trimethoprim resistance genes in Escherichia coli isolates of human and animal origin in Lithuania. J Med Microbiol 59: 315–322. doi: 10.1099/jmm.0.015008-0
![]() |
[45] |
Onderdonk AB, Louie TJ, Tally FP, et al. (1979) Activity of metronidazole against escherichia coli in experimental infra-abdominal sepsis. J Antimicrob Chemother 5: 201–210. doi: 10.1093/jac/5.2.201
![]() |
[46] | Manaia CM, Macedo G, Fatta-kassinos D, et al. (2016) Antibiotic resistance in urban aquatic environments : can it be controlled ? 1543–1557. |
[47] | Alexander J, Hembach N, Schwartz T (2020) Evaluation of antibiotic resistance dissemination by wastewater treatment plant effluents with different catchment areas in Germany. Sci Rep 1–9. |
[48] | Fewtrell L, Bartram J (2001) Guidelines, Standards and Health : Assessment of. IWA Publ 1–431. |
[49] |
Chen X, Yin H, Li G, et al. (2019) Antibiotic-resistance gene transfer in antibiotic-resistance bacteria under different light irradiation: Implications from oxidative stress and gene expression. Water Res 149: 282–291. doi: 10.1016/j.watres.2018.11.019
![]() |
1. | Thabang B. M. Mosaka, John O. Unuofin, Michael O. Daramola, Chedly Tizaoui, Samuel A. Iwarere, Inactivation of antibiotic-resistant bacteria and antibiotic-resistance genes in wastewater streams: Current challenges and future perspectives, 2023, 13, 1664-302X, 10.3389/fmicb.2022.1100102 | |
2. | Willis Gwenzi, Nyashadzashe Ngaza, Jerikias Marumure, Zakio Makuvara, Morleen Muteveri, Isaac Nyambiya, Tendai Musvuugwa, Nhamo Chaukura, 2023, Chapter 7, 978-3-031-23795-9, 107, 10.1007/978-3-031-23796-6_7 | |
3. | Gracie Hornsby, Temitope D. Ibitoye, Shivaramu Keelara, Angela Harris, Validation of a modified IDEXX defined-substrate assay for detection of antimicrobial resistant E. coli in environmental reservoirs, 2023, 25, 2050-7887, 37, 10.1039/D2EM00189F | |
4. | Iwona Beata Paśmionka, Karol Bulski, Piotr Herbut, Elżbieta Boligłowa, Frederico Márcio C. Vieira, Gabriela Bonassa, Marina Celant De Prá, Marcelo Bortoli, Evaluation of the Effectiveness of the Activated Sludge Process in the Elimination Both ATB-Resistant and ATB-Susceptible E. coli Strains, 2021, 14, 1996-1073, 5868, 10.3390/en14185868 | |
5. | Eliezer Ladeia Gomes, Giovanna Velicka Monteiro Vallio, Laura Plazas Tovar, Lucas Bragança de Oliveira, Luiz Sidney Longo Junior, Sarah Isabel Pinto Monteiro do Nascimento Alves, Vinícius Matheus Silva Macedo, Romilda Fernandez‐Felisbino, 2024, 9781119904809, 97, 10.1002/9781119904991.ch4 |
Date | pH | T (℃) | TSS (mg/L) | VSS (mg/L) | VSS/TSS (%) | |
Biological reactor of WWTP1 | 23/03/15 | 7.18 | 16.8 | 2030 | 1810 | 89.2 |
07/04/15 | 7.29 | 19.2 | 2080 | 1900 | 91.3 | |
10/04/15 | 7.22 | 18.2 | 2160 | 1960 | 90.7 | |
20/04/15 | 7.34 | 18.9 | 1950 | 1700 | 87.2 | |
27/04/15 | 7.05 | 18.7 | 1710 | 1550 | 90.6 | |
04/05/15 | 7.11 | 18.7 | 1310 | 1230 | 93.9 | |
11/05/15 | 7.09 | 21.0 | 1560 | 1470 | 94.2 | |
18/05/15 | 7.07 | 22.1 | 1240 | 1190 | 96.0 | |
25/05/15 | 7.16 | 22.8 | 1250 | 1170 | 93.6 | |
01/06/15 | 7.27 | 22.1 | 1420 | 1350 | 95.1 | |
08/06/15 | 7.33 | 23.9 | 1410 | 1300 | 92.2 | |
15/06/15 | 7.29 | 21.7 | 1660 | 1510 | 91.0 | |
Biological reactor of WWTP2 | 23/03/15 | 6.65 | 15.5 | 1040 | 1030 | 99.0 |
07/04/15 | 7.06 | 16.9 | 970 | 960 | 99.0 | |
10/04/15 | 6.85 | 18.5 | 980 | 870 | 88.8 | |
20/04/15 | 7.32 | 15.0 | 2170 | 1850 | 85.3 | |
27/04/15 | 6.98 | 16.4 | 1970 | 1680 | 85.3 | |
04/05/15 | 6.12 | 18.2 | 1720 | 1490 | 86.6 | |
11/05/15 | 6.51 | 19.1 | 1930 | 1710 | 88.6 | |
18/05/15 | 6.47 | 18.7 | 1550 | 1380 | 89.0 | |
25/05/15 | 6.52 | 20.7 | 1790 | 1560 | 87.2 | |
01/06/15 | 7.12 | 21.0 | 1840 | 1570 | 85.3 | |
08/06/15 | 7.24 | 20.2 | 1550 | 1360 | 87.7 | |
15/06/15 | 7.38 | 20.3 | 1930 | 1720 | 89.1 |
Date | pH | T (℃) | TSS (mg/L) | VSS (mg/L) | VSS/TSS (%) | |
Biological reactor of WWTP1 | 23/03/15 | 7.18 | 16.8 | 2030 | 1810 | 89.2 |
07/04/15 | 7.29 | 19.2 | 2080 | 1900 | 91.3 | |
10/04/15 | 7.22 | 18.2 | 2160 | 1960 | 90.7 | |
20/04/15 | 7.34 | 18.9 | 1950 | 1700 | 87.2 | |
27/04/15 | 7.05 | 18.7 | 1710 | 1550 | 90.6 | |
04/05/15 | 7.11 | 18.7 | 1310 | 1230 | 93.9 | |
11/05/15 | 7.09 | 21.0 | 1560 | 1470 | 94.2 | |
18/05/15 | 7.07 | 22.1 | 1240 | 1190 | 96.0 | |
25/05/15 | 7.16 | 22.8 | 1250 | 1170 | 93.6 | |
01/06/15 | 7.27 | 22.1 | 1420 | 1350 | 95.1 | |
08/06/15 | 7.33 | 23.9 | 1410 | 1300 | 92.2 | |
15/06/15 | 7.29 | 21.7 | 1660 | 1510 | 91.0 | |
Biological reactor of WWTP2 | 23/03/15 | 6.65 | 15.5 | 1040 | 1030 | 99.0 |
07/04/15 | 7.06 | 16.9 | 970 | 960 | 99.0 | |
10/04/15 | 6.85 | 18.5 | 980 | 870 | 88.8 | |
20/04/15 | 7.32 | 15.0 | 2170 | 1850 | 85.3 | |
27/04/15 | 6.98 | 16.4 | 1970 | 1680 | 85.3 | |
04/05/15 | 6.12 | 18.2 | 1720 | 1490 | 86.6 | |
11/05/15 | 6.51 | 19.1 | 1930 | 1710 | 88.6 | |
18/05/15 | 6.47 | 18.7 | 1550 | 1380 | 89.0 | |
25/05/15 | 6.52 | 20.7 | 1790 | 1560 | 87.2 | |
01/06/15 | 7.12 | 21.0 | 1840 | 1570 | 85.3 | |
08/06/15 | 7.24 | 20.2 | 1550 | 1360 | 87.7 | |
15/06/15 | 7.38 | 20.3 | 1930 | 1720 | 89.1 |