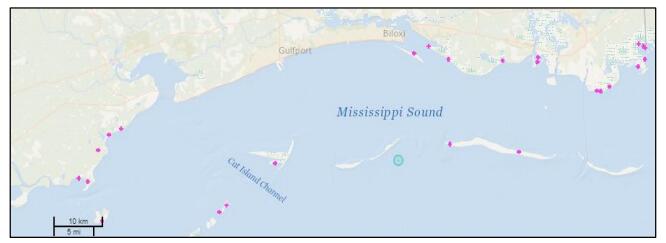
Citation: Joseph A. Kazery, Dayakar P. Nittala, H. Anwar Ahmad. Meteorological effects on trace element solubility in Mississippi coastal wetlands[J]. AIMS Environmental Science, 2019, 6(1): 1-13. doi: 10.3934/environsci.2019.1.1
[1] | Sandrine Chifflet, Marc Tedetti, Hana Zouch, Rania Fourati, Hatem Zaghden, Boubaker Elleuch, Marianne Quéméneur, Fatma Karray, Sami Sayadi . Dynamics of trace metals in a shallow coastal ecosystem: insights from the Gulf of Gabès (southern Mediterranean Sea). AIMS Environmental Science, 2019, 6(4): 277-297. doi: 10.3934/environsci.2019.4.277 |
[2] | Jon Knight, Pat Dale, Patrick Dwyer, Sam Marx . A conceptual approach to integrate management of ecosystem service and disservice in coastal wetlands. AIMS Environmental Science, 2017, 4(3): 431-442. doi: 10.3934/environsci.2017.3.431 |
[3] | Swatantra R. Kethireddy, Grace A. Adegoye, Paul B. Tchounwou, Francis Tuluri, H. Anwar Ahmad, John H. Young, Lei Zhang . The status of geo-environmental health in Mississippi: Application of spatiotemporal statistics to improve health and air quality. AIMS Environmental Science, 2018, 5(4): 273-293. doi: 10.3934/environsci.2018.4.273 |
[4] | Beth Ravit, Frank Gallagher, James Doolittle, Richard Shaw, Edwin Muñiz, Richard Alomar, Wolfram Hoefer, Joe Berg, Terry Doss . Urban wetlands: restoration or designed rehabilitation?. AIMS Environmental Science, 2017, 4(3): 458-483. doi: 10.3934/environsci.2017.3.458 |
[5] | Africa de la Hera, Emilio Custodio Gimena, Àngel García Cortés . Evaluating ecosystem services and drivers of change in Spanish groundwater-related wetlands included in the Ramsar Convention. AIMS Environmental Science, 2017, 4(2): 232-250. doi: 10.3934/environsci.2017.2.232 |
[6] | Hamdy E. Nour, Fatma Ramadan, Nouf El Shammari, Mohamed Tawfik . Status and contamination assessment of heavy metals pollution in coastal sediments, southern Kuwait. AIMS Environmental Science, 2022, 9(4): 538-552. doi: 10.3934/environsci.2022032 |
[7] | Md. Saiful Islam, Md. Habibullah-Al-Mamun . Accumulation of trace elements in sediment and fish species of Paira River, Bangladesh. AIMS Environmental Science, 2017, 4(2): 310-322. doi: 10.3934/environsci.2017.2.310 |
[8] | Maryem EL FAHEM, Abdellah BENZAOUAK, Habiba ZOUITEN, Amal SERGHINI, Mohamed FEKHAOUI . Hydrogeochemical assessment of mine water discharges from mining activity. Case of the Haut Beht mine (central Morocco). AIMS Environmental Science, 2021, 8(1): 60-85. doi: 10.3934/environsci.2021005 |
[9] | Jian Zhang, Zhonghua Gou, Leigh Shutter . Effects of internal and external planning factors on park cooling intensity: field measurement of urban parks in Gold Coast, Australia. AIMS Environmental Science, 2019, 6(6): 417-434. doi: 10.3934/environsci.2019.6.417 |
[10] | G Mathias Kondolf, Pilar Lopez-Llompart . National-local land-use conflicts in floodways of the Mississippi River system. AIMS Environmental Science, 2018, 5(1): 47-63. doi: 10.3934/environsci.2018.1.47 |
Estuaries are coastal wetlands that serve a variety of roles from being nurseries for marine species to chemical sink. As a nursery, estuaries house larval fish and crustaceans, some of which are of economic importance and a primary sources of protein for many populations. Game fish such as red drum, seatrout, and snapper are fished recreationally and also commercially harvested along with white shrimp, blue crabs, oysters, and many more [1]. Seafood, being a critical food resource is needed to be monitored in estuaries for potential pollutants as they may accumulate in marine organisms and passed on to humans [2,3]. Trace elements (TE) like arsenic (As), selenium (Se), boron (B), and heavy metals like lead (Pb), cadmium (Cd), copper (Cu), and zinc (Zn) are pollutants that have a wide range of toxicity to many organisms. Some are essential to our growth and development at low concentrations but harmful at higher doses, while others are highly toxic even at smaller doses. Tabelin et al. reviewed the sources, modes of enrichment, mechanisms of release, and mitigation strategies for these hazardous and toxic inorganic elements in naturally contaminated rocks [4]. Whalley et al. reported substantial amounts of arsenic (5 to 1740 µg/kg) in the sediments around estuaries and sequestration from seawater can be considered as one of the main mechanism by which arsenic, boron, and selenium are enriched on the sedimentary rocks [5,6]. Wetlands, such as estuaries, can act as a geochemical trap that bond pollutants to the sediments [7] thereby reducing their bioavailability. According to Peterson et al. a basic understanding of a wetland's specific hydrology, precipitation, surface runoff, and flooding is the most essential understanding to the management of specific sites [8,9]. More recently it has been observed that the Mississippi-Atchafalaya River system acts as a sink for many TEs. This stacking of TEs from agriculture, industry, urban runoff, or river discharge will also affect the Northern Gulf of Mexico area [10].
Much work has been done on the kinetics of specific TEs especially the pollution with chemical contaminants found in estuaries and sediments [11] and their effects of salinity under oxidized or reduced conditions [12,13]. Other studies focused on the bioavailability of TEs in relation to sediment types or particulate variations through typical laboratory tests [14,15]. The bioavailability of trace elements in the estuaries is a complex phenomenon and is affected by many factors, such as chemical and mineralogy composition of soils and sediments including their solid phase partitioning along with the effect of salinity, pH, organic matter, and redox conditions [16,17]. Interaction of rainwater with pollutants in storm water [18], acid-drainage [19], and microbial activity [20] were typically studied on kinetics at the molecular level. There is little information available on the direct effects of meteorological conditions on TEs bioavailability at the ecosystem level particularly in Mississippi (MS) estuaries. This approach of direct measurements allow us to have a clear understanding of environmental conditions using empirical data [21]. The current research seeks to determine the overall effect of rainfall, drought intensities, and storm data on TEs binding to soil-sediments and its significance at the ecosystem level. It is hypothesized that the weather conditions such as the presence of rain, rainfall variations, drought intensity and storms affects the concentrations of soil-bound As, Cd, Cr, Pb, and Zn either by chemical redox conditions caused by inundated sediments or by precipitation reactions that compete for sorption sites in the soil due to changes in salinity.
Data on the trace elements was acquired from NOAA's Data Integration Visualization Exploration and Reporting (DIVER) system, which collects chemical contaminant data for the Gulf of Mexico and other geographic regions. Analysis on DIVER system's environmental data adds uniqueness by evaluating various hazardous and toxic inorganic elements that could pose very serious problems in Mississippi gulf coast marsh habitats and estuaries. Related meteorological information and weather data were collected from NOAA's National Centers for Environmental Information (NCEI). Drought information was collected from the U.S. Drought Monitor database, which stores weekly drought data. The study samples for 2010 and 2011 were selected based on the location and collection matrix. All samples were documented to be surface-soil sediments from marsh habitats along Mississippi's coastline and were collected by a core sampler from depths 1–10 cm (Figure 1). A total of 49 samples each for As, Cd, Cr and 181 samples each for Pb, and Zn were collected and distributed to various private and public laboratories to determine contamination chemistry and composition. Analyses were conducted by inductively coupled plasma- atomic emission spectrometry, mass spectrometry, and atomic absorption spectrometry following EPA protocols, SW6010B, SW6020, HY017, and 200.7. Detection limits varied based on lab, analyte, and instrument ranging from 0.012–2.5 mg/kg, 0.004–1 mg/kg, 0.01–1 mg/kg, and nearly zero to 0.35 and 0.78 mg/kg for As, Cd, Cr, Pb, and Zn respectively. Data on precipitation were collected from several weather stations along the Mississippi Gulf Coast: Biloxi (Station USC00220792), Gulfport Naval Center (Station USC00223671), Saucier Experimental Forest (Station USC00227840), Ocean Springs (Station USC00226484 & US1MSJC0015), Gulfport-Biloxi Airport (Station USW00093874), Pascagoula International Airport (USW00053858), and Sandhill Crane (USR0000MSAN).
Data were analyzed using SAS v. 9.4 and graphs were created using Microsoft Excel for consistency. The mean precipitation was calculated for multiple rainfall events occurring the same day at different stations. The mean values for daily precipitation rain intensity errors were masked for over or underestimations [22]. Due to non-Gaussian nature of precipitation, data were transformed to log (x + 1) for positive skewness and to remove the heterogeneity [23,24] thus making the data to be normally distributed. Soil samples were collected by several cruises between May and October of 2011. The mean TE concentrations were calculated for multiple samples across the Mississippi coast for the same day. For each TE concentration, data were organized into groups (rainfall and no rainfall) in order to determine the effect of rain [25]. Data on drought (obtained from the National Drought Mitigation Center's (NDMC)) were categorized on a scale of 1–6 as non-drought and abnormally dry to exceptional drought conditions. This study also included normal weather, thunderstorm winds, coastal flood, marine thunderstorm, and marine winds information data.
Analysis of variance (ANOVA) was performed to examine the variations of log transformed daily rainfall, the occurrence of precipitation, drought intensity, rainfall or no rainfall, TE soil concentrations and other miscellaneous storms against time data. Correlations (r) and regression analysis were performed for rainfall and drought intensity for each TE concentrations.
A total of 166 weather observations were collected from NCEI and were analyzed. Sample collection were done from May to October for the years 2010 and 2011, with exception of Arsenic and Zinc, where sampling and chemical analysis was only conducted for one year. Arsenic soil sediment concentrations were collected in 2010 and for Zinc in 2011. The log transformed daily rainfall means were found to be 0.39 and 0.29, respectively for 2010 and 2011 (Figure 2). The yearly rainfall data were not significantly different (p > 0.05) and this may be due to precipitation amounts averaging similar volumes of rainfall between the months of May and October in Mississippi's coast. The monthly analysis of log transformed rainfall showed a significant variation (p < 0.05) with the lowest recorded in October (0.11) and highest in August (0.60). The monthly distributions for the amounts of daily precipitation reached its peak during August and declined gradually till it reached the lowest for the month of October (Figure 3).
Zinc was the only TE that displayed a strong positive correlation, r = 0.80, with daily rainfall. This strong positive correlations are due to high concentrations of Zn that were retained in the soil with increased amount of rainfall and this may be due to adsorption of Zn to soil-sediments (Figure 4). After initial rainfall, the amount of Zn-bound sediment to other soil components, such as oxidized metals or reduced sulfur has considerably increased. Decomposing organic matter or reduced metal-oxides releasing soluble Zn can be explained by oxidized metal-oxide or reduced sulfur found in other layers [26,27].
There was no difference in the amount of daily rainfall data between 2010 and 2011. This resulted in non-significant difference in the yearly comparisons for As and Zn. Statistical analysis showed no significant variation between TE soil concentrations and occurrences of rainfall.
Drought data over the two sampling years exhibited a significant difference across every month (Figure 5). Drought categories included: No Drought (1), Abnormally Dry (2), Moderate Drought (3), Severe Drought (4), Extreme Drought (5), and Exceptional Drought (6). Droughts were more frequent and more intense on the Mississippi Coast in 2011 during the summer months ending in September. In 2010 the only month of the sampling period burdened by drought was October. Arsenic and Zinc were not observed to be affected with dry conditions. This could be due to less drought intensity prevailing during the sampling period in 2010. Significant variations for Cd, Cr, and Pb were observed among each drought intensity sampling periods. Both Cd and Cr concentrations in the soil exhibit a negative correlation with a mean of 28.6 and 29.3 µg/kg, during Non-drought conditions and with a mean of 8.7 µg/kg during Extreme drought conditions (Figure 6). Lead (Pb) exhibited a positive correlation for drought intensities with a mean of 12.7 µg/kg during Non-drought and 45.8 µg/kg in Extreme drought conditions. Cd and Cr concentrations in the soil showed significant variations during drier weather conditions. Pb concentrations appears to be minimal during normal conditions but showed an increase in values as the drought conditions increased. This was further observed by Pearson coefficients of −0.63 for Cd, −0.27 for Cr, and 0.63 for Pb, and were all found to be significant at p < 0.05. Analysis on drought conditions included (1) No Drought, (2) Abnormally dry, & (5) Extreme Drought conditions as the other drought intensities were not observed during that specific sampling time.
The increase of Cd and Cr during Non-drought conditions could have been due to rain events that cleansed the accumulated contaminants and other pollutants [28,29]. There was a significant temporal difference for Cd between the two sampling years and this could have been due to a more intense droughts in 2011. Dissolved organic matter as well as reduced compounds may leach through the sediment during dry conditions there by releasing sequestered Cd, which in turn percolates further into the soil with the changing ground water level [6,30,31,32]. Highest concentration was observed for Pb during dry seasons rather than wet conditions whereas Cr illustrated a reverse phenomenon [8,33]. However, if sediment was submerged during the dry periods increase salinity may enhance the mobility of Cd forming stable chloride complexes [34].
Miscellaneous storm data that represented extreme storm events of high magnitude collected during the sampling periods of 2010 and 2011 were analyzed. Cadmium, Pb, and Zn demonstrated significant variability between normal conditions, thunderstorms with high winds, marine storms, high marine winds, and coastal flooding. Storm data variations represent a typical normal weather to rain events where thunderstorm winds can cause either extreme high tides or low tides. Coastal floods would had heavy wave actions and seawater intrusion due to Marine thunderstorms and Marine winds [33,35]. For Cd, there was a significant difference between Normal conditions, Thunderstorm winds, and Marine winds averaging 27.1, 22.8, 30.0 µg/kg, respectively (Figure 7). The results showed lowest mean values for thunderstorm winds, which was contrary to other studies, and under saturated water conditions Cd precipitates with other soil components such as sulfides [36,37,38,39].
Chromium showed an opposite effect when compared to Cd (Figure 8). Lead had the mean of 24.0 µg/kg during thunderstorm conditions indicating that the soil was affected by rainwater. Marine storms, winds, & coastal floods brings in seawater, which increase the mobility of many TEs [32,38]. The amount of seawater exposure from these Coastal floods, Marine storms, and Marine winds were shown to effect Pb concentrations in sediments, with the mean values of 11.4, 10.0, 11.0 µg/kg respectively (Figure 9) [27].
Increased levels in salinity typically promoted desorption from sediment. Sediment bound Pb had been observed to have a less linear relationship with seawater and demonstrates different behaviors at different salinities by forming complexes with chlorides from salt water [4,40]. As for Zn, a mean of 556.7 µg/kg was observed during Thunderstorms with high winds and 121.0 µg/kg during normal conditions (Figure 10).
There was a significant variation between the months for the concentrations of Cd, Cr, and Pb, over the sampling periods in 2010 and 2011 (Figure 11). Arsenic remained relatively stable in the sediment averaging 21.09 µg/kg. Coastal soils were higher in Cd and Cr concentrations in 2010 (28.89 µg/kg for Cd and 28.91 µg/kg for Cr) compared to 2011 (both 9.16 µg/kg). Precipitation was similar as the drought intensities demonstrated strong relationships between the two sampling years. Lower levels of Cd was observed during May (24.38 µg/kg) and October (8.89 µg/kg) compared to the other months that had the mean range from 30.03 to 31.11 µg/kg. Chromium (Cr) exhibited its highest soil concentration in June with 38.29 µg/kg and lowest in October with 15.56 µg/kg. This was likely due to intense drought conditions, with exception of July and August, which showed no significant difference for 2010 sampling period. The highest Pb concentration was observed in October (33.33 µg/kg) and the lowest during June to September (10.56–12.05 µg/kg) with r = 0.63. Zinc had the highest mean in May (199.60 µg/kg) during Thunderstorms with high winds, and the lowest in October (66.96 µg/kg). This increase in sediment-bound Zn suggests an enrichment from anthropogenic input from either point or nonpoint sources.
This study was aimed at determining the effects of meteorological conditions, such as rainfall, droughts, and storms, on TEs at the ecosystem level. The results reported in this study were derived from the data collected only during the sampling periods in 2010 and 2011. We concluded that Arsenic was not affected by any of the studied weather conditions. Zinc was the only TE that was affected by the amount of rainfall demonstrating a strong positive correlation (0.80) that may be due to adsorption to other components found in the soil. During Thunderstorms, both Pb and Zn concentrations increased in the soil. This may have been due to storm runoff from roads or other human impacted areas to the soils that act as a chemical sink by redox processes. TEs were also greatly affected by drought intensities, either in a positive correlation (Pb, 0.63) or negative correlation (Cd, −0.63; Cr, −0.27). It was observed that concentrations of Cd and Cr were decreased while the concentrations of Pb increased in soils during our sampling periods in 2010 and 2011. Arsenic and Zinc were collected only for one of the sampling years and monthly observations exhibited stable concentration of Arsenic while concentrations of Zinc were decreased from May to October.
This study observed coastal soils entrapping of TEs (Pb and Zn) while releasing other TEs (Cd and Cr) under various weather conditions. This release of TEs become bioavailable to the local aquatic organisms such as bivalves, crustaceans, and larval fish. Such accumulation raises concern, since chronic exposure had been shown to affect human health. Overall, this empirical study may offer a better insight of TEs dynamics and their effects on the environment and ecosystem.
This work was supported by the National Institute on Minority Health and Health Disparities of the National Institutes of Health under Award Number G12MD007581. The content is solely the responsibility of the authors and does not represent the official views of the National Institutes of Health.
The authors declare no conflict of interest.
The study focused only on Gulf Coast of Mississippi, USA and therefore, care must be taken when extrapolating this data to other coastal regions.
The transport and distribution of TEs along the Gulf Coast needs further monitoring. Analyzing TEs in aquatic organisms of human interest will assist in determining potential risk to the environment and public health.
[1] | Shervette V (2006) Assessment of Estuarine Habitats for Resident and Estuarine-dependent Species: Tools for Conservation and Management. PhD diss., Texas A & M University, 1–187. |
[2] | Paalman MAA (1997) Processes affecting the distribution and speciation of heavy metals in the Rhine/Meuse Estuary. Published by Utrecht University, 148: 1–149. |
[3] |
Mok JS, Kwon JY, Son KT, et al. (2014) Contents and Risk Assessment of Heavy Metals in Marine Invertebrates from Korean Coastal Fish Markets. J Food Protect 77: 1022–1030. doi: 10.4315/0362-028X.JFP-13-485
![]() |
[4] |
Tabelin CB, Igarashi T, Villacorte-Tabelin M, et al. (2018) Arsenic, selenium, boron, lead, cadmium, copper, and zinc in naturally contaminated rocks: A review of their sources, modes of enrichment, mechanisms of release, and mitigation strategies. Sci Total Environ 645: 1522–1553. doi: 10.1016/j.scitotenv.2018.07.103
![]() |
[5] |
Whalley C, Rowlatt S, Bennett M, et al. (1999) Total Arsenic in Sediments from the Western North Sea and the Humber Estuary. Mar Pollut Bull 38: 394–400. doi: 10.1016/S0025-326X(98)00158-1
![]() |
[6] |
Xie X, Wang Y, Ellis A, et al. (2011) The sources of geogenic arsenic in aquifers at Datong basin, northern China: Constraints from isotopic and geochemical data. J Geochem Explor 110: 155–166. doi: 10.1016/j.gexplo.2011.05.006
![]() |
[7] |
Sun Z, Mou X, Tong C, et al. (2015) Spatial variations and bioaccumulation of heavy metals in intertidal zone of the Yellow River estuary, China. Catena 126: 43–52. doi: 10.1016/j.catena.2014.10.037
![]() |
[8] | Peterson M, Waggy G, Woodrey M, et al. (2007) Grand Bay national estuarine research reserve: An ecological characterization. Moss Point, Mississippi. Silver Spring, MD 20910, 1–268. |
[9] |
McComb JQ, Han FX, Rogers C, et al. (2015) Trace elements and heavy metals in the Grand Bay National Estuarine Reserve in the northern Gulf of Mexico. Mar Pollut Bull 99: 61–69. doi: 10.1016/j.marpolbul.2015.07.062
![]() |
[10] |
Reiman JH, Xu YJ, He S, et al. (2018) Metals geochemistry and mass export from the Mississippi-Atchafalaya River system to the Northern Gulf of Mexico. Chemosphere 205: 559–569. doi: 10.1016/j.chemosphere.2018.04.094
![]() |
[11] |
Hu XF, Jiang Y, Shu Y, et al. (2014) Effects of mining wastewater discharges on heavy metal pollution and soil enzyme activity of the paddy fields. J Geochem Explor 147: 139–150. doi: 10.1016/j.gexplo.2014.08.001
![]() |
[12] |
Du Laing G, Vandecasteele B, De Grauwe P, et al. (2007) Factors affecting metal concentrations in the upper sediment layer of intertidal reedbeds along the river Scheldt. J Environ Monitor 9: 449–455. doi: 10.1039/b618772b
![]() |
[13] |
Du Laing G, Meers E, Dewispelaere M, et al. (2009) Heavy metal mobility in intertidal sediments of the Scheldt estuary: Field monitoring. Sci Total Environ 407: 2919–2930. doi: 10.1016/j.scitotenv.2008.12.024
![]() |
[14] |
Hatje V, Payne TE, Hill DM, et al. (2003) Kinetics of trace element uptake and release by particles in estuarine waters: Effects of pH, salinity, and particle loading. Environ Int 29: 619–629. doi: 10.1016/S0160-4120(03)00049-7
![]() |
[15] | Turner A (2000) Trace Metal Contamination in Sediments from U.K. Estuaries: An Empirical Evaluation of the Role of Hydrous Iron and Manganese Oxides. Estuar Coast Shelf Sci 50: 355–371. |
[16] |
Ouddane B, Skiker M, Fischer JC, et al. (1999) Distribution of iron and manganese in the Seine river estuary: Approach with experimental laboratory mixing. J Environ Monitor 1: 489–496. doi: 10.1039/a903721g
![]() |
[17] |
Wong VNL, Johnston SG, Burton ED, et al. (2010) Seawater causes rapid trace metal mobilisation in coastal lowland acid sulfate soils: Implications of sea level rise for water quality. Geoderma 160: 252–263. doi: 10.1016/j.geoderma.2010.10.002
![]() |
[18] |
Charters FJ, Cochrane TA, O'Sullivan AD (2016) Untreated runoff quality from roof and road surfaces in a low intensity rainfall climate. Sci Total Environ 550: 265–272. doi: 10.1016/j.scitotenv.2016.01.093
![]() |
[19] |
Nordstrom DK (2009) Acid rock drainage and climate change. J Geochem Explor 100: 97–104. doi: 10.1016/j.gexplo.2008.08.002
![]() |
[20] |
Shehane SD, Harwood VJ, Whitlock JE, et al. (2005) The influence of rainfall on the incidence of microbial faecal indicators and the dominant sources of faecal pollution in a Florida river. J Appl Microbiol 98: 1127–1136. doi: 10.1111/j.1365-2672.2005.02554.x
![]() |
[21] | Alloway BJ, (2013) Sources of Heavy Metals and Metalloids in Soils, In: Alloway BJ, editor. Dordrecht: Springer Netherlands, 11–50. |
[22] |
Mearns LO, Giorgi F, McDaniel L, et al. (1995) Analysis of daily variability of precipitation in a nested regional climate model: Comparison with observations and doubled CO2 results. Global Planet Change 10: 55–78. doi: 10.1016/0921-8181(94)00020-E
![]() |
[23] |
Hatje V, Birch GF, Hill DM (2001) Spatial and Temporal Variability of Particulate Trace Metals in Port Jackson Estuary, Australia. Estuarine Coastal Shelf Sci 53: 63–77. doi: 10.1006/ecss.2001.0792
![]() |
[24] |
Katz RW (1983) Statistical Procedures for Making Inferences about Precipitation Changes Simulated by an Atmospheric General Circulation Model. J Atmos Sci 40: 2193–2201. doi: 10.1175/1520-0469(1983)040<2193:SPFMIA>2.0.CO;2
![]() |
[25] |
Ragosta M, Caggiano R, D'Emilio M, et al. (2002) Source origin and parameters influencing levels of heavy metals in TSP, in an industrial background area of Southern Italy. Atmos Environ 36: 3071–3087. doi: 10.1016/S1352-2310(02)00264-9
![]() |
[26] |
Chuan MC, Shu GY, Liu JC (1996) Solubility of heavy metals in a contaminated soil: Effects of redox potential and pH. Water Air Soil Pollut 90: 543–556. doi: 10.1007/BF00282668
![]() |
[27] |
Huerta-Diaz MA, Tessier A, Carignan R (1998) Geochemistry of trace metals associated with reduced sulfur in freshwater sediments. Appl Geochem 13: 213–233. doi: 10.1016/S0883-2927(97)00060-7
![]() |
[28] | Byrnes MR, Davis JRA, Kennicutt IIMC, et al. (2017) Habitats and Biota of the Gulf of Mexico: Before the Deepwater Horizon Oil Spill. Volume 1: Water Quality, Sediments, Sediment Co ntaminants, Oil and Gas Seeps, Coastal Habitats, Offshore Plankton and Benthos, and Shellfish. 948. |
[29] | Ideriah TJK, Ikpe FN, Nwanjoku FN (2013) Distribution and Speciation of Heavy Metals in Crude Oil Contaminated Soils from Niger Delta, Nigeria. World Environ 3: 18–28. |
[30] |
Stephens SR, Alloway BJ, Parker A, et al. (2001) Changes in the leachability of metals from dredged canal sediments during drying and oxidation. Environ Pollut 114: 407–413. doi: 10.1016/S0269-7491(00)00231-1
![]() |
[31] |
Church TM, Bernat M, Sharma P (1986) Distribution of natural uranium, thorium, lead, and polonium radionuclides in tidal phases of a Delaware salt marsh. Estuaries 9: 2–8. doi: 10.2307/1352187
![]() |
[32] |
Li QS, Liu YN, Du YF, et al. (2011) The behavior of heavy metals in tidal flat sediments during fresh water leaching. Chemosphere 82: 834–838. doi: 10.1016/j.chemosphere.2010.11.026
![]() |
[33] |
Peterson ML, Carpenter R (1986) Arsenic distributions in porewaters and sediments of Puget Sound, Lake Washington, the Washington coast and Saanich Inlet, B.C. Geochim Cosmochim Ac 50: 353–369. doi: 10.1016/0016-7037(86)90189-4
![]() |
[34] | Chu B, Chen X, Li Q, et al. (2014) Effects of salinity on the transformation of heavy metals in tropical estuary wetland soil. Chem Ecol 31: 186–198. |
[35] |
Charlatchka R, Cambier P (2000) Influence of reducing conditions on solubility of trace metals in contaminated soils. Water Air Soil Pollut 118: 143–168. doi: 10.1023/A:1005195920876
![]() |
[36] |
Du Laing G, De Vos R, Vandecasteele B, et al. (2008) Effect of salinity on heavy metal mobility and availability in intertidal sediments of the Scheldt estuary. Estuar Coast Shelf Sci 77: 589–602. doi: 10.1016/j.ecss.2007.10.017
![]() |
[37] |
Acosta JA, Jansen B, Kalbitz K, et al. (2011) Salinity increases mobility of heavy metals in soils. Chemosphere 85: 1318–1324. doi: 10.1016/j.chemosphere.2011.07.046
![]() |
[38] | Millward GE, Liu YP (2003) Modelling metal desorption kinetics in estuaries. Sci Total Environ 314–316: 613–623. |
[39] |
Mearns LO, Giorgi F, McDaniel L, et al. (1995) Analysis of daily variability of precipitation in a nested regional climate model: Comparison with observations and doubled CO2 results. Global Planet Change 10: 55–78. doi: 10.1016/0921-8181(94)00020-E
![]() |
[40] |
Kraepiel AML, Chiffoleau JF, Martin JM, et al. (1997) Geochemistry of trace metals in the Gironde estuary. Geochim Cosmochim Ac 61: 1421–1436. doi: 10.1016/S0016-7037(97)00016-1
![]() |
1. | Banjo Ayobami Akinyemi, Chunping Dai, Luffa cylindrical fibre as a natural reinforcement for cement composites: A review, 2022, 11, 2165-0373, 297, 10.1080/21650373.2021.1952658 | |
2. | A Budhiyanto, A Oktavianus, B Tedjokusumo, K Harsono, I T Yang, Comparison of simulation-based methods and metaheuristic optimization algorithms for optimizing window design by considering daylighting and heat transfer in a tropical region of Indonesia, 2021, 907, 1755-1307, 012016, 10.1088/1755-1315/907/1/012016 | |
3. | Aline Varella Rodrigues, Bruno Seiki Domingos Onishi, Sidney José Lima Ribeiro, Facile Formation of Sulfurized Nanorod‐Like ZnO/Zn(OH)2 and Hierarchical Flower‐Like γ‐Zn(OH)2/ϵ‐Zn(OH)2 from a Green Synthesis and Application as Luminescent Solar Concentrator, 2023, 24, 1439-4235, 10.1002/cphc.202300134 |