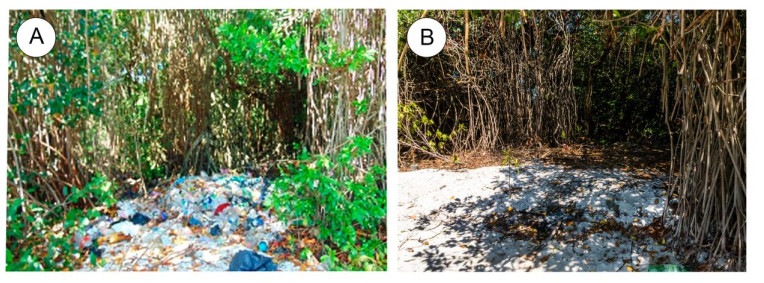
Mangrove ecosystems serve as critical natural filters and retention points for continental waste entering the sea, making them primary accumulation sites for solid waste, such as plastics. These plastics undergo transformation from macroplastics to microplastics through weathering. We investigated the resistance and degradation process of plastic waste in the mangrove swamp of the Botanical Garden at the Autonomous University of Carmen, focusing specifically on its transformation into microplastics over time. By quantifying the collected waste and identifying by-products, we evaluated the degradation process of these materials, which impact the environment and become microplastics. Our analysis provided a detailed approximation of this transformation process through the quantification and examination of collected waste and its by-products. We explored the degradation dynamics and their environmental ramifications. Using data derived from collected waste samples, we estimated an annual generation of 12,300 kg of plastic waste and 1,351 kg of microplastics. This latter figure represents 11% of the total annual plastic waste, posing a significant threat to the biodiversity and ecological balance of mangroves. Our findings underscore the urgent need for more effective and sustainable waste management practices within these critical ecosystems. Furthermore, this research emphasizes the importance of continuous monitoring and investigation of degradation processes to better understand the long-term effects of plastic pollution on mangrove environments and to develop effective strategies to mitigate its adverse impacts.
Citation: Laines Canepa José Ramón, Sosa Olivier José Aurelio, Padilla-Rivera Alejandro, Hernández Barajas José Roberto, Morales-Rodríguez Neil Ebeth Meled, Burelo Ramos Carlos Manuel, Espinosa de los Monteros Alejandra Elvira, Núñez-Nogueira Gabriel. Transformation of plastic debris to microplastics: An approximate analysis of mangrove environments[J]. Urban Resilience and Sustainability, 2024, 2(4): 348-364. doi: 10.3934/urs.2024018
[1] | Sunny Han Han, Yujing Li, Peiheng Yu . What makes a successful industrial heritage park?—China's experience based on the ecosystem cultural services perspective. Urban Resilience and Sustainability, 2024, 2(2): 93-109. doi: 10.3934/urs.2024006 |
[2] | Heitor Castro Brasiel, Danielli Araújo Lima . Clustered-map probabilistic cellular automata for fire propagation in the Brazilian Cerrado with heterogeneous vegetation and wind interference. Urban Resilience and Sustainability, 2024, 2(1): 45-75. doi: 10.3934/urs.2024004 |
[3] | Fairuz Amanina, Zul Ilham . Placemaking strategies in greening Universiti Malaya Main Library. Urban Resilience and Sustainability, 2024, 2(1): 76-92. doi: 10.3934/urs.2024005 |
[4] | Tracey Skillington, Johanna Marie Kirsch . Assessing inequalities in access to the city’s green and blue spaces through the experiences of its residents. Urban Resilience and Sustainability, 2024, 2(3): 272-288. doi: 10.3934/urs.2024014 |
[5] | Fasil Kebede Tesfaye, Ayitenew Mogninet Getaneh, Mequanint Birhan . The management of sustainable energy sources in the mechanical sector. Urban Resilience and Sustainability, 2023, 1(2): 138-145. doi: 10.3934/urs.2023010 |
[6] | Ana Perić . Reframing collaborative planning theory and practice. Urban Resilience and Sustainability, 2024, 2(4): 365-370. doi: 10.3934/urs.2024019 |
[7] | Aibin Yan, Dinghan Zheng . Restoration and integration of the Huang Family Garden within the contemporary urban fabric of Shanghai. Urban Resilience and Sustainability, 2024, 2(1): 27-44. doi: 10.3934/urs.2024003 |
[8] | Tatyana P. Kalikhman . The economic situation and ways to solve the environmental problems in the city of Ust-Kut. Urban Resilience and Sustainability, 2024, 2(2): 203-214. doi: 10.3934/urs.2024010 |
[9] | Cheikh Cisse . At the crossroads of datas: Using artificial intelligence for efficient and resilient planning in African cities. Urban Resilience and Sustainability, 2023, 1(4): 309-313. doi: 10.3934/urs.2023019 |
[10] | Prakeerth Anam, B. Madhusudhana Rao, SS. Asadi . Computer-based analysis for design and schedule management for commercial buildings. Urban Resilience and Sustainability, 2023, 1(1): 37-47. doi: 10.3934/urs.2023003 |
Mangrove ecosystems serve as critical natural filters and retention points for continental waste entering the sea, making them primary accumulation sites for solid waste, such as plastics. These plastics undergo transformation from macroplastics to microplastics through weathering. We investigated the resistance and degradation process of plastic waste in the mangrove swamp of the Botanical Garden at the Autonomous University of Carmen, focusing specifically on its transformation into microplastics over time. By quantifying the collected waste and identifying by-products, we evaluated the degradation process of these materials, which impact the environment and become microplastics. Our analysis provided a detailed approximation of this transformation process through the quantification and examination of collected waste and its by-products. We explored the degradation dynamics and their environmental ramifications. Using data derived from collected waste samples, we estimated an annual generation of 12,300 kg of plastic waste and 1,351 kg of microplastics. This latter figure represents 11% of the total annual plastic waste, posing a significant threat to the biodiversity and ecological balance of mangroves. Our findings underscore the urgent need for more effective and sustainable waste management practices within these critical ecosystems. Furthermore, this research emphasizes the importance of continuous monitoring and investigation of degradation processes to better understand the long-term effects of plastic pollution on mangrove environments and to develop effective strategies to mitigate its adverse impacts.
Plastic pollution has emerged as a global environmental challenge, impacting a wide array of ecosystems and species worldwide. Mangrove ecosystems, which are vital for environmental health and biodiversity, are increasingly threatened by the accumulation of plastic debris [1,2]. Studies indicate that plastic waste not only mars the natural beauty of these habitats but also poses significant risks to local flora and fauna [3,4,5,6]. However, a less explored aspect is the transformation of these plastic wastes into microplastics and their long-term impact [7,8].
Our primary objectives are to: (1) analyze the degradation process of plastic waste in the mangroves of the Botanical Garden of the Autonomous University of Carmen (UNACAR), particularly its transformation into microplastics over time; (2) understand the environmental impact of these microplastics by assessing the quantities and stages of degradation of plastic waste collected at different intervals; and (3) provide scientific data on the transformation processes of plastics in mangrove ecosystems, contributing essential insights for the design of effective and straightforward waste management strategies.
Through this research, we aim to support environmental policy-making by the authorities of the UNACAR Botanical Garden and promote sustainable practices for responsible waste management in mangrove areas often affected by indiscriminate dumping (Figure 1). By addressing these challenges, the study aspires to strengthen broader initiatives in environmental education, waste management, and the sustainable development of mangrove ecosystems.
The transformation of plastic waste into microplastics is a complex process driven by various mechanisms. The key processes involved in this transformation are detailed in Table 1.
Process | Description |
Fragmentation![]() |
Large plastic waste breaks down into fragments due to physical factors such as UV radiation, waves, wind, and mechanical abrasion. Prolonged exposure to sunlight and environmental weakens polymer bonds, forming microplastics [6,7,9,10,11]. |
Chemical Degradation![]() |
Plastics, composed of long polymer chains, degrade chemically under specific environmental conditions. Oxidation, hydrolysis and photodegradation alter the molecular structure of plastics, making them more susceptible to fragmentation [12]. |
Biological Degradation![]() |
Some microorganisms colonize the surface of plastics and partially degrade them. While biodegradation of conventional plastics is a slow, it contributes to reducing plastic size and facilitating the transformation into microplastics [12,13]. |
Weathering![]() |
Environmental factors such as temperature, humidity, and sunlight exposure cause weathering, resulting in physical and chemical changes that deteriorate plastics, making them more prone to fragmentation [14]. |
Biophysical Interactions![]() |
Biological activity, such as feeding by marine organisms, contributes to plastic fragmentation. Larger plastics may be ingested and excreted as smaller particles [12,15,16]. |
The significance of this investigation extends across a diverse spectrum of stakeholders, including environmental researchers, policymakers, mangrove ecosystem managers, and the broader community, all of whom share a growing concern about the environmental repercussions of plastic pollution. By gaining a nuanced understanding of the conversion process of plastic waste into microplastics and its subsequent environmental impacts, stakeholders are better equipped to devise and implement more effective mitigation and preservation strategies for these vital ecosystems.
Recognizing that the production of plastic waste is a direct precursor to the formation of microplastics, measuring the volume of plastic in a given locale enables the prediction of potential microplastic production. This knowledge is pivotal for assessing the scale of microplastic pollution and formulating targeted interventions to combat this escalating environmental challenge.
The designated study region is in an estuarine area 1.1 km long estuarine region within the mangrove zone of the 23 de Julio neighborhood in Ciudad del Carmen, Campeche, Mexico (Figure 2). This area is northwest of Laguna de Términos, between the geographic coordinates N 18° 38' 8.315'' W 91° 47' 19.705'' and N 18° 38' 7.274'' W 91° 46' 44.691''. It is bordered to the east by the UNACAR Botanical Garden and to the west by the Las Pilas neighborhood.
The climate in the study area is classified as warm subhumid (Aw2(w) [17]. The region experiences an average annual temperature of 27 ℃, with May being the warmest month and January the mildest. The average annual precipitation in the area is 1,447 mm, reaching a monthly average of 245 mm in September and decreasing in April with a monthly average of 22 mm [18].
The vegetation primarily consists of four mangrove species: Red mangrove (Rhizophora mangle L.), black mangrove (Avicennia germinans L.), white mangrove (Laguncularia racemosa (L.) C.F. Gaerttn), and button mangrove (Conocarpus erectus L.). Other species such as palo mulato (Bursera simaruba (L.) Sarg.), beach grape (Coccoloba uvifera L.), and black Chechen (Metopium brownie (Jacq.) Urb.) are also present.
Sampling was conducted according to the Mexican standards NMX-AA-015 [19] and NMX-AA-022 [20], which outline the procedures for sample selection, identification, and quantification of by-products present in municipal solid waste. NMX-AA-015 describes the quartering method, which aims to obtain a random sample for by-product determination and, if necessary, perform laboratory analysis.
The quartering technique involves emptying containers and bags to form a large waste pile on a flat, horizontal surface (4 m × 4 m). The waste pile is homogenized and divided into four sections (A, B, C, D). Opposing sections (A-D, B-C) are removed to reduce the pile size until a minimum of 50 kg of waste is achieved. The removed sections are used to determine the volumetric weight and composition of by-products.
NMX-AA-022 lists 26 by-products that make up municipal solid waste.
The samples obtained via quartering were quantified following this list, assigning a percentage value to each by-product relative to the total waste weight. The sum of the weights of the by-products equaled or exceeded 98% of the total waste weight.
Sampling routes were selected based on representative areas previously identified within the mangrove swamp where the accumulation of plastic waste was observed. Waste was collected systematically and repetitively along this sampling route.
Equipment and materials used for sample collection included scales, shovels, gloves, collection bags, and tape measures. Sampling was conducted systematically along predefined routes during three distinct periods over the year. Student groups participating in a two-semester course, "Integral Management of Urban Solid Waste and Special Management, " at the Universidad Juárez Autónoma de Tabasco's Environmental Engineering Program, were trained to adhere to NMX standards. The gathered waste was securely stored in plastic bags, each with a 45-gallon capacity and a material thickness of 3/1000 inches. Detailed records of the location, date, and time of each collection effort were maintained, along with relevant observations.
The collected waste was classified by material type, shape, and size, following the categories established in the NMX-AA-022 standards.
Each category was weighed using precision balance. The total weight of plastic waste collected along the sampling route was calculated and expressed in kilograms (kg). Data analysis was performed to determine patterns and temporal variations in plastic waste generation.
All procedures and results obtained during sample collection and analysis were meticulously recorded. A detailed report was prepared, encompassing the methodology, results, conclusions, and recommendations for plastic waste management in the mangrove ecosystem.
A variety of techniques have been developed to determine and characterize microplastics, including visual identification, spectroscopic methods such as Fourier-transform infrared spectroscopy (FTIR) and Raman spectroscopy, thermal analysis using thermogravimetric analysis (TGA), and pyrolysis-gas chromatography-mass spectrometry (Py-GC-MS). Chemical methods, such as pressurized fluid extraction and fluorescence staining, as well as elemental analysis, have also been utilized. The suitability of these methods varies depending on the specific type of plastic or the matrix of the samples being analyzed [21].
In this study, we focused on classifying and weighing plastic waste as the primary analytical technique. The plastics were categorized by type (PET, HDPE, PVC, LDPE, PP, PS, and others) and form (rigid or film). This methodological approach was selected for its practical relevance in assessing the degradation behavior of each plastic type under mangrove conditions. It allows us to effectively classify the collected plastic waste and estimate its contribution to the generation of microplastics in the mangrove ecosystem.
While advanced techniques offer detailed characterization, our decision to adopt this practical approach aligns with our research objectives and addresses logistical and resource constraints, particularly when working at larger scales.
The estimation of microplastic generation was based on technical literature and the following assumptions [9,22,23]:
Macro to Microplastic Conversion Rate: It is inferred that a percentage of the total weight of each type of plastic is converted into microplastics, reflecting the transformation of macroscopic plastic waste into microscopic particles due to physical, chemical, and biological processes. This conversion percentage is based on average data obtained from previous studies, adapted to the specific conditions of the mangrove. Additionally, the plastic waste leakage variable (amount of plastic entering the oceans and other environmental compartments, e.g., rivers, soil, or atmosphere) is considered. This is calculated as 7% in areas identified as hotspots—areas with high concentrations of plastic waste and higher potential for environmental impact—and 15% in worst-case conditions (WCC), corresponding to scenarios where waste management practices are minimal or non-existent, thus increasing the probability of plastic release into the environment [24]. This percentage is averaged between hotspots and WCC, resulting in 11%.
Influence of Environmental Conditions: The unique conditions of the mangrove, including humidity, temperature, solar exposure, and microbial activity, are considered to adjust the conversion rates of macro to microplastics.
The transformation percentage of macroplastics into microplastics is adjusted based on specific environmental conditions present in the mangrove ecosystem. Key factors such as average humidity levels (72%), temperatures ranging from 27 ℃ to 33 ℃, and solar radiation intensity are considered, as these significantly influence the photodegradation and fragmentation processes. Researchers (e.g., [1,9]) have found that increased humidity accelerates microbial degradation, while higher temperatures and radiation intensify photochemical reactions. Accordingly, the selected transformation rate of 11% reflects an average derived from comparable ecosystems with similar conditions.
In terms of classification by polymer, the three most relevant types are polyethylene (PE) at 30.67%, polypropylene (PP) at 23.56%, and polystyrene (PS) at 17.51% [25]. Mahidin et al. [26] mentions the types of microplastics present in the soil, weighted by type of plastic: Polyethylene (21%), polypropylene (21%), polystyrene (14%), polyvinyl chloride (14%), polyester (15%), and others (14%).
Using the weight data by plastic type and assumptions on conversion rates, the estimated amount of microplastics generated by each plastic category is calculated using the following Eq (1):
(1) |
The results estimate the amount of microplastics that each type of plastic contributes to the mangrove environment. This is discussed in the context of microplastic pollution in mangrove ecosystems and compared with data available in the literature. The relevance of these findings highlights the critical issue of microplastic pollution within these vital ecosystems.
Limitations of this estimate include uncertainties associated with the assumptions of conversion rates and the applicability of data from previous studies to the specific conditions of the mangrove studied. These factors introduce a degree of variability that must be acknowledged when interpreting the results.
Sustainable management of mangrove ecosystems is fundamental for biodiversity conservation and mitigation of environmental impacts. In the face of growing challenges such as plastic pollution and habitat degradation, it is imperative to implement a comprehensive methodology to guide environmental decision-making and sustainable policy development. This methodology should encompass environmental assessment, stakeholder analysis, implementation of concrete actions, and ongoing monitoring. Adopting a holistic and collaborative approach ensures the preservation of these valuable ecosystems for future generations.
A hotspot for debris accumulation was identified between the mangrove and urbanization boundaries at geographic coordinates N 18° 38' 8.009'' W 91° 47' 19.73'' (Figure 3). Mangrove trees were observed to the east of the study site, with debris trapped among their roots. This phenomenon was noted at multiple points along an 800-meter stretch of the riparian zone.
The sample collection stage was carried out in September and December 2022, and February 2023, with three samples taken for statistical purposes, one each month. Along the 2.1 km of the sampling route, a significant accumulation of waste, mainly plastics, was observed. Predominant plastic materials included tires, plastic bottles, bags, wrappers, and other everyday objects. Six waste collection points along the coastline were selected to systematize the collection procedure and ensure the repeatability of the data.
The observed waste not only marred the natural beauty of the mangrove but also posed a serious threat to biodiversity and ecosystem health, emphasizing the need for effective waste management measures and public awareness campaigns (Figure 4).
Collected waste was homogenized following NMX-AA-015 and classified by type of material, shape, and size, following the categories established in NMX-AA-022. Each identified by-product was weighed with precision instruments (Figure 5).
A total of 358.0 kg of waste was collected during the three sampling periods (164.7 kg, 131.6 kg, and 61.7 kg, respectively, for each sampling). This waste was separated into categories: Recyclable (including plastic waste, cardboard, paper, waxed cardboard, synthetic fibers, rubber, aluminum cans, tin cans, and glass), organic, sanitary, hazardous, electrical/electronic, and others. The total amount of plastic waste collected was 101 kg (25.9 kg, 47.7 kg, and 27.4 kg, respectively, for each sampling), representing 28.2% of the total waste collected (Table 2).
Type of plastic | Total amount of plastic generated during sampling (kg) | Composition (% wt) * | Composition (% wt) ** |
PET (1) | 27.0 | 7.5 | 26.7 |
HDPE (2) | 4.7 | 1.3 | 4.7 |
PVC (3) | 0.6 | 0.2 | 0.6 |
LDPE (4) | 0.4 | 0.1 | 0.4 |
Polypropylene (PP) (5) | 2.4 | 0.7 | 2.4 |
Polystyrene (PS) (6) | 5.5 | 1.5 | 5.4 |
Polyurethane | 3.0 | 0.8 | 3.0 |
Other (7) | 2.0 | 0.6 | 2.0 |
Rigid plastic and film | 55.3 | 15.5 | 54.8 |
Total Plastics Collected | 101.0 | 28.2 | 100.0 |
*Based on a total weight of 358.0 kg of waste. **Based on a total weight of 101.0 kg of plastic waste. |
During the sample collection phase, various plastics were identified and classified within the mangrove ecosystem. The average weight of plastic waste was 33.7 kg/day (12,300 kg/year) and was distributed among the following categories:
● Polyethylene (PE), including PET, HDPE, and LDPE, constituted 31.8% (10.7 kg/day);
● Polypropylene (PP) accounted for 2.4% (0.8 kg/day);
● Polystyrene (PS) accounted for 5.4% (1.8 kg/day);
● Others (PVC, polyurethane, rigid plastics, and films) accounted for 60.4% (20.3 kg/day).
Using a conversion rate of 11%, the formation of microplastics was calculated. The amount generated by each type of plastic was estimated as follows:
● Polyethylene (PE): 1.18 kg/day;
● Polypropylene (PP): 0.09 kg/day;
● Polystyrene (PS): 0.20 kg/day;
● Others: 2.23 kg/day.
As a result, the total generation of microplastics was estimated to be 3.70 kg/day (1,351 kg/year).
These results indicate that the largest contribution to microplastic generation comes from PE, followed by PP. However, many rigid plastics and films, which do not have any symbol to identify them, were included in the classification of "others".
The environmental conditions specific to the mangrove ecosystem, including high humidity, temperature, and direct solar exposure, play a pivotal role in the degradation of plastics. For instance, the elevated humidity enhances microbial colonization, facilitating biodegradation, while high solar radiation accelerates photodegradation. Our findings align with the rates observed in tropical coastal ecosystems, where such factors collectively expedite the transformation of macroplastics into microplastics. These results underscore the necessity for localized studies to tailor waste management strategies to specific environmental conditions.
The quantity of microplastics produced is indicative of the composition of the plastic waste retrieved from the mangrove, highlighting the pressing issue of certain plastics, notably plastic films, and their substantial role in microplastic pollution within this ecosystem. When juxtaposed with findings from existing literature, a parallel trend emerges in research focused on coastal and marine ecosystems. In these studies, flexible and single-use plastics have been identified as significant contributors to microplastic pollution. This correlation is attributed to their prevalent presence in collected waste and their heightened vulnerability to physical degradation, underscoring the critical need for targeted measures to mitigate their impact.
The findings underscore the importance of implementing targeted strategies for plastic waste management in mangroves, with a focus on reducing the input of flexible and single-use plastics, which are significant generators of microplastics.
The environmental assessment of the mangrove forest identified the main problems and threats. Mangrove forests are recognized as ecosystems that provide essential environmental and ecosystem services in coastal or riparian zones where they grow [27,28]. As ecosystems dependent on tidal movements and located in river mouths and nearby areas, they become important areas for the deposition or trapping of solid waste, such as plastics, which generate significant impacts on the development and healthy growth of mangrove plants [27,28]
The major problems associated with this type of contamination are related to the accumulation of large amounts of solid plastic materials on soils, root systems, and branches, causing changes in the excessive growth of adventitious roots (pneumatophores) or damage to them [28]. Despite the abnormal and excessive growth of pneumatophores when covered by plastic debris, poor tree growth is also an observed effect [27].
The consequences of the loss of mangrove areas would include significant impacts on primary productivity, biological diversity, the capture of atmospheric CO2 (loss of carbon sinks), and a decrease in breeding areas for birds and many aquatic species such as fish, mollusks, and crustaceans. Additionally, the natural coastal environments that filter many environmental pollutants would be compromised [29,30].
Threats associated with the increasing discharge of solid waste in coastal-estuarine zones put mangrove communities at high risk, impacting not only tree species but also all the species related to these highly productive environments [30]. This includes meiofauna, sediment-dwelling species, and microbial communities responsible for important nutrient recycling and biogeochemical processes [29].
Engaging stakeholders, including local communities, scientists, NGOs, and policymakers, is essential for effective mangrove management. Collaborative workshops and consultations can facilitate inclusive strategies.
A clear and measurable objective is proposed for mangrove conservation and sustainable management, with its respective goal, indicator, strategy, and actions.
Objective: Reduction of Plastic Waste Pollution
● Goal: Achieve a 50% reduction in plastic waste found in the mangrove within two years.
● Indicators: The amount of plastic waste collected during regular mangrove clean-ups and the number of reported plastic pollution incidents.
Strategy: Integrated Waste Management Program
● Description: Implement a waste management program that includes installing selective collection points, recycling campaigns, and environmental education to reduce waste generation and promote proper disposal.
Actions:
● Place waste separation containers in key areas near the mangrove swamp.
● Organize regular community clean-up days in the mangrove swamp.
● Develop workshops and educational materials on the importance of reducing, reusing, and recycling.
Specific policies were developed for waste management:
1. Implementation of collection and recycling programs
o Establish waste collection points in strategic areas near the mangrove swamp to facilitate proper waste disposal.
o Promote recycling and reuse of materials, especially plastics, through collaboration with local recycling companies.
2. Environmental education and awareness
o Develop environmental education campaigns for the local community, tourists, and businesses, focusing on the importance of keeping the mangrove ecosystems clean.
o Organize workshops and mangrove clean-up activities to encourage community participation in waste management.
3. Littering regulations and fines
o Establish strict regulations to prohibit waste dumping in the mangrove and surrounding areas.
o Impose fines and penalties on individuals and companies that fail to comply with waste management regulations.
Periodic evaluations will be conducted to measure the effectiveness of implemented policies and actions, and adjustments will be made as necessary.
1) Develop environmental education programs to raise public awareness of the importance of mangroves and the need for their conservation.
2) Encourage community participation in mangrove conservation and clean-up activities.
1) Establish alliances with local, national, and international organizations to strengthen mangrove management.
2) Seek funding and technical support to implement mangrove conservation and restoration projects.
1) Be adaptive and flexible when adjusting policies and strategies in response to environmental changes or new scientific findings.
2) Promote innovation and the adoption of sustainable technologies in mangrove management.
Coastal areas are major tourist attractions and, consequently, are highly vulnerable to plastic pollution due to inadequate waste management practices. The botanical garden of UNACAR is a natural attraction in the region, housing several species of mangroves that create a habitat requiring conservation. The long lifespan of plastics and their exposure to environmental conditions such as solar radiation [6,7,9,10,11], high temperatures and humidity [14], erosion by water and wind, and their contact with organisms that may mistake them for shelter or food [12,15,16], influence the processes of fragmentation, chemical and biological degradation, weathering, and biological interactions. The plastics with the highest generation rate, polyethylene (PE) and polypropylene (PP), correspond to those identified as the most responsible for leakage and leakage intensities as reported by [24].
Building upon these findings, it is essential to explore how local environmental conditions, such as temperature, humidity, and solar radiation, accelerate the degradation and transformation of plastics into microplastics. Tropical climates, such as those in the study region, typically experience high temperatures ranging between 27 ℃ and 33 ℃, along with elevated humidity levels (72% annual average), creating an ideal environment for photodegradation and microbial activity. Solar radiation, particularly UV exposure, breaks down the chemical bonds in plastics, facilitating fragmentation and enhancing microplastic formation. For instance, researchers have found that higher UV radiation and prolonged sunlight exposure significantly increase degradation rates in polyethylene and polypropylene materials [1,9].
In addition to solar radiation and temperature, high humidity levels promote the colonization of plastic debris by microorganisms, which further contribute to their degradation through biological and chemical processes. The combination of these factors not only accelerates the transformation of macroplastics into microplastics but also amplifies their environmental impact, as smaller particles are more readily ingested by local fauna and integrated into the food web. This highlights the critical need for localized assessments to refine our understanding of how environmental variables affect the decomposition rates of different plastic types in mangrove ecosystems.
Moreover, the role of mangroves as unique environments for plastic degradation cannot be overlooked. Mangroves serve as a natural trap for debris, where plastic waste becomes lodged in root systems and exposed to tidal movements, which enhance mechanical abrasion and further contribute to fragmentation. These processes are compounded by biological interactions, as certain marine organisms ingest and excrete plastics in smaller forms, thus increasing microplastic prevalence [12,30]. Such findings underscore the necessity for targeted mitigation measures focused on reducing plastic inputs into these vulnerable ecosystems.
Our results, which estimate an 11% conversion rate of macroplastics to microplastics under local environmental conditions, align with data reported for similar tropical environments. However, the variability in transformation rates due to differing environmental conditions emphasizes the importance of justifying and refining assumptions based on localized data. The unique conditions of the mangrove ecosystem studied—such as high levels of organic matter, fluctuating salinity, and frequent waterlogging—may further influence the degradation processes, warranting additional research to better quantify their impact.
Addressing the issue of microplastic pollution requires a multifaceted approach. Implementing localized waste management strategies that account for the specific environmental conditions and degradation dynamics of mangroves is essential. Additionally, ongoing monitoring of microplastic generation and its environmental consequences will support more effective policy interventions and conservation efforts tailored to protect these critical ecosystems and their biodiversity. Future studies should also explore advanced methodologies to precisely measure the decomposition rates of plastics under various climatic scenarios to strengthen the scientific foundation for such interventions.
This research emphasizes need to address the accumulation of microplastics in mangrove ecosystems, particularly in southeastern Mexico. By providing a preliminary estimate of microplastic generation from urban waste, this study highlights the significant risks posed to the health and biodiversity of these critical environments. The method employed for estimating microplastic generation is straightforward and immediate, bypassing the need for complex laboratory analyses. However, the study acknowledges the value of precise analytical techniques in accurately identifying microplastic generation and distribution. Such methods are essential for developing more effective mitigation strategies and informing environmental management policies.
The findings advocate for a multi-faceted, collaborative effort involving scientists, policymakers, governmental agencies, and the broader community to combat this pressing environmental issue. Protecting mangrove ecosystems from both macro- and microplastic pollution is essential not only vital for preserving the health and functionality of these habitats but also for safeguarding the oceans and ensuring the sustainable use of natural resources for future generations.
The authors declare they have not used Artificial Intelligence (AI) tools in the creation of this article.
We express our gratitude to the UNACAR Authorities for permitting us to sample waste in the mangroves of the botanical garden.
We appreciate the support of the students of the Environmental Engineering degree at DACBiol-UJAT.
The authors declare that there is no conflict of interest in the development of the topic and the compilation of information.
José Ramón Laines-Canepa: Oversaw the sampling of waste in the botanical garden of UNACAR and writing—review and editing, Writing—original draft, Methodology, Conceptualization.
José Aurelio Sosa-Olivier: Review and editing.
Alejandro Padilla-Rivera: Estimation of microplastic generation and writing—review and editing.
José Roberto Hernández-Barajas: Writing—review and editing of manuscript.
Neil Ebeth Meled Morales-Rodríguez: Drone photography and figure design and editing.
Carlos Manuel Burelo-Ramos: Writing and review.
Alejandra Elvira Espinosa de los Monteros: Writing and editing document in English.
Gabriel Núñez-Nogueira: Writing—review and editing of manuscript.
[1] |
Cappa P, Walton MEM, Paler MKO, et al. (2023) Impact of mangrove forest structure and landscape on macroplastics capture. Mar Pollut Bull 194: 115434. https://doi.org/10.1016/j.marpolbul.2023.115434 doi: 10.1016/j.marpolbul.2023.115434
![]() |
[2] |
Al-Tarshi M, Dobretsov S, Gallardo W (2024) Marine litter and microplastic pollution in mangrove sediments in the Sea of Oman. Mar Pollut Bull 201: 116132. https://doi.org/10.1016/j.marpolbul.2024.116132 doi: 10.1016/j.marpolbul.2024.116132
![]() |
[3] |
Ordóñez OG, Arenas MRB (2019) Impacts of marine debris contamination in the mangrove ecosystem of the Ciénaga Grande de Santa Marta, Colombian Caribbean. Rev Cienc Mar Y Costeras 11: 134–154. https://doi.org/10.15359/revmar.11-2.8 doi: 10.15359/revmar.11-2.8
![]() |
[4] |
Kumar R, Verma A, Shome A, et al. (2021) Impacts of plastic pollution on ecosystem services, sustainable development goals, and need to focus on circular economy and policy interventions. Sustainability 13: 9963. https://doi.org/10.3390/su13179963 doi: 10.3390/su13179963
![]() |
[5] | Tekman MB, Walther B, Peter C, et al. (2022) Impacts of Plastic Pollution in the Oceans on Marine Species, Biodiversity and Ecosystems, Berlin: WWW Germany. https://doi.org/10.5281/zenodo.5898684 |
[6] |
George M, Nallet F, Fabre P (2024) A threshold model of plastic waste fragmentation: New insights into the distribution of microplastics in the ocean and its evolution over time. Mar Pollut Bull 199: 116012. https://doi.org/10.1016/j.marpolbul.2023.116012 doi: 10.1016/j.marpolbul.2023.116012
![]() |
[7] |
Zhang K, Hamidian AH, Tubić A, et al. (2021) Understanding plastic degradation and microplastic formation in the environment: A review. Environ Pollut 274: 116554. https://doi.org/10.1016/j.envpol.2021.116554 doi: 10.1016/j.envpol.2021.116554
![]() |
[8] |
Talukdar A, Kundu P, Bhattacharjee S, et al. (2023) Microplastics in mangroves with special reference to Asia: Occurrence, distribution, bioaccumulation and remediation options. Sci Total Environ 904: 166165. https://doi.org/10.1016/j.scitotenv.2023.166165 doi: 10.1016/j.scitotenv.2023.166165
![]() |
[9] |
Andrady AL (2022) Weathering and fragmentation of plastic debris in the ocean environment. Mar Pollut Bull 180: 113761. https://doi.org/10.1016/j.marpolbul.2022.113761 doi: 10.1016/j.marpolbul.2022.113761
![]() |
[10] |
Born MP, Brüll C (2022) From model to nature—A review on the transferability of marine (micro-) plastic fragmentation studies. Sci Total Environ 811: 151389. https://doi.org/10.1016/j.scitotenv.2021.151389 doi: 10.1016/j.scitotenv.2021.151389
![]() |
[11] |
Dimassi SN, Hahladakis JN, Yahia MND, et al. (2022) Degradation-fragmentation of marine plastic waste and their environmental implications: A critical review. Arab J Chem 15: 104262. https://doi.org/10.1016/j.arabjc.2022.104262 doi: 10.1016/j.arabjc.2022.104262
![]() |
[12] |
Lv S, Cui K, Zhao S, et al. (2024) Continuous generation and release of microplastics and nanoplastics from polystyrene by plastic-degrading marine bacteria. J Hazard Mater 465: 133339. https://doi.org/10.1016/j.jhazmat.2023.133339 doi: 10.1016/j.jhazmat.2023.133339
![]() |
[13] |
Reddy CN, Kallem P, Mounika K, et al. (2023) Review of microplastic degradation: Understanding metagenomic approaches for microplastic degrading organisms. Polym Test 128: 108223. https://doi.org/10.1016/j.polymertesting.2023.108223 doi: 10.1016/j.polymertesting.2023.108223
![]() |
[14] |
Shruti VC, Kutralam-Muniasamy G (2023) Migration testing of microplastics in plastic food-contact materials: Release, characterization, pollution level, and influencing factors. Trends Anal Chem 170: 117421. https://doi.org/10.1016/j.trac.2023.117421 doi: 10.1016/j.trac.2023.117421
![]() |
[15] |
Shin H, Jeong CB (2022) Metabolism deficiency and oxidative stress induced by plastic particles in the rotifer Brachionus plicatilis: Common and distinct phenotypic and transcriptomic responses to nano- and microplastics. Mar Pollut Bull 182: 113981. https://doi.org/10.1016/j.marpolbul.2022.113981 doi: 10.1016/j.marpolbul.2022.113981
![]() |
[16] |
Peng BY, Xu Y, Sun Y, et al. (2023) Biodegradation of polyethylene (PE) microplastics by mealworm larvae: Physiological responses, oxidative stress, and residual plastic particles. J Clean Prod 402: 136831. https://doi.org/10.1016/j.jclepro.2023.136831 doi: 10.1016/j.jclepro.2023.136831
![]() |
[17] | Instituto Nacional de Ecología (1997) Programa de Manejo de la Zona de Protección de Flora y Fauna Laguna de Términos. Available from: cpaot.org.mx/centro/ine-semarnat/anp/AN19.pdf. |
[18] | Geofolio 2024. Available from: https://geofolio.org/. |
[19] | Secretaria de Comercio y Fomento Industrial, NMX-AA-015-1985. Available from: biblioteca.semarnat.gob.mx/janium/Documentos/Ciga/agenda/DOFsr/NMX-AA-015-1985.pdf. |
[20] | Secretaria de Comercio y Fomento Industrial, NMX-AA-022-1985. Available from: biblioteca.semarnat.gob.mx/janium/Documentos/Ciga/agenda/DOFsr/NMX-AA-022-1985.pdf. |
[21] |
Munno K, De Frond H, O'Donnell B, et al. (2020) Increasing the accessibility for characterizing microplastics: Introducing new application-based and spectral libraries of plastic particles (SLoPP and SLoPP-E). Anal Chem 92: 2443–2451. https://doi.org/10.1021/acs.analchem.9b03626 doi: 10.1021/acs.analchem.9b03626
![]() |
[22] |
Hidalgo-Ruz V, Gutow L, Thompson RC, et al. (2012) Microplastics in the marine environment: A review of the methods used for identification and quantification. Environ Sci Technol 46: 3060–3075. https://doi.org/10.1021/es2031505 doi: 10.1021/es2031505
![]() |
[23] |
Ragusa A, Svelato A, Santacroce C, et al. (2021) Plasticenta: First evidence of microplastics in human placenta. Environ Int 146: 106274. https://doi.org/10.1016/j.envint.2020.106274 doi: 10.1016/j.envint.2020.106274
![]() |
[24] | Boucher J, Zgola M, Liao X, et al. (2020) National Guidance for Plastic Pollution Hotspotting and Shaping Action—Introduction to the Methodology, Nairobi: United Nations Environment Programme. Available from: wedocs.unep.org/bitstream/handle/20.500.11822/33166/NGP.pdf. |
[25] | Aguirre Santos JA, Álvarez Zeferino JC, Cruz Salas A (2023) Caracterización de residuos plásticos en la estación de transferencia de Iztapalapa, México, in X Simposio Iberoamericano de Ingeniería de Residuos At: Castelló de la Plana, España. https://doi.org/10.13140/RG.2.2.34687.79525 |
[26] |
Gani A, Irham M, Windari W (2022) An overview of the potential risks, sources, and analytical methods for microplastics in soil. AIMS Environ Sci 9: 185–216. https://doi.org/10.3934/environsci.2022013 doi: 10.3934/environsci.2022013
![]() |
[27] |
van Bijsterveldt CEJ, van Wesenbeeck BK, Ramadhani S, et al. (2021) Does plastic waste kill mangroves? A field experiment to assess the impact of macro plastics on mangrove growth, stress response and survival. Sci Total Environ 756: 143826. https://doi.org/10.1016/j.scitotenv.2020.143826 doi: 10.1016/j.scitotenv.2020.143826
![]() |
[28] |
De K, Sautya S, Dora GU, et al. (2023) Mangroves in the "Plasticene": High exposure of coastal mangroves to anthropogenic litter pollution along the Central-West coast of India. Sci Total Environ 858: 160071. https://doi.org/10.1016/j.scitotenv.2022.160071 doi: 10.1016/j.scitotenv.2022.160071
![]() |
[29] |
Carugati L, Gatto B, Rastelli E, et al. (2018) Impact of mangrove forests degradation on biodiversity and ecosystem functioning. Sci Rep 8: 13298. https://doi.org/10.1038/s41598-018-31683-0 doi: 10.1038/s41598-018-31683-0
![]() |
[30] |
Mendes DS, Beasley CR, Silva DNN, et al. (2023) Microplastic in mangroves: A worldwide review of contamination in biotic and abiotic matrices. Mar Pollut Bull 195: 115552. https://doi.org/10.1016/j.marpolbul.2023.115552 doi: 10.1016/j.marpolbul.2023.115552
![]() |
1. | Yoso Wiyarno, Sri Widyastuti, Muhammad Al Kholif, Wawan Gunawan, Innovative Multimedia Filtration for Effective Microplastic Removal in Mangrove Ecosystems: A Sustainable Approach to Environmental Health, 2025, 5, 2809-4255, 24, 10.53623/idwm.v5i1.599 |
Process | Description |
Fragmentation![]() |
Large plastic waste breaks down into fragments due to physical factors such as UV radiation, waves, wind, and mechanical abrasion. Prolonged exposure to sunlight and environmental weakens polymer bonds, forming microplastics [6,7,9,10,11]. |
Chemical Degradation![]() |
Plastics, composed of long polymer chains, degrade chemically under specific environmental conditions. Oxidation, hydrolysis and photodegradation alter the molecular structure of plastics, making them more susceptible to fragmentation [12]. |
Biological Degradation![]() |
Some microorganisms colonize the surface of plastics and partially degrade them. While biodegradation of conventional plastics is a slow, it contributes to reducing plastic size and facilitating the transformation into microplastics [12,13]. |
Weathering![]() |
Environmental factors such as temperature, humidity, and sunlight exposure cause weathering, resulting in physical and chemical changes that deteriorate plastics, making them more prone to fragmentation [14]. |
Biophysical Interactions![]() |
Biological activity, such as feeding by marine organisms, contributes to plastic fragmentation. Larger plastics may be ingested and excreted as smaller particles [12,15,16]. |
Type of plastic | Total amount of plastic generated during sampling (kg) | Composition (% wt) * | Composition (% wt) ** |
PET (1) | 27.0 | 7.5 | 26.7 |
HDPE (2) | 4.7 | 1.3 | 4.7 |
PVC (3) | 0.6 | 0.2 | 0.6 |
LDPE (4) | 0.4 | 0.1 | 0.4 |
Polypropylene (PP) (5) | 2.4 | 0.7 | 2.4 |
Polystyrene (PS) (6) | 5.5 | 1.5 | 5.4 |
Polyurethane | 3.0 | 0.8 | 3.0 |
Other (7) | 2.0 | 0.6 | 2.0 |
Rigid plastic and film | 55.3 | 15.5 | 54.8 |
Total Plastics Collected | 101.0 | 28.2 | 100.0 |
*Based on a total weight of 358.0 kg of waste. **Based on a total weight of 101.0 kg of plastic waste. |
Process | Description |
Fragmentation![]() |
Large plastic waste breaks down into fragments due to physical factors such as UV radiation, waves, wind, and mechanical abrasion. Prolonged exposure to sunlight and environmental weakens polymer bonds, forming microplastics [6,7,9,10,11]. |
Chemical Degradation![]() |
Plastics, composed of long polymer chains, degrade chemically under specific environmental conditions. Oxidation, hydrolysis and photodegradation alter the molecular structure of plastics, making them more susceptible to fragmentation [12]. |
Biological Degradation![]() |
Some microorganisms colonize the surface of plastics and partially degrade them. While biodegradation of conventional plastics is a slow, it contributes to reducing plastic size and facilitating the transformation into microplastics [12,13]. |
Weathering![]() |
Environmental factors such as temperature, humidity, and sunlight exposure cause weathering, resulting in physical and chemical changes that deteriorate plastics, making them more prone to fragmentation [14]. |
Biophysical Interactions![]() |
Biological activity, such as feeding by marine organisms, contributes to plastic fragmentation. Larger plastics may be ingested and excreted as smaller particles [12,15,16]. |
Type of plastic | Total amount of plastic generated during sampling (kg) | Composition (% wt) * | Composition (% wt) ** |
PET (1) | 27.0 | 7.5 | 26.7 |
HDPE (2) | 4.7 | 1.3 | 4.7 |
PVC (3) | 0.6 | 0.2 | 0.6 |
LDPE (4) | 0.4 | 0.1 | 0.4 |
Polypropylene (PP) (5) | 2.4 | 0.7 | 2.4 |
Polystyrene (PS) (6) | 5.5 | 1.5 | 5.4 |
Polyurethane | 3.0 | 0.8 | 3.0 |
Other (7) | 2.0 | 0.6 | 2.0 |
Rigid plastic and film | 55.3 | 15.5 | 54.8 |
Total Plastics Collected | 101.0 | 28.2 | 100.0 |
*Based on a total weight of 358.0 kg of waste. **Based on a total weight of 101.0 kg of plastic waste. |