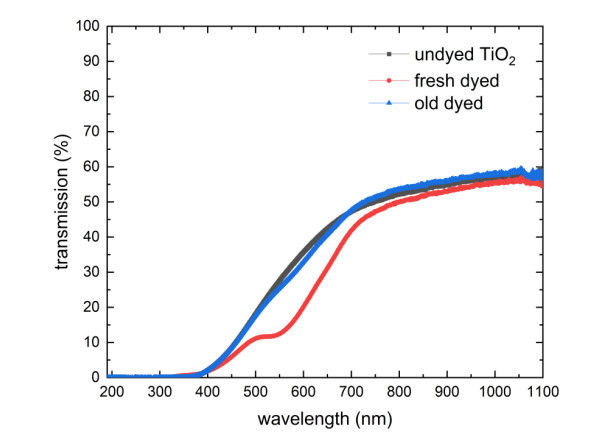
Long-term stability belongs to the main problems of dye-sensitized solar cells (DSSCs), impeding their practical application. Especially the usually fluid electrolyte tends to evaporation, thus drying the cells if they are not perfectly sealed. While gelling the electrolyte with different polymers often reduces the efficiency, using a glycerol-based electrolyte was already shown to result in similar or even improved efficiency. At the same time, drying of the cells was significantly reduced. Here we report on improving glycerol-based electrolytes further by varying the iodine-triiodide ratio and the overall concentration in the electrolyte. Long-term tests with unsealed glass-based DSSCs were performed over more than 1 year, showing that most of the cells increased efficiency during this time, opposite to cells with a commercial solvent-based iodine-triiodide electrolyte which completely dried after 2–3 months.
Citation: Katrin Gossen, Marius Dotter, Bennet Brockhagen, Jan Lukas Storck, Andrea Ehrmann. Long-term investigation of unsealed DSSCs with glycerol-based electrolytes of different compositions[J]. AIMS Materials Science, 2022, 9(2): 283-296. doi: 10.3934/matersci.2022017
[1] | Andrea Ehrmann, Tomasz Blachowicz . Recent coating materials for textile-based solar cells. AIMS Materials Science, 2019, 6(2): 234-251. doi: 10.3934/matersci.2019.2.234 |
[2] | Noah M. Johnson, Yuriy Y. Smolin, Chris Shindler, Daniel Hagaman, Masoud Soroush, Kenneth K. S. Lau, Hai-Feng Ji . Photochromic dye-sensitized solar cells. AIMS Materials Science, 2015, 2(4): 503-509. doi: 10.3934/matersci.2015.4.503 |
[3] | Roger Chang, Kemakorn Ithisuphalap, Ilona Kretzschmar . Impact of particle shape on electron transport and lifetime in zinc oxide nanorod-based dye-sensitized solar cells. AIMS Materials Science, 2016, 3(1): 51-65. doi: 10.3934/matersci.2016.1.51 |
[4] | Laila H. Abdel-Rahman, Ahmed M. Abu-Dief, Badriah Saad Al-Farhan, Doaa Yousef, Mohamed E. A. El-Sayed . Kinetic study of humic acid adsorption onto smectite: The role of individual and blend background electrolyte. AIMS Materials Science, 2019, 6(6): 1176-1190. doi: 10.3934/matersci.2019.6.1176 |
[5] | Bauyrzhan Rakhadilov, Ainur Zhassulan, Daryn Baizhan, Aibek Shynarbek, Kuanysh Ormanbekov, Tamara Aldabergenova . The effect of the electrolyte composition on the microstructure and properties of coatings formed on a titanium substrate by microarc oxidation. AIMS Materials Science, 2024, 11(3): 547-564. doi: 10.3934/matersci.2024027 |
[6] | Jie Sun, Tzvetanka Boiadjieva-Scherzer, Hermann Kronberger . Incorporation of zirconium into PEO coating on Ti6Al4V alloy from acidic electrolyte. AIMS Materials Science, 2021, 8(6): 974-989. doi: 10.3934/matersci.2021059 |
[7] | Carlos N. Kabengele, Giresse N. Kasiama, Etienne M. Ngoyi, Clement L. Inkoto, Juvenal M. Bete, Philippe B. Babady, Damien S. T. Tshibangu, Dorothée D. Tshilanda, Hercule M. Kalele, Pius T. Mpiana, Koto-Te-Nyiwa Ngbolua . Biogenic synthesis, characterization and effects of Mn-CuO composite nanocatalysts on Methylene blue photodegradation and Human erythrocytes. AIMS Materials Science, 2023, 10(2): 356-369. doi: 10.3934/matersci.2023019 |
[8] | Claas Hüter, Shuo Fu, Martin Finsterbusch, Egbert Figgemeier, Luke Wells, Robert Spatschek . Electrode–electrolyte interface stability in solid state electrolyte systems: influence of coating thickness under varying residual stresses. AIMS Materials Science, 2017, 4(4): 867-877. doi: 10.3934/matersci.2017.4.867 |
[9] | Bauyrzhan Rakhadilov, Lyaila Bayatanova, Sherzod Kurbanbekov, Ravil Sulyubayev, Nurdaulet Shektibayev, Nurbol Berdimuratov . Investigation on the effect of technological parameters of electrolyte-plasma cementation method on phase structure and mechanical properties of structural steel 20X. AIMS Materials Science, 2023, 10(5): 934-947. doi: 10.3934/matersci.2023050 |
[10] | Nirmalya Tripathy, Rafiq Ahmad, Jeong Eun Song, Hyun Park, Gilson Khang . ZnO nanonails for photocatalytic degradation of crystal violet dye under UV irradiation. AIMS Materials Science, 2017, 4(1): 267-276. doi: 10.3934/matersci.2017.1.267 |
Long-term stability belongs to the main problems of dye-sensitized solar cells (DSSCs), impeding their practical application. Especially the usually fluid electrolyte tends to evaporation, thus drying the cells if they are not perfectly sealed. While gelling the electrolyte with different polymers often reduces the efficiency, using a glycerol-based electrolyte was already shown to result in similar or even improved efficiency. At the same time, drying of the cells was significantly reduced. Here we report on improving glycerol-based electrolytes further by varying the iodine-triiodide ratio and the overall concentration in the electrolyte. Long-term tests with unsealed glass-based DSSCs were performed over more than 1 year, showing that most of the cells increased efficiency during this time, opposite to cells with a commercial solvent-based iodine-triiodide electrolyte which completely dried after 2–3 months.
Dye-sensitized solar cells (DSSCs) have been investigated since their discovery 30 years ago [1] since they can be built from low-cost, nontoxic materials without the necessity to work in a cleanroom. Even natural dyes, typically derived from plants, can be used to prepare DSSCs, with the disadvantage, however, of relatively low efficiencies, typically below 1% [2,3,4,5,6], with few examples of efficiencies in the range of 2–4% [7,8]. On the other hand, working with inexpensive materials allows for applying them on large areas, such as facades or textile architecture, e.g. tents.
It should be mentioned that using toxic ruthenium-based dyes, such as N719, or highly expensive dyes, such as N712, leads to significantly higher efficiencies. González-Verjan et al., e.g., report efficiencies of 4.0–6.4% using N719 combined with a nanoporous TiO2 layer and I2/LiI as electrolyte [9]. Subalakshmi and Senthilselvan also used N719 in their study of the effect of fluorine-doping of the TiO2 photoanode, reaching 4.6–7.4% efficiency [10]. Investigating combustion synthesized TiO2 as photoanode, Umale et al. also used N719 and reached an efficiency of 6.1% [11]. The effect of Pt-free metal sulfide counter electrodes was investigated by Subalakshmi et al. using N719, reporting an efficiency of 4.0% [12]. Patil et al. compared the 4 N, N-dimethylaniline-based polyene sensitizers NKX-2553, NKX-2554, NKX-2600, and NKX-2569 theoretically with a frontier molecular orbitals approach and report values of 5–7% efficiency with unclear DSSC setup [13]. N712 dye is only scarcely found in the literature due to its high cost and reduced efficiency in comparison with N719. It should be mentioned that even higher efficiencies between 12% and 14% under simulated sunlight and even 29% under ambient light were reported in the literature; however, usually for only one or a few cells and without time-dependent measurements [14,15,16,17].
Another important issue to make DSSCs technologically applicable is their long-term stability. Most studies investigate maximum efficiencies, gained directly after production or few days afterward, when the efficiency is usually highest. Long-term investigations, on the other hand, often concentrate on the degradation of the dye [18,19], while especially for the possible application in textile architecture, a fluid electrolyte causes more problems [20]. Evaporation or drying of the electrolyte is typically counteracted by using gel-electrolytes or solid-state electrolytes [21,22,23], often based on poly (ethylene oxide) (PEO) with ionic conductivity [24,25]. Gelling or polymerizing the electrolyte aims at encapsulating the electrolyte to reduce leakage and evaporation [23,26,27,28]. Some groups carried out accelerated aging experiments to improve gel or solid-state electrolytes further in terms of long-term stability [29,30,31,32,33]. These gel- or solid-state polymer electrolytes, however, often suffer from reduced efficiency, as compared to common fluid electrolytes [28,34,35,36].
One of the materials which are sometimes included in gel- or solid-state electrolytes is glycerol. Glycerol has a very low vapor pressure and can thus be expected to show nearly no evaporation for long time. Recently, we showed long-term stability of a new glycerol/poly (ethylene oxide) polymer electrolyte during 75 days, however, with a relatively low efficiency around 0.002% [35]. Other groups used glycerol as a plasticizer to improve the ionic conductivity or generally the efficiency as compared to pure polymer based electrolytes [37,38,39,40], often only showing single measurements of the DSSCs so that long-term evaluations can rarely be found in the literature. Even less literature is available about glycerol as an addition to fluid electrolytes [41].
This is why in a previous project we suggested a glycerol-based electrolyte. Indeed, the first results showed significantly reduced electrolyte evaporation and constant efficiencies for more than four months or in several cases even increasing values during the time of the study [42]. Here, continuing this study, we investigate the influence of the iodine:triiodide ratio as well as the concentration of the electrolyte in the glycerol, showing values of more than 1 year of long-term examination.
DSSCs were prepared using fluorine-doped tin oxide (FTO) coated glasses (Man Solar, Petten, The Netherlands) as front and back electrodes. All samples were prepared in triplicates. The front electrode had an additional TiO2 layer of approximate thickness 6 µm, built from nanoparticles with typical diameters around 100–200 nm [43]. A graphite layer was coated onto the counter electrodes by pencil (J. S. Staedtler), supporting reentry of the electrons into the cells, returning from an external load.
Dying of the TiO2 layer was performed with a forest fruit tea (Mayfair, Wilken Tee GmbH, Fulda, Germany) to enable comparison with the previous study. This tea contains anthocyanins which are used here as natural dyes. UV/Vis spectra of this tea can be found in Refs. [4,43,44]; transmission spectra of the pure TiO2 coated electrode, the TiO2 coated electrode with fresh and with old dye are depicted in Figure 1. The fresh dyed electrode shows a clear difference from the undyed TiO2 around 550 nm, while the older dyed electrode has nearly fully lost its color and shows a transmission spectrum quite similar to the undyed TiO2. The dying solution was prepared by mixing 2.5 g tea with 30 mL distilled water for 15 min and subsequent insertion of the TiO2 layers for 10 min. Finally, the excess dye was rinsed with distilled water, before the front electrodes were dried in air. It should be mentioned that such natural dyes typically have a factor ~70 lower efficiencies than DSSCs with N719 dye [44]; however, due to our aim to work with nontoxic dyes, N719 or similar dyes are not tested here.
After preparation of front and back electrodes, the glasses were mounted with the conductive sides pointing towards each other and fixed with transparent adhesive tape. This procedure led to an efficient cell area of 6 cm2, which is significantly larger than most DSSCs reported in the literature and is well-known to reduce the efficiency [45], but improves the reliability of the measurements and was chosen as study aims at producing large-scale long-term-stable solar cells.
The glycerol electrolytes were produced by adding iodine and potassium iodide to glycerol in different concentrations (15%, 20%, and 25%) and weight-ratios (1:1, 1:1.5, 1:2, 1:2.5, and 1:3). Concentrations are given as wt% of iodine with respect to the overall mass. All prepared DSSCs are shown in Table 1. A commercial water-based iodine–triiodide electrolyte (Man Solar) was used as reference. The filling of the cells with the various electrolytes was done using capillary forces. Since this study investigates evaporation of the electrolyte, also for the possible use in textile-based solar cells, the DSSCs were not sealed.
Concentration | Ratio (w/w) | Iodine mass (g) | Potassium iodide mass (g) | Glycerol mass (g) |
15% | 1:1 | 1.50 | 1.50 | 7.00 |
15% | 1:1.5 | 1.50 | 2.25 | 6.25 |
15% | 1:2 | 1.50 | 3.00 | 5.50 |
15% | 1:2.5 | 1.50 | 3.75 | 4.75 |
15% | 1:3 | 1.50 | 4.50 | 4.00 |
20% | 1:1 | 2.00 | 2.00 | 6.00 |
20% | 1:1.5 | 2.00 | 3.00 | 5.00 |
20% | 1:2 | 2.00 | 4.00 | 4.00 |
20% | 1:2.5 | 2.00 | 5.00 | 3.00 |
25% | 1:1 | 2.50 | 2.50 | 5.00 |
25% | 1:1.5 | 2.50 | 3.75 | 3.75 |
25% | 1:2 | 2.50 | 5.00 | 2.50 |
Electrical characterization was performed using a Keithley 2450 sourcemeter, illuminating the samples by a solar simulator LS0500 with AM 1.5 G spectrum (LOT-Quantum Design GmbH, Darmstadt/Germany), applying 100 mW/cm2. Between measurements, the DSSCs were stored in the dark at room temperature.
Figure 2 depicts exemplary current-voltage curves, taken on the first and on the last day of the measurements. For most curves, a change in the slope is visible, i.e. the fill factor reduces for 15%/1:2/no 3 (black curve) and 20%/1:2.5/no 1 (red curve), while the sample 25%/1:2/no 1 show a similar fill factor in both graphs. However, the curves of DSSCs with glycerol-based electrolytes have similar or even increased open-circuit voltages and short-circuit currents after more than 1 year or measurements, while the DSSC with the commercial electrolyte is clearly no longer working on the last day of the tests.
Figure 3 shows the results of the long-term test of the DSSCs with commercial iodine–triiodide electrolyte. The efficiencies of the three nominally identical cells rise slightly from the first day, reaching a maximum typically around 3–5 days, and afterwards decrease significantly. After 45–90 days, the samples have nearly reached zero efficiency, clearly showing that such fluid electrolytes cannot be used in unsealed DSSCs, as it would be necessary in textile architecture.
As usual for natural dyes, the energy conversion efficiencies are well below 1%, but approx. one order of magnitude higher than in cells with the previously mentioned glycerol/poly(ethylene oxide) polymer electrolytes [23]. Since this study deals with long-term stability of the electrolyte and not with improving the peak efficiency of DSSCs, the efficiency was not tried to be improved. Nevertheless, the results given here can easily be transferred to cells with toxic Ru-based dyes.
Next, the results of the long-time measurements of all samples with 15 wt% iodine are depicted in Figure 4. Here, the efficiencies during the first days are smaller than those of the commercial electrolyte, while nearly all samples maintain their original efficiency during one year of measurements. Taking into account the best specimens in each set of samples only, it can be stated that the iodine:potassium iodide mass ratio of 1:3 shows the highest efficiency. However, for most of the samples, significant deviations between the nominally identical specimens as well as between subsequent measurements are visible. These problems of imperfect reproducibility were discussed in a previous paper [23], where samples based on gel electrolytes showed even stronger irregularities.
On the one hand, manually preparing solar cells in the lab generally causes low reproducibility. Even with well-defined protocols regarding the application of the graphite layer, small deviations of the hand position or the pencil tip geometry will cause thicker or thinner layers and more or less FTO glass covered by graphite, leading to more or less optimized reentry efficiency of the electrons. An even more significant challenge is the thickness of the electrolyte layer which is mainly defined by the pressure between the glasses, posed by the transparent adhesive tape. Evidently, smallest deviations of the gap between both electrodes will result in significant modifications of the current in the solar cell. This explains the differences between nominally identical DSSCs during the first measurements and underlines the importance of measuring more than one specimen.
On the other hand, for later measurements, the sometimes visible severe differences between subsequent tests can be explained by the lab setup and measurement process, typically using crocodile clamps which after several measurements may destroy parts of the thin FTO layer on the glass, thus reducing the measured efficiency due to an increased contact resistance. This effect is, e.g., visible in Figure 4d in the blue line on the last-but-one day or in Figure 4e for the blue line on the last day. Such possible measurement errors also have to be taken into account during the evaluation of DSSC measurements in the lab where clamps are often used for measurements due to other problems with durable contacts.
A third point should also be mentioned. Comparing, e.g., the measurements at day 49 and the subsequent four test days, there is a certain minimum-maximum structure visible in nearly all curves. These deviations from day to day, reproduced in all cells, can typically be attributed to fluctuations in the environmental conditions, i.e. temperature and relative humidity in the lab, and indicate already the importance of taking into account such parameters, especially in case of not completely sealed DSSCs.
Next, Figure 5 shows the efficiencies of the samples prepared with 20 wt% iodine and different mass ratios of iodine:potassium iodide. While the ratio 1:1 (Figure 5a) shows firstly very low efficiencies, most specimens have more or less constant or even slightly increasing efficiencies with time. Here, the optimum ratio is 1:2.5 (Figure 5d), resulting in a highly stable efficiency over time and in the comparison of the three nominally identical specimens.
Finally, Figure 6 shows the results of the long-term tests of samples with 25 wt% iodine. All samples start at very low efficiencies, especially in case of a 1:1 ratio of iodine:potassium iodide (Figure 6a). These low values during the first weeks can be attributed to the high viscosity of these samples, leading to long durations until the pores of the semiconductor are filled. Differences between the three nominally identical samples per iodine:potassium iodide mass ratio are less pronounced than for 15% iodine or for the commercial electrolyte. On the other hand, the results are less stable than for the samples with 20 wt% iodine and a 1:2.5 mass ratio of iodine:potassium iodide.
Comparing all results, the sample with 20 wt% iodine and a 1:2.5 mass ratio of iodine:potassium iodide (Figure 5d) can be regarded as optimum, regarding reproducibility within the set of nominally identical samples and long-term stability during a year of measurements. The samples with 25 wt% iodine and the smallest amounts of potassium iodide (Figure 6a, b) show slightly higher efficiencies after one year, but significantly lower efficiencies during the first weeks, which would not make them technologically feasible.
As a next step, new specimens with 20 wt% iodine and a 1:2.5 mass ratio of iodine:potassium iodide have to be prepared to increase the efficiency by optimizing the residual parts of the solar cells, which have been used in very basic versions (e.g. without nanostructuring etc.) to enable easier comparison with results of other groups as well as to avoid undesired additional irreproducibility due to a more sophisticated design of materials and structures.
The efficiencies, open-circuit voltages and short-circuit currents are given for the first and the last day in Table 2. Here, similar trends are visible as in Figures 2 and 3—while the DSSC with commercial electrode is completely dried after more than one year, the cells with glycerol-based electrolytes are still working, in some cases better than on the first day.
Electrolyte | VOC/V (day 0) | VOC/V (day 372) | ISC/mA (day 0) | ISC/mA (day 372) | FF/% (day 0) | FF/% (day 372) | η/% (day 0) | η/% (day 372) |
ManSolar | 0.34 | 0.01 | 1.76 | 0 | 0.25 ± 0.07 | -/- | 0.025 ± 0.007 | 0.000 |
15%/1:1 | 0.16 | 0.26 | 0.58 | 1.13 | 0.34 ± 0.22 | 0.24 ± 0.05 | 0.005 ± 0.003 | 0.012 ± 0.002 |
15%/1:1.5 | 0.23 | 0.23 | 0.85 | 1.35 | 0.38 ± 0.08 | 0.21 ± 0.02 | 0.012 ± 0.003 | 0.011 ± 0.001 |
15%/1:2 | 0.21 | 0.24 | 1.12 | 1.17 | 0.29 ± 0.16 | 0.31 ± 0.11 | 0.011 ± 0.006 | 0.014 ± 0.005 |
15%/1:2.5 | 0.18 | 0.27 | 0.71 | 0.77 | 0.26 ± 0.22 | 0.41 ± 0.23 | 0.006 ± 0.004 | 0.014 ± 0.008 |
15%/1:3 | 0.21 | 0.36 | 0.94 | 1.33 | 0.43 ± 0.12 | 0.23 ± 0.02 | 0.014 ± 0.004 | 0.018 ± 0.002 |
20%/1:1 | 0.07 | 0.28 | 0.37 | 1.11 | 0.24 ± 0.01 | 0.26 ± 0.20 | 0.001 ± 0.000 | 0.013 ± 0.005 |
20%/1:1.5 | 0.18 | 0.30 | 1.06 | 1.51 | 0.32 ± 0.20 | 0.24 ± 0.03 | 0.010 ± 0.007 | 0.018 ± 0.002 |
20%/1:2 | 0.15 | 0.29 | 0.55 | 0.69 | 0.54 ± 0.47 | 0.28 ± 0.12 | 0.007 ± 0.006 | 0.009 ± 0.004 |
20%/1:2.5 | 0.22 | 0.38 | 1.02 | 0.85 | 0.37 ± 0.04 | 0.19 ± 0.16 | 0.014 ± 0.001 | 0.010 ± 0.008 |
25%/1:1 | 0.06 | 0.33 | 0.28 | 0.90 | 0.27 ± 0.05 | 0.33 ± 0.05 | 0.001 ± 0.000 | 0.017 ± 0.002 |
25%/1:1.5 | 0.16 | 0.34 | 0.69 | 0.72 | 0.30 ± 0.04 | 0.33 ± 0.06 | 0.005 ± 0.001 | 0.014 ± 0.002 |
25%/1:2 | 0.16 | 0.29 | 0.78 | 0.71 | 0.32 ± 0.20 | 0.30 ± 0.11 | 0.007 ± 0.004 | 0.010 ± 0.004 |
On day 0, the largest open-circuit voltages are found for the commercial ManSolar electrolyte, followed by 15%/1:1.5 and 20%/1:2.5. A clear trend, however, is not visible, which is typical for measurements during the first days when the electrolyte is not completely spread through the pores of the dyed semiconductor. On the last day of the measurements, the cells with commercial electrolyte have a voltage near zero, while all DSSCs with glycerol-based electrolytes have increased open-circuit voltages.
Comparing the short-circuit currents, the value for the cells with commercial electrolyte is again highest on day 0 and completely vanished on the last day of measurements. Most cells have increased their short-circuit currents during one year of measurements, only the cells with highly-viscous electrolytes 20%/1:2.5 and 25%/1:2 show reduced values.
Comparing the efficiencies shows again that in most cases the values for the DSSCs with glycerol-based electrolytes increase during the year of measurements, instead of reducing to zero, as the cells with the commercial electrolyte do. The fill factors decrease in most cases, but stay stable for some samples, especially those with electrolytes 25%.
These results are, to the best of our knowledge, the first values reported for a long-term study of one year. Typical long-term studies of DSSCs reported in the literature report about durations of 25–150 days [46,47,48,49,50,51,52], while a building-integrated (i.e. sealed) DSSC was only investigated in terms of color comfort after two years [53].
To conclude, the recent long-term study measured glycerol-based iodine-triiodide electrolytes in unsealed DSSCs with FTO glass electrodes. All DSSCs with glycerol-based electrolyte showed stable or increasing efficiencies over more than one year. By modifying the iodine-triiodide ratio, specimens with 20 wt% iodine and a 1:2.5 mass ratio of iodine:potassium iodide were found to have the highest efficiency along the whole measuring duration, with larger amounts of iodine being potentially interesting if a method can be developed to reach the maximum efficiencies earlier.
Next tests will concentrate on developing DSSCs with this electrolyte further to improve the overall efficiency while maintaining the long-term stability enabled by this novel electrolyte.
This research partly funded by the German Federal Ministry for Economic Affairs and Energy via the AiF, based on a resolution of the German Bundestag, grant number KK5044902SY0. The APC is funded by the Deutsche Forschungsgemeinschaft (DFG, German Research Foundation) (490988677) and Bielefeld University of Applied Sciences.
The authors declare no conflict of interest.
[1] |
O'Reagan B, Grätzel M (1991) A low cost, high efficiency solar cell based on dye sensitized colloidal TiO2 films. Nature 353: 737-740. https://doi.org/10.1038/353737a0 doi: 10.1038/353737a0
![]() |
[2] |
Gong J, Sumathy K, Qiao Q, et al. (2017) Review on dye-sensitized solar cells (DSSCs): Advanced techniques and research trends. Renewable Sustainable Energy Rev 68: 234-246. https://doi.org/10.1016/j.rser.2016.09.097 doi: 10.1016/j.rser.2016.09.097
![]() |
[3] |
Ehrmann A, Blachowicz T (2019) Recent coating materials for textile-based solar cells. AIMS Mater Sci 6: 234-251. https://doi.org/10.3934/matersci.2019.2.234 doi: 10.3934/matersci.2019.2.234
![]() |
[4] | Ehrmann A, Blachowicz T (2019) Comment on 'Dye-sensitized solar cells using Aloe vera and cladode of cactus extracts as natural sensitizers' [Chem. Phys. Lett. 679 (2017) 97-101]. Chem Phys Lett 714: 227-229. https://doi.org/10.1016/j.cplett.2018.10.009 |
[5] |
Burgos MJC, Roa S, Cerda B, et al. (2021) Effects of PbS-NPs doping on the photovoltaic performance of natural dye-sensitized TiO2 photoelectrodes. Solid State Commun 340: 114523. https://doi.org/10.1016/j.ssc.2021.114523 doi: 10.1016/j.ssc.2021.114523
![]() |
[6] |
Erande KB, Hawaldar PY, Suryawanshi SR, et al. (2021) Extraction of natural dye (specifically anthocyanin) from pomegranate fruit source and their subsequent use in DSSC. Mater Today Proc 43: 2716-2720. https://doi.org/10.1016/j.matpr.2020.06.357 doi: 10.1016/j.matpr.2020.06.357
![]() |
[7] |
Siregar N, Panggabean JH, Sirait M, et al. (2021) Fabrication of dye-sensitized solar cells (DSSC) using Mg-doped ZnO as photoanode and extract of rose myrtle (Rhodomyrtus tomentosa) as natural dye. Int J Photoenergy 2021: 4033692. https://doi.org/10.1155/2021/4033692 doi: 10.1155/2021/4033692
![]() |
[8] |
Rani AJML, Shanmugasundaram K, Sundaramurthy D, et al. (2021) Correlation study on biopolymer-blended cobalt and iodine gel electrolyte to enhance the efficiency of natural dye-based DSSCs. Energy Fuels 35: 15033-15044. https://doi.org/10.1021/acs.energyfuels.1c02264 doi: 10.1021/acs.energyfuels.1c02264
![]() |
[9] |
González-Verjan VA, Trujillo-Navarrete B, Félix-Navarro RM, et al. (2020) Effect of TiO2 particle and pore size on DSSC efficiency. Mater Renew Sustain Energy 9: 13. https://doi.org/10.1007/s40243-020-00173-7 doi: 10.1007/s40243-020-00173-7
![]() |
[10] |
Subalakshmi K, Senthilselvan J (2018) Effect of fluorine-doped TiO2 photoanode on electron transport, recombination dynamics and improved DSSC efficiency. Sol Energy 171: 914-928. https://doi.org/10.1016/j.solener.2018.06.077 doi: 10.1016/j.solener.2018.06.077
![]() |
[11] |
Umale S, Sudhakar V, Sontakke SM, et al. (2019) Improved efficiency of DSSC using combustion synthesized TiO2. Mater Res Bull 109: 222-226. https://doi.org/10.1016/j.materresbull.2018.09.044 doi: 10.1016/j.materresbull.2018.09.044
![]() |
[12] |
Subalakshmi K, Kumar KA, Paul OP, et al. (2019) Platinum-free metal sulfide counter electrodes for DSSC applications: Structural, electrochemical and power conversion efficiency analyses. Sol Energy 193: 507-518. https://doi.org/10.1016/j.solener.2019.09.075 doi: 10.1016/j.solener.2019.09.075
![]() |
[13] |
Patil DS, Avhad KC, Sekar N (2018) Linear correlation between DSSC efficiency, intramolecular charge transfer characteristics, and NLO properties—DFT approach. Comput Theor Chem 1138: 75-83. https://doi.org/10.1016/j.comptc.2018.06.006 doi: 10.1016/j.comptc.2018.06.006
![]() |
[14] |
Yella A, Lee HW, Tsao HN, et al. (2011) Porphyrin-sensitized solar cells with cobalt (II/III)-based redox electrolyte exceed 12 percent efficiency. Science 334: 629-634. https://doi.org/10.1126/science.1209688 doi: 10.1126/science.1209688
![]() |
[15] |
Mathew S, Yella A, Gao P, et al. (2014) Dye-sensitized solar cells with 13% efficiency achieved through the molecular engineering of porphyrin sensitizers. Nat Chem 6: 242-247. https://doi.org/10.1038/nchem.1861 doi: 10.1038/nchem.1861
![]() |
[16] |
Kakiage K, Aoyama Y, Yano T, et al. (2015) Highly-efficient dye-sensitized solar cells with collaborative sensitization by silyl-anchor and carboxy-anchor dyes. Chem Commun 51: 15894-15897. https://doi.org/10.1039/C5CC06759F doi: 10.1039/C5CC06759F
![]() |
[17] |
Freitag M, Teuscher J, Saygili Y, et al. (2017) Dye-sensitized solar cells for efficient power generation under ambient lighting. Nat Photonics 11: 372-378. https://doi.org/10.1038/nphoton.2017.60 doi: 10.1038/nphoton.2017.60
![]() |
[18] |
Hara K, Wang ZS, Cui Y, et al. (2009) Long-term stability of organic-dye-sensitized solar cells based on an alkyl-functionalized carbazole dye. Energy Environ Sci 2: 1109-1114. https://doi.org/10.1039/b907486d doi: 10.1039/b907486d
![]() |
[19] | Kabir F, Sakib SN, Matin N (2019) Stability study of natural green dye based DSSC. Optik 181 458-464. https://doi.org/10.1016/j.ijleo.2018.12.077 |
[20] |
Kohn S, Wehlage D, Junger IJ, et al. (2019) Electrospinning a dye-sensitized solar cell. Catalysts 9: 975. https://doi.org/10.3390/catal9120975 doi: 10.3390/catal9120975
![]() |
[21] | Chen KF, Liu CH, Huang HK, et al. (2013) Polyvinyl butyral-based thin film polymeric electrolyte for dye-sensitized solar cell with long-term stability. Int J Electrochem Sci 8: 3524-3539. |
[22] |
Yoon JS, Kang Dk, Won JG, et al. (2012) Dye-sensitized solar cells using ion-gel electrolytes for long-term stability. J Power Sources 201: 395-401. https://doi.org/10.1016/j.jpowsour.2011.11.012 doi: 10.1016/j.jpowsour.2011.11.012
![]() |
[23] |
Iftikhar H, Sonai GG, Hashmi SG, et al. (2019) Progress on electrolytes development in dye-sensitized solar cells. Materials 12: 1998. https://doi.org/10.3390/ma12121998 doi: 10.3390/ma12121998
![]() |
[24] |
Dissanayake MAKL, Ekanayake EMBS, Bandara, LRAK, et al. (2016) Efficiency enhancement by mixed cation effect in polyethylene oxide (PEO)-based dye-sensitized solar cells. J Solid State Electrochem 20: 193-201. https://doi.org/10.1007/s10008-015-3018-1 doi: 10.1007/s10008-015-3018-1
![]() |
[25] |
Lei B, Li GR, Chen P, et al. (2019) A quasi-solid-state solar rechargeable battery with polyethylene oxide gel electrolyte. ACS Appl Energy Mater 2: 1000-1005. https://doi.org/10.1021/acsaem.8b02193 doi: 10.1021/acsaem.8b02193
![]() |
[26] |
Wang Y (2009) Recent research progress on polymer electrolytes for dye-sensitized solar cells. Sol Energy Mater Sol Cells 93: 1167-1175. https://doi.org/10.1016/j.solmat.2009.01.009 doi: 10.1016/j.solmat.2009.01.009
![]() |
[27] |
Shi Y, Zhan C, Wang L, et al. (2009) The electrically conductive function of high-molecular weight poly(ethylene oxide) in polymer gel electrolytes used for dye-sensitized solar cells. Phys Chem Chem Phys 11: 4230-4235. https://doi.org/10.1039/b901003c doi: 10.1039/b901003c
![]() |
[28] |
de Freitas JN, Nogueira AF, de Paoli MA (2009) New insights into dye-sensitized solar cells with polymer electrolytes. J Mater Chem 19: 5279. https://doi.org/10.1039/b900928k doi: 10.1039/b900928k
![]() |
[29] |
Tao L, Huo ZP, Ding Y, et al. (2015) High-efficiency and stable quasi-solid-state dye-sensitized solar cell based on low molecular mass organogelator electrolyte. J Mater Chem A 3: 2344-2352. https://doi.org/10.1039/C4TA06188H doi: 10.1039/C4TA06188H
![]() |
[30] |
Bella F, Popovic J, Lamberti A, et al. (2017) Interfacial effects in solid-liquid electrolytes for improved stability and performance of dye-sensitized solar cells. ACS Appl Mater Interfaces 9: 43. https://doi.org/10.1021/acsami.7b11899 doi: 10.1021/acsami.7b11899
![]() |
[31] |
Huo Z, Wang L, Tao L, et al. (2017) A supramolecular gel electrolyte formed from amide based co-gelator for quasi-solid-state dye-sensitized solar cell with boosted electron kinetic processes. J Power Sources 359: 80-87. https://doi.org/10.1016/j.jpowsour.2017.04.099 doi: 10.1016/j.jpowsour.2017.04.099
![]() |
[32] |
Bastianini M, Vivani R, Nocchetti M, et al. (2014) Effect of iodine intercalation in nanosized layered double hydroxides for the preparation of quasi-solid electrolyte in DSSC devices. Sol Energy 107: 692-699. https://doi.org/10.1016/j.solener.2014.06.014 doi: 10.1016/j.solener.2014.06.014
![]() |
[33] |
Bella F, Vlachopoulos N, Nonomura K, et al. (2015) Direct light-induced polymerization of cobalt-based redox shuttles: an ultrafast way towards stable dye-sensitized solar cells. Chem Commun 51: 16308-16311. https://doi.org/10.1039/C5CC05533D doi: 10.1039/C5CC05533D
![]() |
[34] |
Song D, Cho W, Lee JH, et al. (2014) Toward higher energy conversion efficiency for solid polymer electrolyte dye-sensitized solar cells: Ionic conductivity and TiO2 pore-filling. J Phys Chem Lett 5: 1249-1258. https://doi.org/10.1021/jz5002727 doi: 10.1021/jz5002727
![]() |
[35] |
Storck JL, Dotter M, Brockhagen B, et al. (2020) Evaluation of novel glycerol/PEO gel polymer electrolytes for non-toxic dye-sensitized solar cells with natural dyes regarding long-term stability and reproducibility. Crystals 10: 1158. https://doi.org/10.3390/cryst10121158 doi: 10.3390/cryst10121158
![]() |
[36] |
Storck JL, Dotter M, Adabra S, et al. (2020) Long-term stability improvement of non-toxic dye-sensitized solar cells via poly (ethylene oxide). Polymers 12: 3035. https://doi.org/10.3390/polym12123035 doi: 10.3390/polym12123035
![]() |
[37] |
Kobayashi K, Pagot G, Vezzù K, et al. (2021) Effect of plasticizer on the ion-conductive and dielectric behavior of poly(ethylene carbonate)-based Li electrolytes. Polym J 53: 149-155. https://doi.org/10.1038/s41428-020-00397-4 doi: 10.1038/s41428-020-00397-4
![]() |
[38] |
Mustafa MO, Ghareeb HB, Aziz S, et al. (2020) Electrochemical characteristics of glycerolized PEO-based polymer electrolytes. Membranes 10: 116. https://doi.org/10.3390/membranes10060116 doi: 10.3390/membranes10060116
![]() |
[39] |
Nagaraj P, Sasidharan A, David V, et al. (2017) Effect of cross-linking on the performances of starch-based biopolymer as gel electrolyte for dye-sensitized solar cell applications. Polymers 9: 667. https://doi.org/10.3390/polym9120667 doi: 10.3390/polym9120667
![]() |
[40] |
Li Q, Chen X, Tang Q, et al. (2013) Imbibition of polypyrrole into three-dimensional poly (hydroxyethyl methacrylate/glycerol) gel electrolyte for robust quasi-solid-state dye-sensitized solar cells. J Mater Chem A 1: 8055-8060. https://doi.org/10.1039/c3ta11166k doi: 10.1039/c3ta11166k
![]() |
[41] | Kumar M, Kumar A, Kumar S, et al. (2021) Fabrication and characterization of TiO2 based dye-sensitized solar cell. AIP Conf Proc 2352: 010043. |
[42] |
Gossen K, Ehrmann A (2020) Glycerin-based electrolyte for reduced drying of dye-sensitized solar cells. Optik 207: 163772. https://doi.org/10.1016/j.ijleo.2019.163772 doi: 10.1016/j.ijleo.2019.163772
![]() |
[43] |
Junger IJ, Homburg SV, Grethe T, et al. (2017) Examination of the sintering process-dependent properties of TiO2 on glass and textile substrates. J Photonics Energy 7: 015001. https://doi.org/10.1117/1.JPE.7.015001 doi: 10.1117/1.JPE.7.015001
![]() |
[44] |
Hö lscher F, Trümper PR, Junger IJ, et al. (2018) Raising reproducibility in dye-sensitized solar cells under laboratory conditions. J Renew Sustain Energy 10: 013506. https://doi.org/10.1063/1.5013181 doi: 10.1063/1.5013181
![]() |
[45] |
Udomrungkhajornchai S, Junger IJ, Ehrmann A (2020) Optimization of the TiO2 layer in DSSCs by a nonionic surfactant. Optik 203: 163945. https://doi.org/10.1016/j.ijleo.2019.163945 doi: 10.1016/j.ijleo.2019.163945
![]() |
[46] |
Hara K, Wang, ZS, Cui Y, et al. (2009) Long-term stability of organic-dye-sensitized solar cells based on an alkyl-functionalized carbazole dye. Energy Environ Sci 2: 1109-1114. https://doi.org/10.1039/b907486d doi: 10.1039/b907486d
![]() |
[47] |
Jung HS, Koo BK, Kim JY, et al. (2014) Enhanced photovoltaic properties and long-term stability in plasmonic dye-sensitized solar cells via noncorrosive redox mediator. ACS Appl Mater Interfaces 6: 21. https://doi.org/10.1021/am5051982 doi: 10.1021/am5051982
![]() |
[48] |
Sonai GG, Tiihonen A, Miettunen K, et al. (2017) Long-term stability of dye-sensitized solar cells assembled with cobalt polymer gel electrolyte. J Phys Chem C 121: 17577-17585. https://doi.org/10.1021/acs.jpcc.7b03865 doi: 10.1021/acs.jpcc.7b03865
![]() |
[49] |
Ma'alinia A, Moghaddam HA, Nouri E, et al. (2018) Long-term stability of dye-sensitized solar cells using a facile gel polymer electroly. New J Chem 42: 13256-13262. https://doi.org/10.1039/C8NJ02157K doi: 10.1039/C8NJ02157K
![]() |
[50] |
Griffini G, Bella F, Nisic F, et al. (2014) Multifunctional luminescent down-shifting fluoropolymer coatings: A straightforward strategy to improve the UV-light harvesting ability and long-term outdoor stability of organic dye-sensitized solar cells. Adv Energy Mater 5: 1401312. https://doi.org/10.1002/aenm.201401312 doi: 10.1002/aenm.201401312
![]() |
[51] |
Önen T, Karakus MÖ, Coskun R, et al. (2019) Reaching stability at DSSCs with new type gel electrolytes. J Photoch Photobio A 385: 112082. https://doi.org/10.1016/j.jphotochem.2019.112082 doi: 10.1016/j.jphotochem.2019.112082
![]() |
[52] |
Jang YJ, Thogiti S, Lee K, et al. (2019) Long-term stable solid-state dye-sensitized solar cells assembled with solid-state polymerized hole-transporting material. Crystals 9: 452. https://doi.org/10.3390/cryst9090452 doi: 10.3390/cryst9090452
![]() |
[53] |
Roy A, Ghosh A, Bhandari S, et al. (2019) Color comfort evaluation of dye-sensitized solar cell (DSSC) based building-integrated photovoltaic (BIPV) glazing after 2 years of ambient exposure. J Phys Chem C 123: 23834-23837. https://doi.org/10.1021/acs.jpcc.9b05591 doi: 10.1021/acs.jpcc.9b05591
![]() |
1. | Marius Dotter, Lion Lukas Placke, Jan Lukas Storck, Uwe Güth, Characterization of PAN-TiO2 Nanofiber Mats and their Application as Front Electrodes for Dye-sensitized Solar Cells, 2022, 65, 2350-3696, 298, 10.14502/tekstilec.65.2022081 | |
2. | Angellina Ebenezer Anitha, Marius Dotter, A Review on Liquid Electrolyte Stability Issues for Commercialization of Dye-Sensitized Solar Cells (DSSC), 2023, 16, 1996-1073, 5129, 10.3390/en16135129 |
Concentration | Ratio (w/w) | Iodine mass (g) | Potassium iodide mass (g) | Glycerol mass (g) |
15% | 1:1 | 1.50 | 1.50 | 7.00 |
15% | 1:1.5 | 1.50 | 2.25 | 6.25 |
15% | 1:2 | 1.50 | 3.00 | 5.50 |
15% | 1:2.5 | 1.50 | 3.75 | 4.75 |
15% | 1:3 | 1.50 | 4.50 | 4.00 |
20% | 1:1 | 2.00 | 2.00 | 6.00 |
20% | 1:1.5 | 2.00 | 3.00 | 5.00 |
20% | 1:2 | 2.00 | 4.00 | 4.00 |
20% | 1:2.5 | 2.00 | 5.00 | 3.00 |
25% | 1:1 | 2.50 | 2.50 | 5.00 |
25% | 1:1.5 | 2.50 | 3.75 | 3.75 |
25% | 1:2 | 2.50 | 5.00 | 2.50 |
Electrolyte | VOC/V (day 0) | VOC/V (day 372) | ISC/mA (day 0) | ISC/mA (day 372) | FF/% (day 0) | FF/% (day 372) | η/% (day 0) | η/% (day 372) |
ManSolar | 0.34 | 0.01 | 1.76 | 0 | 0.25 ± 0.07 | -/- | 0.025 ± 0.007 | 0.000 |
15%/1:1 | 0.16 | 0.26 | 0.58 | 1.13 | 0.34 ± 0.22 | 0.24 ± 0.05 | 0.005 ± 0.003 | 0.012 ± 0.002 |
15%/1:1.5 | 0.23 | 0.23 | 0.85 | 1.35 | 0.38 ± 0.08 | 0.21 ± 0.02 | 0.012 ± 0.003 | 0.011 ± 0.001 |
15%/1:2 | 0.21 | 0.24 | 1.12 | 1.17 | 0.29 ± 0.16 | 0.31 ± 0.11 | 0.011 ± 0.006 | 0.014 ± 0.005 |
15%/1:2.5 | 0.18 | 0.27 | 0.71 | 0.77 | 0.26 ± 0.22 | 0.41 ± 0.23 | 0.006 ± 0.004 | 0.014 ± 0.008 |
15%/1:3 | 0.21 | 0.36 | 0.94 | 1.33 | 0.43 ± 0.12 | 0.23 ± 0.02 | 0.014 ± 0.004 | 0.018 ± 0.002 |
20%/1:1 | 0.07 | 0.28 | 0.37 | 1.11 | 0.24 ± 0.01 | 0.26 ± 0.20 | 0.001 ± 0.000 | 0.013 ± 0.005 |
20%/1:1.5 | 0.18 | 0.30 | 1.06 | 1.51 | 0.32 ± 0.20 | 0.24 ± 0.03 | 0.010 ± 0.007 | 0.018 ± 0.002 |
20%/1:2 | 0.15 | 0.29 | 0.55 | 0.69 | 0.54 ± 0.47 | 0.28 ± 0.12 | 0.007 ± 0.006 | 0.009 ± 0.004 |
20%/1:2.5 | 0.22 | 0.38 | 1.02 | 0.85 | 0.37 ± 0.04 | 0.19 ± 0.16 | 0.014 ± 0.001 | 0.010 ± 0.008 |
25%/1:1 | 0.06 | 0.33 | 0.28 | 0.90 | 0.27 ± 0.05 | 0.33 ± 0.05 | 0.001 ± 0.000 | 0.017 ± 0.002 |
25%/1:1.5 | 0.16 | 0.34 | 0.69 | 0.72 | 0.30 ± 0.04 | 0.33 ± 0.06 | 0.005 ± 0.001 | 0.014 ± 0.002 |
25%/1:2 | 0.16 | 0.29 | 0.78 | 0.71 | 0.32 ± 0.20 | 0.30 ± 0.11 | 0.007 ± 0.004 | 0.010 ± 0.004 |
Concentration | Ratio (w/w) | Iodine mass (g) | Potassium iodide mass (g) | Glycerol mass (g) |
15% | 1:1 | 1.50 | 1.50 | 7.00 |
15% | 1:1.5 | 1.50 | 2.25 | 6.25 |
15% | 1:2 | 1.50 | 3.00 | 5.50 |
15% | 1:2.5 | 1.50 | 3.75 | 4.75 |
15% | 1:3 | 1.50 | 4.50 | 4.00 |
20% | 1:1 | 2.00 | 2.00 | 6.00 |
20% | 1:1.5 | 2.00 | 3.00 | 5.00 |
20% | 1:2 | 2.00 | 4.00 | 4.00 |
20% | 1:2.5 | 2.00 | 5.00 | 3.00 |
25% | 1:1 | 2.50 | 2.50 | 5.00 |
25% | 1:1.5 | 2.50 | 3.75 | 3.75 |
25% | 1:2 | 2.50 | 5.00 | 2.50 |
Electrolyte | VOC/V (day 0) | VOC/V (day 372) | ISC/mA (day 0) | ISC/mA (day 372) | FF/% (day 0) | FF/% (day 372) | η/% (day 0) | η/% (day 372) |
ManSolar | 0.34 | 0.01 | 1.76 | 0 | 0.25 ± 0.07 | -/- | 0.025 ± 0.007 | 0.000 |
15%/1:1 | 0.16 | 0.26 | 0.58 | 1.13 | 0.34 ± 0.22 | 0.24 ± 0.05 | 0.005 ± 0.003 | 0.012 ± 0.002 |
15%/1:1.5 | 0.23 | 0.23 | 0.85 | 1.35 | 0.38 ± 0.08 | 0.21 ± 0.02 | 0.012 ± 0.003 | 0.011 ± 0.001 |
15%/1:2 | 0.21 | 0.24 | 1.12 | 1.17 | 0.29 ± 0.16 | 0.31 ± 0.11 | 0.011 ± 0.006 | 0.014 ± 0.005 |
15%/1:2.5 | 0.18 | 0.27 | 0.71 | 0.77 | 0.26 ± 0.22 | 0.41 ± 0.23 | 0.006 ± 0.004 | 0.014 ± 0.008 |
15%/1:3 | 0.21 | 0.36 | 0.94 | 1.33 | 0.43 ± 0.12 | 0.23 ± 0.02 | 0.014 ± 0.004 | 0.018 ± 0.002 |
20%/1:1 | 0.07 | 0.28 | 0.37 | 1.11 | 0.24 ± 0.01 | 0.26 ± 0.20 | 0.001 ± 0.000 | 0.013 ± 0.005 |
20%/1:1.5 | 0.18 | 0.30 | 1.06 | 1.51 | 0.32 ± 0.20 | 0.24 ± 0.03 | 0.010 ± 0.007 | 0.018 ± 0.002 |
20%/1:2 | 0.15 | 0.29 | 0.55 | 0.69 | 0.54 ± 0.47 | 0.28 ± 0.12 | 0.007 ± 0.006 | 0.009 ± 0.004 |
20%/1:2.5 | 0.22 | 0.38 | 1.02 | 0.85 | 0.37 ± 0.04 | 0.19 ± 0.16 | 0.014 ± 0.001 | 0.010 ± 0.008 |
25%/1:1 | 0.06 | 0.33 | 0.28 | 0.90 | 0.27 ± 0.05 | 0.33 ± 0.05 | 0.001 ± 0.000 | 0.017 ± 0.002 |
25%/1:1.5 | 0.16 | 0.34 | 0.69 | 0.72 | 0.30 ± 0.04 | 0.33 ± 0.06 | 0.005 ± 0.001 | 0.014 ± 0.002 |
25%/1:2 | 0.16 | 0.29 | 0.78 | 0.71 | 0.32 ± 0.20 | 0.30 ± 0.11 | 0.007 ± 0.004 | 0.010 ± 0.004 |