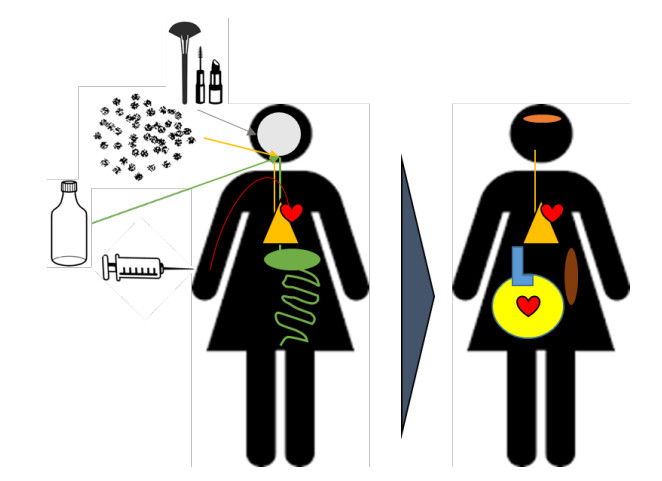
Citation: PA Stapleton. Toxicological considerations of nano-sized plastics[J]. AIMS Environmental Science, 2019, 6(5): 367-378. doi: 10.3934/environsci.2019.5.367
[1] | Gina M. Moreno, Keith R. Cooper . Morphometric effects of various weathered and virgin/pure microplastics on sac fry zebrafish (Danio rerio). AIMS Environmental Science, 2021, 8(3): 204-220. doi: 10.3934/environsci.2021014 |
[2] | Ebere Enyoh Christian, Qingyue Wang, Wirnkor Verla Andrew, Chowdhury Tanzin . Index models for ecological and health risks assessment of environmental micro-and nano-sized plastics. AIMS Environmental Science, 2022, 9(1): 51-65. doi: 10.3934/environsci.2022004 |
[3] | Andrew Wirnkor Verla, Christian Ebere Enyoh, Isiuku Beniah Obinna, Evelyn Ngozi Verla, Wang Qingyue, Md. Akhter Hossain Chowdhury, Emmanuel Chinedu Enyoh, Tanzin Chowdhury . Effect of macro-and micro-plastics in soil on growth of Juvenile Lime Tree (Citrus aurantium). AIMS Environmental Science, 2020, 7(6): 526-541. doi: 10.3934/environsci.2020033 |
[4] | B. Ravit, K. Cooper, B. Buckley, I. Yang, A. Deshpande . Organic compounds associated with microplastic pollutants in New Jersey, U.S.A. surface waters. AIMS Environmental Science, 2019, 6(6): 445-459. doi: 10.3934/environsci.2019.6.445 |
[5] | B. Ravit, K. Cooper, G. Moreno, B. Buckley, I. Yang, A. Deshpande, S. Meola, D. Jones, A. Hsieh . Microplastics in urban New Jersey freshwaters: distribution, chemical identification, and biological affects. AIMS Environmental Science, 2017, 4(6): 809-826. doi: 10.3934/environsci.2017.6.809 |
[6] | Katharina Meixner, Mona Kubiczek, Ines Fritz . Microplastic in soil–current status in Europe with special focus on method tests with Austrian samples. AIMS Environmental Science, 2020, 7(2): 174-191. doi: 10.3934/environsci.2020011 |
[7] | Patricia Morcillo, Maria Angeles Esteban, Alberto Cuesta . Mercury and its toxic effects on fish. AIMS Environmental Science, 2017, 4(3): 386-402. doi: 10.3934/environsci.2017.3.386 |
[8] | Abigail W. Porter, Sarah J. Wolfson, Lily. Young . Pharmaceutical transforming microbes from wastewater and natural environments can colonize microplastics. AIMS Environmental Science, 2020, 7(1): 99-116. doi: 10.3934/environsci.2020006 |
[9] | Vernieda B. Vergara, Christy A. Emond, John F. Kalinich . Tissue distribution patterns of solubilized metals from internalized tungsten alloy in the F344 rat. AIMS Environmental Science, 2016, 3(2): 290-304. doi: 10.3934/environsci.2016.2.290 |
[10] | Eliasu Issaka, Mabruk Adams, Enock Adjei Agyekum, Josephine Baffoe, Blessing Tornyeava . Per- and poly-fluoroalkyl substances: A review of sources, properties, chromatographic detection, and toxicological implications. AIMS Environmental Science, 2025, 12(2): 321-351. doi: 10.3934/environsci.2025015 |
Plastics are produced through the chemical and physical processing of naturally occurring constituents. Through polymerization and polycondensation, base constituents react together to form polymer chains, a process that can rarely be reversed. Therefore, once the reaction has occurred, these molecules cannot return to their previous basic form only be further processed or recycled to differing polymeric forms. Industrial chemicals may be added to the reaction to develop harder or more malleable results. Due to chemical stability, the environmental accumulation of plastics is on the rise and the research documenting these increases is receiving mainstream interest. Unfortunately, as identified in a recent editorial in Nature Nanotechnology, the laboratory and environmental toxicological assessments have not been completed, and overall, we simply do not know the outcomes [1].
The term “nanoplastic” is relatively novel. The first utilization of the term in a Web of Science search was within a 2004 abstract describing computational methods pertaining to material deformation [2]. As such, there has been some discussion in the literature regarding the definition of a ‘nanoplastic’. However, this is an important characterization for clarity as the field moves forward.
By definition, microplastics are plastic pieces that are less 5 millimeters (mm) in one dimension; therefore, nanoplastics would be considered ultrafine plastics that fall under this umbrella term. The discrepancy of terminology lies with how the nanoplastic produced. Nanoplastics in ecotoxicological settings are primarily formed by bulk degredation and have been defined as plastic materials less than 1000 nanometers (nm) [3]. There are secondarily derived through physical and mechanical breakdown, photodegradation, thermodegradation, and biodegradation of larger microplastics [4]. The size definition of nanomaterials is not isolated to plastics, but a symptom of a greater debate between scientists and regulators [5].
Nanomaterials traditionally describe particles that are intentionally produced at the nano-scale to take advantage of the physico-chemical properties available only at that size range [6]. Engineered or primary nanoplastics identified in personal care products, biomedical applications, and laboratory use are defined as less than 100 nanometers (nm) in a single dimension. For the purposes of this manuscript, we will define nanoplastics as particles that are less than 100 nm.
Unfortunately, due to their small size range, the quantity of nanoplastics in the environment currently cannot be measured. This is because the technologies to identify these small particles on a large scale have not yet been formulated. The traditional methodology of filtration cannot be used as the pores in most traditional containment centers are large enough to allow nanoplastics to pass through. Within the laboratory, nanotechnology techniques are in place to assess the small, known quantities to be characterized. These include dynamic light scattering, Raman spectroscopy, transmission electron microscopy, hyperspectral microscopy, and mass or size-based particle counters [7]. Further, laboratory assessments can modify nanoplastics to allow for their identification or quantification. This may be with the addition of a metallic core, or surface modifications including radioactive or fluorescent labelling [8,9,10]. Therefore, we await the analytic chemistry technologies. Further reading on the challenges of micro-, and subsequently nanoplastic, analyses are discussed here [11,12].
Nanoparticle potentials and toxicities are associated with the physico-chemical properties of the particles. This concept also holds true for nanoplastics. These particle characterizations include shape, size, chemical construct, and surface charge, each playing a key role in industrial and biocompatibility [13].
Nanoplastics may be a variety of shapes. These include intentionally produced spheres for personal care products, angular particles generated from bulk fragmentation, or long and thin synthetic fibers. As mentioned above, nanomaterials are defined as having one dimension that measures less than 100 nm; therefore, nanoplastics can range greatly in size [3]. The size of the particle directly relates to nanoplastic surface area-to-mass ratios. The surface area-to-mass corresponds to the amount of surface are of an object (particle) within a given volume or collection of particles. For example: 100, 10 nm sized particles can line up along the surface of a single 1 micron particle. Therefore, the large surface area-to-mass of the nanoparticles provide a greater surface for biological contact or chemical adsorption [1].
Plastic polymers are generally formed using industrial chemicals to promote specific material characteristics (e.g., color, flexibility, hardness). According to the Society of the Plastics Industry (SPI) and as it pertains to plastic waste management guideline, there are 7 different types of plastics classified by their recycling code: (1) Polyethylene Terephthalate [PET(E)], (2) High-Density Polyethylene (HDPE), (3) Polyvinyl Chloride (PVC), (4) Low-Density Polyethylene (LDPE), (5) Polypropylene (PP), (6) Polystyrene, and (7) Other as not identified above, including Polycarbonate and polylactide (nylon) [14]. Each of these is made with differing general properties and commonly used in household products. While other modifications are available on the market due to material advances since the SPI guidelines were established (e.g., acrylics, acrylonitrile butadiene styrene, and polybrominated compounds), the toxicological assessments at the nanoscale have not been assessed. Exposure may not be limited to the baseline product or chemical modifications during degradation, but also chemical leaching of the additives may provide additional sources of contamination or toxicity [15].
Not only do nanoplastics have a polarization associated with their chemicals construct that may influence the hydrophilicity and hydrophobicity of the particle; but they can also adsorb chemical contaminants to their surface, transporting them within the environment or through a biological system [16,17,18]. Of the particles analyzed thus far, polyethylene has the greatest chemical sorption rates [19]. These chemical additions may act as a secondary toxicant or as a functionalized group on the surface of the particle, encouraging or discouraging biological interaction. These differential surface modifications and particle transformations will impact nanoplastic fate and toxicity [9,16,18,20].
Given their size characteristics, nanoplastics easily escape traditional containment structures and solutions. Through disposal and degradation, nanoplastic particles can easily bypass landfill and wastewater containment, entering marine systems or becoming airborne; once in these forms, nanoplastics have the propensity for biological interactions associated with environmental and human exposure. As ongoing research continues to encourage the development of technologies and methodologies to aid in nanoplastic evaluation, it reveals the far-reaching scope of these particles.
Toxicology encompasses the biological relevance and adverse effects associated with exposure. As described above, nanoplastics have the propensity to be taken up by and enter animal and human systems. Studies are underway to establish the biological consequences associated with these exposures.
Due to their small size, nanoplastics are widely distributed in the aquatic environment and can be easily ingested and taken up by a wide range of aquatic biota. Ingestion of microplastics represents an environmental concern for the health of the individual as well as for the trophic transfer of plastic contaminants to larger predators as in the case of transfer from algae, to zooplankton, and fish [21,22]. Small nanoplastics were found to directly absorb through the intestinal wall of mussels [20] and bioaccumulate in barnacles [23]. Evidence of plastic particles in the terrestrial environment confirm nanoplastic uptake by plants, earthworms, and in air pollution or aerosolized particulate matter [24].
As it pertains to biological activity, the nanoplastic chemical construct and surface charge influences cellular uptake rates in mussels and sea urchins [25,26]. Further, exposures to nanopolystyrene particles impair insulin and lipid peroxidation signaling cascades [27,28]. Interestingly, nanoplastic toxicity is differential as it relates to the health and anaerobic digestion activity of microbial communities [29]. Genotoxicity and modified genetic expression patterns has been identified after exposure in brine shrimp and zooplankton, leading to the hypothesis that nanoplastics may be mutagenic in high doses [28,30]. Co-incubation of polystyrene and polycarbonate nanoparticles promoted upregulation of stress responses within the innate immune system of fish [31]. The majority of work done in the field has been conducted in environmental models and this body of work has recently been reviewed [32].
With respect to human health, nanoplastic exposure may be through gastric ingestion, pulmonary inhalation, dermal application, and intentional injection (Figure 1). Exposure to nanoplastics may also be described as: (1) intentional means, as with the use of personal care products or biomedical applications [33], (2) unintentional exposure through intentional plastic use, as with consumption of bottled water [34], or (3) unintentional exposure, as with nanoplastic inhalation as a part of air pollution or digestion through food production [13,35,36,37]. Given the proliferation of nanoplastics within the food and water sources, gastric exposure is likely. However, as it pertains to the human environment, higher concentrations of airborne microplastics and extrapolated nanoplastics have been measured indoors [36].
While it is easiest or most comfortable to look at downstream contamination, separate from our homes and daily use activities, synthetic clothing is a primary source of airborne micro- and subsequent nanoplastics in the indoor and outdoor environments [35]. With regard to the widespread use of synthetic clothing and the amount of time that people spend indoors in domestic and occupational settings, this type and route of exposure need to be taken into consideration in future studies.
Recently, the potential human consumption of microplastics was assessed via meta-analysis [38]. Through these analyses, the authors calculated adults would be exposed to an average of 258 to 312 microplastic particles daily. The authors further determined that exposure would differential between the adults/children, sexes, and oral consumption or inhalation exposure [38]. Given the size disparity between micro- and nanoplastic particles, the estimate of nanoparticle exposure would be exponential.
Current theories of human exposure and toxicity to nanoplastics identify ingestion as the primary exposure route [39]. While no direct toxicological assessments associated with the human ingestion of nanoplastics have been conducted, studies have identified that humans are consuming microplastics via their drinking water [34]. Further preliminary prospective analyses of human stool provide evidence of excretion of these particles, indicating exposure through food consumption [40]. When combined with studies of ingestion uptake in environmental models, it is evident that systemic up of nanoplastics in humans is likely. However, while concerns regarding human exposure via agroecosystems persist, analytical studies focused on known quantities of ingestion, paired with intestinal uptake, excretion, and particle fate have not been conducted [22].
Second to nanoplastic ingestion is inhalation as a plausible route of human exposure. This may occur through indoor activities as identified above, or through the drying of contaminated waterways or wastewater [39]. Inhalation of nanosized particles or ultrafine air pollution (PM0.1) is associated with many health effects [41,42]. Particles within this size range deposit deep within the lung and remain in the alveolar space or translocate to other regions of the body [43,44,45]. As it pertains to plastics, through case study analyses [46], airborne microplastics exposures are known to cause disease (i.e., inflammation and cancer) after occupational exposure [36]. Further, animal studies suggest an increase in pulmonary inflammation associated with occupational exposures [47].
Studies have been conducted using nanoplastic injection as an exposure route. These studies primarily evaluate material translocation and excretion. Interestingly, using ex vivo assessment, our laboratory has determined that fluorescently labeled 20 nm polystyrene nanoparticles particles can cross the placental barrier and enter the fetal compartment via the umbilical vein within 90 minutes of infusion into the maternal uterine artery [48].
Nanoplastics have been identified in personal care products, specifically facial scrubs [33], leading to the direct application of these materials onto the surface of the skin. While no studies to date have evaluated whether nanoplastics can cross the skin barrier, a single study evaluated engineered nanomaterials applied to textiles and identified that uptake of particles within this size range crossing intact skin is very low [49].
In vitro assessments investigate the local toxicities of particle-cellular interactions, making the assumption of systemic uptake, nanoplastic translocation, and deposition from the original site of exposure. The systemic outcomes associated with nanoparticle exposure are being elucidated; however, in each laboratory application of nanoplastic to the biological environment, toxicity has been identified.
Few cellular studies have been conducted to identify the cytotoxic effects of nanoplastic exposure and biological interactions. Co-incubation of nano-sized polystyrene and polyethylene particles have culminated in impaired the cellular metabolism of human lung cells [50] and increased oxidative stress [51] in epithelial and cerebral cell cultures. Further, the nanoplastic physoicochemical properties including size and surface modifications will directly affect cellular uptake and function in the forms of membrane disturbances, energy production, and oxidative stress [20,52].
While many models consider the direct exposure of nanoplastics, future considerations must be made as it pertains to secondary toxicity associated with particle translocation and deposition (Figure 1). Often, the organs and systems considered as it pertains to nanomaterial transport and systemic toxicity are the vasculature, lymphatics, and filter organs (e.g. liver, kidney, spleen) [43]. Unfortunately, the majority of this work has yet to be conducted.
However, maternal-fetal models of exposure provide crucial data regarding translocation, deposition, and physiological barrier function. Within these assessments, nanoplastics have been identified within the embryonic tissues of zebrafish [53]. Recent evidence from our laboratory identifies the translocation of nano-sized polystyrene particle from the maternal to the fetal compartment, across the placental barrier within 70 minutes of injection into the uterine artery [48]. This perturbation of the placental barrier was echoed in a size-dependent manner wherein, nanopolystyrene particles were taken up by placental cells and translocated between fetal and maternal compartments in human placenta [54,55].
Taking into account particle translocation within the maternal system, recent preliminary evidence from our laboratory indicates the propensity of nanoplastic to migrate out of the maternal lungs within 24-hours after pulmonary exposure, depositing within the liver, spleen, and kidney [8]. Further, within our maternal-fetal model, we were able to detect 20 nm fluorescently-labelled polystyrene particles within the fetus, depositing within the placenta, heart, liver, and brain [8]. However, the local effects within fetal tissues or the lifelong outcome of this nanoplastic deposition is currently unknown.
At the present time, it is established that nanoplastic particles can cross biological membranes and influence cellular signaling; however, the cellular and systemic toxicities associated with these exposures have yet to be revealed. Future studies also must identify environmentally-relevant concentrations and take into account the nanoplastic physicochemical properties of each analyzed.
Plastics and their constituents are produced at a faster rate than their toxicities can be evaluated. For example, Bisphenol A (BPA) found industrial use in the mid-1950’s in the production of polycarbonate plastic and after initial assessments, was deemed safe for food packaging [56]. Low dose exposure to BPA was later identified as an endocrine disrupting compound with possible carcinogenic properties and subsequently banned for food product use in Canada, the EU, and the US between 2008 and 2012 [57]. In its place, BPA analogs Bisphenol S and F (BPS and BPF, respectively) are incorporated in consumer products to provide the same merchandise quality [58,59]. Unfortunately, given the novelty of these compounds, full toxicological assessments have not been completed and early results are conflicting [60,61,62]. In this example, the fields of engineering and chemistry have acted at a faster rate than the toxicological assessments of the new compounds can be properly conducted.
Further, with respect to the management of discarded plastics, the use of reverse polymerization is well documented. Concerning to toxicologists is the occupational and environmental exposures associated with reforming the chemical identity of these manipulated compounds and the intermediary gaseous components released during the process. However, there are few management strategies currently available to control plastic waste.
Understanding material fate and the toxicological effects of nanoplastics requires a collaborative effort from a wide variety of professionals including environmentalists, waste management specialists, chemists, engineers, and toxicologists. Recently, Rutgers University hosted a conference focused on the Impacts of Microplastics in the Urban Environment. At this meeting, the organizers had the foresight to invite experts in each of these fields to present their current work and encourage an open dialogue. Continued communication and engagement between these groups will allow collaborative efforts to identify a better understanding of particle properties, waste management strategies, changes to the properties over the plastic lifecycle, and the biological relevance of these differing properties.
We thank Dr. Sara Fournier for her review of the manuscript. This work was supported by the National Institute of Environmental Health Sciences (R00-ES024783) and the Rutgers Center for Environmental Exposures and Disease (P30-ES005022).
The author declares no conflict of interest.
[1] | Nanoplastic should be better understood. (2019) Nat Nanotechnol 14: 299. |
[2] | Tsuru T, Shibutani Y (2006) Coupled simulation synchronized by molecular and dislocation dynamics. Comput Methods, Pts 1 and 2: 583-588. |
[3] |
Gigault J, Halle AT, Baudrimont M, et al. (2018) Current opinion: What is a nanoplastic? Environ Pollut 235: 1030-1034. doi: 10.1016/j.envpol.2018.01.024
![]() |
[4] |
Jahnke A, Arp HPH, Escher BI, et al. (2017) Reducing Uncertainty and Confronting Ignorance about the Possible Impacts of Weathering Plastic in the Marine Environment. Environ Sci Tech Lett 4: 85-90. doi: 10.1021/acs.estlett.7b00008
![]() |
[5] |
Miernicki M, Hofmann T, Eisenberger I, et al. (2019) Legal and practical challenges in classifying nanomaterials according to regulatory definitions. Nat Nanotechnol 14: 208-216. doi: 10.1038/s41565-019-0396-z
![]() |
[6] |
Oberdorster G, Oberdorster E, Oberdorster J (2005) Nanotoxicology: an emerging discipline evolving from studies of ultrafine particles. Environ Health Persp 113: 823-839. doi: 10.1289/ehp.7339
![]() |
[7] |
Wagner S, Reemtsma T (2019) Things we know and don't know about nanoplastic in the environment. Nat Nanotechnol 14: 300-301. doi: 10.1038/s41565-019-0424-z
![]() |
[8] | Stapleton PA. Nanoplastic translocation between the maternal-fetal environment 2019; Rutgers University, New Brunswick, NJ. |
[9] |
Sander M, Kohler HE, McNeill K (2019) Assessing the environmental transformation of nanoplastic through (13)C-labelled polymers. Nat Nanotechnol 14: 301-303. doi: 10.1038/s41565-019-0420-3
![]() |
[10] |
Mitrano DM, Beltzung A, Frehland S, et al. (2019) Synthesis of metal-doped nanoplastics and their utility to investigate fate and behaviour in complex environmental systems. Nat Nanotechnol 14: 362-368. doi: 10.1038/s41565-018-0360-3
![]() |
[11] |
Silva AB, Bastos AS, Justino CIL, et al. (2018) Microplastics in the environment: Challenges in analytical chemistry-A review. Anal Chim Acta 1017: 1-19. doi: 10.1016/j.aca.2018.02.043
![]() |
[12] |
Song YK, Hong SH, Jang M, et al. (2015) A comparison of microscopic and spectroscopic identification methods for analysis of microplastics in environmental samples. Mar Pollut Bull 93: 202-209. doi: 10.1016/j.marpolbul.2015.01.015
![]() |
[13] |
Bouwmeester H, Hollman PC, Peters RJ (2015) Potential Health Impact of Environmentally Released Micro-and Nanoplastics in the Human Food Production Chain: Experiences from Nanotoxicology. Environ Sci Technol 49: 8932-8947. doi: 10.1021/acs.est.5b01090
![]() |
[14] | Council RD Different Types of Plastic and Their Classification. https://www.ryedale.gov.uk/attachments/article/690/Different_plastic_polymer_types.pdf. |
[15] |
Oehlmann J, Schulte-Oehlmann U, Kloas W, et al. (2009) A critical analysis of the biological impacts of plasticizers on wildlife. Philos Trans R Soc Lond B Biol Sci 364: 2047-2062. doi: 10.1098/rstb.2008.0242
![]() |
[16] |
Song Z, Yang X, Chen F, et al. (2019) Fate and transport of nanoplastics in complex natural aquifer media: Effect of particle size and surface functionalization. Sci Total Environ 669: 120-128. doi: 10.1016/j.scitotenv.2019.03.102
![]() |
[17] |
Liu L, Fokkink R, Koelmans AA (2016) Sorption of polycyclic aromatic hydrocarbons to polystyrene nanoplastic. Environ Toxicol Chem 35: 1650-1655. doi: 10.1002/etc.3311
![]() |
[18] |
Tallec K, Blard O, Gonzalez-Fernandez C, et al. (2019) Surface functionalization determines behavior of nanoplastic solutions in model aquatic environments. Chemosphere 225: 639-646. doi: 10.1016/j.chemosphere.2019.03.077
![]() |
[19] |
Alimi OS, Farner Budarz J, Hernandez LM, et al. (2018) Microplastics and Nanoplastics in Aquatic Environments: Aggregation, Deposition, and Enhanced Contaminant Transport. Environ Sci Technol 52: 1704-1724. doi: 10.1021/acs.est.7b05559
![]() |
[20] |
Bhattacharjee S, Ershov D, Islam MA, et al. (2014) Role of membrane disturbance and oxidative stress in the mode of action underlying the toxicity of differently charged polystyrene nanoparticles. Rsc Adv 4: 19321-19330. doi: 10.1039/C3RA46869K
![]() |
[21] |
Cedervall T, Hansson LA, Lard M, et al. (2012) Food chain transport of nanoparticles affects behaviour and fat metabolism in fish. PLoS One 7: e32254. doi: 10.1371/journal.pone.0032254
![]() |
[22] |
Ng EL, Huerta Lwanga E, Eldridge SM, et al. (2018) An overview of microplastic and nanoplastic pollution in agroecosystems. Sci Total Environ 627: 1377-1388. doi: 10.1016/j.scitotenv.2018.01.341
![]() |
[23] | Bhargava S, Lee SSC, Ying LSM, et al. (2018) Fate of Nanoplastics in Marine Larvae: A Case Study Using Barnacles, Amphibalanus amphitrite. Acs Sustainable Chem& Engin 6: 6932-6940. |
[24] | Chang CW, Seibel AJ, Song JW (2019) Application of microscale culture technologies for studying lymphatic vessel biology. Microcirculat e12547. |
[25] | Della Torre C, Bergami E, Salvati A, et al. (2014) Accumulation and Embryotoxicity of Polystyrene Nanoparticles at Early Stage of Development of Sea Urchin Embryos Paracentrotus lividus. Environ Sci & Techno 48: 12302-12311. |
[26] |
Canesi L, Ciacci C, Fabbri R, et al. (2016) Interactions of cationic polystyrene nanoparticles with marine bivalve hemocytes in a physiological environment: Role of soluble hemolymph proteins. Environ Res 150: 73-81. doi: 10.1016/j.envres.2016.05.045
![]() |
[27] |
Shao H, Han Z, Krasteva N, et al. (2019) Identification of signaling cascade in the insulin signaling pathway in response to nanopolystyrene particles. Nanotoxicology 13: 174-188. doi: 10.1080/17435390.2018.1530395
![]() |
[28] |
Mishra P, Vinayagam S, Duraisamy K, et al. (2019) Distinctive impact of polystyrene nano-spherules as an emergent pollutant toward the environment. Environ Sci Pollut Res Int 26: 1537-1547. doi: 10.1007/s11356-018-3698-z
![]() |
[29] |
Fu SF, Ding JN, Zhang Y, et al. (2018) Exposure to polystyrene nanoplastic leads to inhibition of anaerobic digestion system. Sci Total Environ 625: 64-70. doi: 10.1016/j.scitotenv.2017.12.158
![]() |
[30] | Zhang W, Liu Z, Tang S, et al. (2019) Transcriptional response provides insights into the effect of chronic polystyrene nanoplastic exposure on Daphnia pulex. Chemosphere 238: 124563. |
[31] |
Greven AC, Merk T, Karagoz F, et al. (2016) Polycarbonate and polystyrene nanoplastic particles act as stressors to the innate immune system of fathead minnow (Pimephales promelas). Environ Toxicol Chem 35: 3093-3100. doi: 10.1002/etc.3501
![]() |
[32] |
Shen M, Zhang Y, Zhu Y, et al. (2019) Recent advances in toxicological research of nanoplastics in the environment: A review. Environ Pollut 252: 511-521. doi: 10.1016/j.envpol.2019.05.102
![]() |
[33] |
Hernandez LMY, N.; Tufenkji, N. (2017) Are there nanoplastics in you personal care products. Environ Sci Technol Lett 4: 280-285. doi: 10.1021/acs.estlett.7b00187
![]() |
[34] |
Mason SA, Welch VG, Neratko J (2018) Synthetic Polymer Contamination in Bottled Water. Front Chem 6: 407. doi: 10.3389/fchem.2018.00407
![]() |
[35] |
Dris R, Gasperi J, Mirande C, et al. (2017) A first overview of textile fibers, including microplastics, in indoor and outdoor environments. Environ Pollut 221: 453-458. doi: 10.1016/j.envpol.2016.12.013
![]() |
[36] |
Prata JC (2018) Airborne microplastics: Consequences to human health? Environ Pollut 234: 115-126. doi: 10.1016/j.envpol.2017.11.043
![]() |
[37] |
Toussaint B, Raffael B, Angers-Loustau A, et al. (2019) Review of micro- and nanoplastic contamination in the food chain. Food Addit Contam Part A Chem Anal Control Expo Risk Assess 36: 639-673. doi: 10.1080/19440049.2019.1583381
![]() |
[38] |
Cox KD, Covernton GA, Davies HL, et al. (2019) Human Consumption of Microplastics. Environ Sci Technol 53: 7068-7074. doi: 10.1021/acs.est.9b01517
![]() |
[39] |
Lehner R, Weder C, Petri-Fink A, et al. (2019) Emergence of Nanoplastic in the Environment and Possible Impact on Human Health. Environ Sci Technol 53: 1748-1765. doi: 10.1021/acs.est.8b05512
![]() |
[40] | Schwabl P, Liebmann B, Koppel S, et al. (2018) Assessment of microplastic concentrations in human stool-Preliminary results of a prospective study. United European Gastroenterol J 6: A127. |
[41] |
Brook RD, Brook JR, Rajagopalan S (2003) Air pollution: the "Heart" of the problem. Curr Hypertens Rep 5: 32-39. doi: 10.1007/s11906-003-0008-y
![]() |
[42] |
Ohlwein S, Kappeler R, Kutlar Joss M, et al. (2019) Health effects of ultrafine particles: a systematic literature review update of epidemiological evidence. Int J Public Health 64: 547-559. doi: 10.1007/s00038-019-01202-7
![]() |
[43] |
Stapleton PA, Minarchick VC, McCawley M, et al. (2012) Xenobiotic particle exposure and microvascular endpoints: a call to arms. Microcirculation 19: 126-142. doi: 10.1111/j.1549-8719.2011.00137.x
![]() |
[44] |
Stapleton PA, Minarchick VC, Cumpston AM, et al. (2012) Impairment of coronary arteriolar endothelium-dependent dilation after multi-walled carbon nanotube inhalation: a time-course study. Int J Mol Sci 13: 13781-13803. doi: 10.3390/ijms131113781
![]() |
[45] |
Porter DW, Hubbs AF, Mercer RR, et al. (2010) Mouse pulmonary dose- and time course-responses induced by exposure to multi-walled carbon nanotubes. Toxicology 269: 136-147. doi: 10.1016/j.tox.2009.10.017
![]() |
[46] |
Mastrangelo G, Fedeli U, Fadda E, et al. (2002) Epidemiologic evidence of cancer risk in textile industry workers: a review and update. Toxicol Ind Health 18: 171-181. doi: 10.1191/0748233702th139rr
![]() |
[47] |
Porter DW, Castranova V, Robinson VA, et al. (1999) Acute inflammatory reaction in rats after intratracheal instillation of material collected from a nylon flocking plant. J Toxicol Environ Health A 57: 25-45. doi: 10.1080/009841099157845
![]() |
[48] | D'Errico JN, Fournier SB, Stapleton PA (2019) Ex vivo perfusion of the rodent placenta. J Vis Exp: e59412. |
[49] |
Som C, Wick P, Krug H, et al. (2011) Environmental and health effects of nanomaterials in nanotextiles and facade coatings. Environ Int 37: 1131-1142. doi: 10.1016/j.envint.2011.02.013
![]() |
[50] | Lim SL, Ng CT, Zou L, et al. (2019) Targeted metabolomics reveals differential biological effects of nanoplastics and nanoZnO in human lung cells. Nanotoxicology: 1-34. |
[51] |
Schirinzi GF, Perez-Pomeda I, Sanchis J, et al. (2017) Cytotoxic effects of commonly used nanomaterials and microplastics on cerebral and epithelial human cells. Environ Res 159: 579-587. doi: 10.1016/j.envres.2017.08.043
![]() |
[52] |
Forte M, Iachetta G, Tussellino M, et al. (2016) Polystyrene nanoparticles internalization in human gastric adenocarcinoma cells. Toxicol In Vitro 31: 126-136. doi: 10.1016/j.tiv.2015.11.006
![]() |
[53] |
Parenti CC, Ghilardi A, Della Torre C, et al. (2019) Evaluation of the infiltration of polystyrene nanobeads in zebrafish embryo tissues after short-term exposure and the related biochemical and behavioural effects. Environ Pollut 254: 112947. doi: 10.1016/j.envpol.2019.07.115
![]() |
[54] |
Grafmueller S, Manser P, Diener L, et al. (2015) Transfer studies of polystyrene nanoparticles in the ex vivo human placenta perfusion model: key sources of artifacts. Sci Technol Adv Mater 16: 044602. doi: 10.1088/1468-6996/16/4/044602
![]() |
[55] |
Grafmueller S, Manser P, Diener L, et al. (2015) Bidirectional Transfer Study of Polystyrene Nanoparticles across the Placental Barrier in an ex Vivo Human Placental Perfusion Model. Environ Health Perspect 123: 1280-1286. doi: 10.1289/ehp.1409271
![]() |
[56] | Vogel SA (2009) The politics of plastics: the making and unmaking of bisphenol a "safety". Am J Public Health 99 Suppl 3: S559-566. |
[57] |
Moon MK (2019) Concern about the Safety of Bisphenol A Substitutes. Diabetes Metab J 43: 46-48. doi: 10.4093/dmj.2019.0027
![]() |
[58] |
Liu B, Lehmler HJ, Sun Y, et al. (2019) Association of Bisphenol A and Its Substitutes, Bisphenol F and Bisphenol S, with Obesity in United States Children and Adolescents. Diabetes Metab J 43: 59-75. doi: 10.4093/dmj.2018.0045
![]() |
[59] |
Liu B, Lehmler HJ, Sun Y, et al. (2017) Bisphenol A substitutes and obesity in US adults: analysis of a population-based, cross-sectional study. Lancet Planet Health 1: e114-e122. doi: 10.1016/S2542-5196(17)30049-9
![]() |
[60] |
Pelch K, Wignall JA, Goldstone AE, et al. (2019) A scoping review of the health and toxicological activity of bisphenol A (BPA) structural analogues and functional alternatives. Toxicology 424: 152235. doi: 10.1016/j.tox.2019.06.006
![]() |
[61] |
Siracusa JS, Yin L, Measel E, et al. (2018) Effects of bisphenol A and its analogs on reproductive health: A mini review. Reprod Toxicol 79: 96-123. doi: 10.1016/j.reprotox.2018.06.005
![]() |
[62] |
Usman A, Ikhlas S, Ahmad M (2019) Occurrence, toxicity and endocrine disrupting potential of Bisphenol-B and Bisphenol-F: A mini-review. Toxicol Lett 312: 222-227. doi: 10.1016/j.toxlet.2019.05.018
![]() |
1. | Hong-Min Qin, Denise Herrera, Dian-Feng Liu, Chao-Qian Chen, Armen Nersesyan, Miroslav Mišík, Siegfried Knasmueller, Genotoxic properties of materials used for endoprostheses: Experimental and human data, 2020, 145, 02786915, 111707, 10.1016/j.fct.2020.111707 | |
2. | Sara B. Fournier, Jeanine N. D’Errico, Derek S. Adler, Stamatina Kollontzi, Michael J. Goedken, Laura Fabris, Edward J. Yurkow, Phoebe A. Stapleton, Nanopolystyrene translocation and fetal deposition after acute lung exposure during late-stage pregnancy, 2020, 17, 1743-8977, 10.1186/s12989-020-00385-9 | |
3. | Dotan Shaniv, Ishai Dror, Brian Berkowitz, Effects of particle size and surface chemistry on plastic nanoparticle transport in saturated natural porous media, 2021, 262, 00456535, 127854, 10.1016/j.chemosphere.2020.127854 | |
4. | Quinn T. Birch, Phillip M. Potter, Patricio X. Pinto, Dionysios D. Dionysiou, Souhail R. Al-Abed, Sources, transport, measurement and impact of nano and microplastics in urban watersheds, 2020, 19, 1569-1705, 275, 10.1007/s11157-020-09529-x | |
5. | Marco Capolupo, Paola Valbonesi, Elena Fabbri, A Comparative Assessment of the Chronic Effects of Micro- and Nano-Plastics on the Physiology of the Mediterranean Mussel Mytilus galloprovincialis, 2021, 11, 2079-4991, 649, 10.3390/nano11030649 | |
6. | Kanawat Wiwatchaitawee, Juliana C. Quarterman, Sean M. Geary, Aliasger K. Salem, Enhancement of Therapies for Glioblastoma (GBM) Using Nanoparticle-based Delivery Systems, 2021, 22, 1530-9932, 10.1208/s12249-021-01928-9 | |
7. | Philip J. Landrigan, John J. Stegeman, Lora E. Fleming, Denis Allemand, Donald M. Anderson, Lorraine C. Backer, Françoise Brucker-Davis, Nicolas Chevalier, Lilian Corra, Dorota Czerucka, Marie-Yasmine Dechraoui Bottein, Barbara Demeneix, Michael Depledge, Dimitri D. Deheyn, Charles J. Dorman, Patrick Fénichel, Samantha Fisher, Françoise Gaill, François Galgani, William H. Gaze, Laura Giuliano, Philippe Grandjean, Mark E. Hahn, Amro Hamdoun, Philipp Hess, Bret Judson, Amalia Laborde, Jacqueline McGlade, Jenna Mu, Adetoun Mustapha, Maria Neira, Rachel T. Noble, Maria Luiza Pedrotti, Christopher Reddy, Joacim Rocklöv, Ursula M. Scharler, Hariharan Shanmugam, Gabriella Taghian, Jeroen A. J. M. Van de Water, Luigi Vezzulli, Pál Weihe, Ariana Zeka, Hervé Raps, Patrick Rampal, Human Health and Ocean Pollution, 2020, 86, 2214-9996, 151, 10.5334/aogh.2831 | |
8. | Lawrence P. Wackett, Serina L. Robinson, The ever-expanding limits of enzyme catalysis and biodegradation: polyaromatic, polychlorinated, polyfluorinated, and polymeric compounds, 2020, 477, 0264-6021, 2875, 10.1042/BCJ20190720 | |
9. | Huanliang Liu, Lijie Tian, Man Qu, Dayong Wang, Acetylation regulation associated with the induction of protective response to polystyrene nanoparticles in Caenorhabditis elegans, 2021, 411, 03043894, 125035, 10.1016/j.jhazmat.2020.125035 | |
10. | Chan-Wei Yu, Tin Chi Luk, Vivian Hsiu-Chuan Liao, Long-term nanoplastics exposure results in multi and trans-generational reproduction decline associated with germline toxicity and epigenetic regulation in Caenorhabditis elegans, 2021, 412, 03043894, 125173, 10.1016/j.jhazmat.2021.125173 | |
11. | C.C. Parenti, A. Binelli, S. Caccia, C. Della Torre, S. Magni, G. Pirovano, M. Casartelli, Ingestion and effects of polystyrene nanoparticles in the silkworm Bombyx mori, 2020, 257, 00456535, 127203, 10.1016/j.chemosphere.2020.127203 | |
12. | Bin Zhang, Jinyu Chao, Liang Chen, Lingchen Liu, Xin Yang, Qing Wang, Research progress of nanoplastics in freshwater, 2021, 757, 00489697, 143791, 10.1016/j.scitotenv.2020.143791 | |
13. | Amrita Banerjee, Weilin L. Shelver, Micro- and nanoplastic induced cellular toxicity in mammals: A review, 2021, 755, 00489697, 142518, 10.1016/j.scitotenv.2020.142518 | |
14. | Shinji Kihara, Ingo Köper, Jitendra P. Mata, Duncan J. McGillivray, Reviewing nanoplastic toxicology: It's an interface problem, 2021, 288, 00018686, 102337, 10.1016/j.cis.2020.102337 | |
15. | Lesley Henderson, Emeka Dumbili, ‘Drinking and Dropping’: On Interacting with Plastic Pollution and Waste in South-Eastern Nigeria, 2021, 4, 2399-7117, 10.5334/wwwj.59 | |
16. | Maxine Swee-Li Yee, Ling-Wei Hii, Chin King Looi, Wei-Meng Lim, Shew-Fung Wong, Yih-Yih Kok, Boon-Keat Tan, Chiew-Yen Wong, Chee-Onn Leong, Impact of Microplastics and Nanoplastics on Human Health, 2021, 11, 2079-4991, 496, 10.3390/nano11020496 | |
17. | Lingmei Sun, Kai Liao, Dayong Wang, Comparison of transgenerational reproductive toxicity induced by pristine and amino modified nanoplastics in Caenorhabditis elegans, 2021, 768, 00489697, 144362, 10.1016/j.scitotenv.2020.144362 | |
18. | Chang-Bum Jeong, Hye-Min Kang, Eunjin Byeon, Min-Sub Kim, Sung Yong Ha, Moonkoo Kim, Jee-Hyun Jung, Jae-Seong Lee, Phenotypic and Transcriptomic Responses of the Rotifer Brachionus koreanus by Single and Combined Exposures to Nano-Sized Microplastics and Water-Accommodated Fractions of Crude Oil, 2021, 03043894, 125703, 10.1016/j.jhazmat.2021.125703 | |
19. | Lesley Henderson, Emeka Dumbili, Perceptions of Plastics Pollution and Waste amongst young people in Nigeria, 2020, 1556-5068, 10.2139/ssrn.3832532 | |
20. | Heesang Shin, Chang-Bum Jeong, Metabolism deficiency and oxidative stress induced by plastic particles in the rotifer Brachionus plicatilis: Common and distinct phenotypic and transcriptomic responses to nano- and microplastics, 2022, 182, 0025326X, 113981, 10.1016/j.marpolbul.2022.113981 | |
21. | Nabanita Guha, Shirin Walke, Padma Thiagarajan, 2022, 9780323899376, 151, 10.1016/B978-0-323-89937-6.00004-8 | |
22. | Dušan Materić, Elke Ludewig, Dominik Brunner, Thomas Röckmann, Rupert Holzinger, Nanoplastics transport to the remote, high-altitude Alps, 2021, 288, 02697491, 117697, 10.1016/j.envpol.2021.117697 | |
23. | Jakob Quade, Sara López-Ibáñez, Ricardo Beiras, Mesocosm trials reveal the potential toxic risk of degrading bioplastics to marine life, 2022, 179, 0025326X, 113673, 10.1016/j.marpolbul.2022.113673 | |
24. | Dušan Materić, Mike Peacock, Joshua Dean, Martyn Futter, Trofim Maximov, Filip Moldan, Thomas Röckmann, Rupert Holzinger, Presence of nanoplastics in rural and remote surface waters, 2022, 17, 1748-9326, 054036, 10.1088/1748-9326/ac68f7 | |
25. | Gaston Casillas, Brian Charles Hubbard, Jana Telfer, Max Zarate-Bermudez, Custodio Muianga, Gregory M. Zarus, Yulia Carroll, April Ellis, Candis M. Hunter, Microplastics Scoping Review of Environmental and Human Exposure Data, 2023, 2, 2673-8929, 78, 10.3390/microplastics2010006 | |
26. | Bhavya Shri Subramaniam Ramasamy, Shanmugam Palanisamy, A review on occurrence, characteristics, toxicology and treatment of nanoplastic waste in the environment, 2021, 28, 0944-1344, 43258, 10.1007/s11356-021-14883-6 | |
27. | Irene Barguilla, Josefa Domenech, Laura Rubio, Ricard Marcos, Alba Hernández, Nanoplastics and Arsenic Co-Exposures Exacerbate Oncogenic Biomarkers under an In Vitro Long-Term Exposure Scenario, 2022, 23, 1422-0067, 2958, 10.3390/ijms23062958 | |
28. | Cassandra Johannessen, Shegufa Shetranjiwalla, 2021, Chapter 80, 978-3-030-88341-6, 123, 10.1007/398_2021_80 | |
29. | Luhua Yang, Xianting Wang, Jun Ma, Gang Li, Lili Wei, G. Daniel Sheng, Nanoscale polystyrene intensified the microbiome perturbation and antibiotic resistance genes enrichment in soil and Enchytraeus crypticus caused by tetracycline, 2022, 174, 09291393, 104426, 10.1016/j.apsoil.2022.104426 | |
30. | Shinji Kihara, Alexander Ashenden, Manmeet Kaur, Judith Glasson, Sunandita Ghosh, Nadine van der Heijden, Anna E. S. Brooks, Jitendra P. Mata, Stephen Holt, Laura J. Domigan, Ingo Köper, Duncan J. McGillivray, Cellular interactions with polystyrene nanoplastics—The role of particle size and protein corona, 2021, 16, 1934-8630, 041001, 10.1116/6.0001124 | |
31. | Sanchari Biswas, Devarati Bagchi, Debjit Ghosh, 2022, chapter 8, 9781799897231, 148, 10.4018/978-1-7998-9723-1.ch008 | |
32. | A. Götz, S. Beggel, J. Geist, Dietary exposure to four sizes of spherical polystyrene, polylactide and silica nanoparticles does not affect mortality, behaviour, feeding and energy assimilation of Gammarus roeseli, 2022, 238, 01476513, 113581, 10.1016/j.ecoenv.2022.113581 | |
33. | Lilia S. Lens-Pechakova, Recent studies on enzyme-catalysed recycling and biodegradation of synthetic polymers, 2021, 4, 25425048, 151, 10.1016/j.aiepr.2021.06.005 | |
34. | Chenxu Yu, Paul Takhistov, Evangelyn Alocilja, Jose Reyes de Corcuera, Margaret W. Frey, Carmen L. Gomes, Yu J. Mao, Eric S. McLamore, Mengshi Lin, Olga V. Tsyusko, Tzuen-Rong J. Tzeng, Jeong-Yeol Yoon, Anhong Zhou, Bioanalytical approaches for the detection, characterization, and risk assessment of micro/nanoplastics in agriculture and food systems, 2022, 414, 1618-2642, 4591, 10.1007/s00216-022-04069-5 | |
35. | Anthony Andrady, Liping Zhu, 2021, Chapter 17, 978-3-030-75601-7, 373, 10.1007/978-3-030-75602-4_17 | |
36. | Eleanor A. Medley, Miranda J. Spratlen, Beizhan Yan, Julie B. Herbstman, Maya A. Deyssenroth, A Systematic Review of the Placental Translocation of Micro- and Nanoplastics, 2023, 2196-5412, 10.1007/s40572-023-00391-x | |
37. | Stephanie Reynaud, Antoine Aynard, Bruno Grassl, Julien Gigault, Nanoplastics: From model materials to colloidal fate, 2022, 57, 13590294, 101528, 10.1016/j.cocis.2021.101528 | |
38. | Keiron P. Roberts, Sui C. Phang, John B. Williams, David J. Hutchinson, Simon E. Kolstoe, Jasper de Bie, Ian D. Williams, Anne M. Stringfellow, Increased personal protective equipment litter as a result of COVID-19 measures, 2021, 5, 2398-9629, 272, 10.1038/s41893-021-00824-1 | |
39. | Sukhendu Maity, Sambuddha Banerjee, Chayan Biswas, Rajkumar Guchhait, Ankit Chatterjee, Kousik Pramanick, Functional interplay between plastic polymers and microbes: a comprehensive review, 2021, 32, 0923-9820, 487, 10.1007/s10532-021-09954-x | |
40. | Prosper Naah Angnunavuri, Francis Attiogbe, Bismark Mensah, Particulate plastics in drinking water and potential human health effects: Current knowledge for management of freshwater plastic materials in Africa, 2023, 316, 02697491, 120714, 10.1016/j.envpol.2022.120714 | |
41. | Manikantha D, C. D. S. L. N. Tulasi, Kalyani Chepuri, 2023, Chapter 12, 978-3-031-21194-2, 261, 10.1007/978-3-031-21195-9_12 | |
42. | Ratul Kumar Das, Doyeli Sanyal, Pratik Kumar, Rama Pulicharla, Satinder Kaur Brar, Science-society-policy interface for microplastic and nanoplastic: Environmental and biomedical aspects, 2021, 290, 02697491, 117985, 10.1016/j.envpol.2021.117985 | |
43. | Huanliang Liu, Lijie Tian, Shuting Wang, Dayong Wang, Size-dependent transgenerational toxicity induced by nanoplastics in nematode Caenorhabditis elegans, 2021, 790, 00489697, 148217, 10.1016/j.scitotenv.2021.148217 | |
44. | Nanotoxicity: Can We Use Traditionary Methods?, 2022, 20, 18165230, 10.15407/nnn.20.01.279 | |
45. | Josefa Domenech, Constanza Cortés, Lourdes Vela, Ricard Marcos, Alba Hernández, Polystyrene Nanoplastics as Carriers of Metals. Interactions of Polystyrene Nanoparticles with Silver Nanoparticles and Silver Nitrate, and Their Effects on Human Intestinal Caco-2 Cells, 2021, 11, 2218-273X, 859, 10.3390/biom11060859 | |
46. | Saugat Shiwakoti, Ju-Young Ko, Dalseong Gong, Bikalpa Dhakal, Jeong-Hye Lee, Radhika Adhikari, Yeonhyang Gwak, Sin-Hee Park, Ik Jun Choi, Valérie B. Schini-Kerth, Ki-Woon Kang, Min-Ho Oak, Effects of polystyrene nanoplastics on endothelium senescence and its underlying mechanism, 2022, 164, 01604120, 107248, 10.1016/j.envint.2022.107248 | |
47. | Priscila Gubert, Greici Gubert, Ronei Cardoso de Oliveira, Isabel Cristina Oliveira Fernandes, Iverson Conrado Bezerra, Bruna de Ramos, Milena Ferreira de Lima, Daniela Teixeira Rodrigues, Adriana Farias Nunes da Cruz, Ernesto Chaves Pereira, Daiana Silva Ávila, Dante Homero Mosca, Caenorhabditis elegans as a Prediction Platform for Nanotechnology-Based Strategies: Insights on Analytical Challenges, 2023, 11, 2305-6304, 239, 10.3390/toxics11030239 | |
48. | Yuting Zhang, Shunlan Wang, Volovych Olga, Yijia Xue, Shuguo Lv, Xiaoping Diao, Yingai Zhang, Qian Han, Hailong Zhou, The potential effects of microplastic pollution on human digestive tract cells, 2022, 291, 00456535, 132714, 10.1016/j.chemosphere.2021.132714 | |
49. | Amrita Banerjee, Weilin L. Shelver, Micro- and Nanoplastic-Mediated Pathophysiological Changes in Rodents, Rabbits, and Chickens: A Review, 2021, 84, 0362028X, 1480, 10.4315/JFP-21-117 | |
50. | Sergio Caputi, Francesca Diomede, Paola Lanuti, Guya Diletta Marconi, Piero Di Carlo, Bruna Sinjari, Oriana Trubiani, Microplastics Affect the Inflammation Pathway in Human Gingival Fibroblasts: A Study in the Adriatic Sea, 2022, 19, 1660-4601, 7782, 10.3390/ijerph19137782 | |
51. | Xuan Li, Lin Lu, Shaoguo Ru, Junho Eom, Dong Wang, Jun Wang, Nanoplastics induce more severe multigenerational life-history trait changes and metabolic responses in marine rotifer Brachionus plicatilis: Comparison with microplastics, 2023, 449, 03043894, 131070, 10.1016/j.jhazmat.2023.131070 | |
52. | Sahadevan Seena, Irene B. Gutiérrez, Juliana Barros, Cláudia Nunes, João Carlos Marques, Santosh Kumar, Ana M.M. Gonçalves, Impacts of low concentrations of nanoplastics on leaf litter decomposition and food quality for detritivores in streams, 2022, 429, 03043894, 128320, 10.1016/j.jhazmat.2022.128320 | |
53. | I. Brandts, M. Cánovas, A. Tvarijonaviciute, M. Llorca, A. Vega, M. Farré, J. Pastor, N. Roher, M. Teles, Nanoplastics are bioaccumulated in fish liver and muscle and cause DNA damage after a chronic exposure, 2022, 212, 00139351, 113433, 10.1016/j.envres.2022.113433 | |
54. | Michael F. Hughes, Haley M. Clapper, Robert M. Burgess, Kay T. Ho, Human and ecological health effects of nanoplastics: May not be a tiny problem, 2021, 28, 24682020, 43, 10.1016/j.cotox.2021.09.004 | |
55. | Bhoomika Yadav, Shraddha Chavan, Anusha Atmakuri, R.D. Tyagi, Patrick Drogui, 2023, 9780323999083, 59, 10.1016/B978-0-323-99908-3.00008-7 | |
56. | Simranjeet Singh, T. S. Sunil Kumar Naik, Amith G. Anil, Jaskaran Dhiman, Vijay Kumar, Daljeet Singh Dhanjal, Liliana Aguilar-Marcelino, Joginder Singh, Praveen C. Ramamurthy, Micro (nano) plastics in wastewater: A critical review on toxicity risk assessment, behaviour, environmental impact and challenges, 2022, 290, 00456535, 133169, 10.1016/j.chemosphere.2021.133169 | |
57. | Weilin Wang, Jinlong Zhang, Zhiqiang Qiu, Zeyang Cui, Ningqi Li, Xin Li, Yawei Wang, Haixia Zhang, Chunyan Zhao, Effects of polyethylene microplastics on cell membranes: A combined study of experiments and molecular dynamics simulations, 2022, 429, 03043894, 128323, 10.1016/j.jhazmat.2022.128323 | |
58. | Yunhan Yang, Qiuli Wu, Dayong Wang, Neuronal Gα subunits required for the control of response to polystyrene nanoparticles in the range of μg/L in C. elegans, 2021, 225, 01476513, 112732, 10.1016/j.ecoenv.2021.112732 | |
59. | Philip J. Landrigan, Hervé Raps, Maureen Cropper, Caroline Bald, Manuel Brunner, Elvia Maya Canonizado, Dominic Charles, Thomas C. Chiles, Mary J. Donohue, Judith Enck, Patrick Fenichel, Lora E. Fleming, Christine Ferrier-Pages, Richard Fordham, Aleksandra Gozt, Carly Griffin, Mark E. Hahn, Budi Haryanto, Richard Hixson, Hannah Ianelli, Bryan D. James, Pushpam Kumar, Amalia Laborde, Kara Lavender Law, Keith Martin, Jenna Mu, Yannick Mulders, Adetoun Mustapha, Jia Niu, Sabine Pahl, Yongjoon Park, Maria-Luiza Pedrotti, Jordan Avery Pitt, Mathuros Ruchirawat, Bhedita Jaya Seewoo, Margaret Spring, John J. Stegeman, William Suk, Christos Symeonides, Hideshige Takada, Richard C. Thompson, Andrea Vicini, Zhanyun Wang, Ella Whitman, David Wirth, Megan Wolff, Aroub K. Yousuf, Sarah Dunlop, The Minderoo-Monaco Commission on Plastics and Human Health, 2023, 89, 2214-9996, 10.5334/aogh.4056 | |
60. | Chan-Wei Yu, Yi-Chun Wu, Vivian Hsiu-Chuan Liao, Nanoplastics exposure disrupts circadian rhythm associated with dysfunction of the endolysosomal pathway and autophagy in Caenorhabditis elegans, 2023, 452, 03043894, 131308, 10.1016/j.jhazmat.2023.131308 | |
61. | Christian Ayafor, Allen C. Chang, Akanksha Patel, Umer Abid, Dongming Xie, Margaret J. Sobkowicz, Hsi‐Wu Wong, In‐Situ Product Removal for the Enzymatic Depolymerization of Poly(ethylene terephthalate) via a Membrane Reactor, 2024, 1864-5631, 10.1002/cssc.202400698 | |
62. | Erwin Pavel Lamparelli, Marianna Marino, Marta Anna Szychlinska, Natalia Della Rocca, Maria Camilla Ciardulli, Pasqualina Scala, Raffaella D'Auria, Antonino Testa, Andrea Viggiano, Francesco Cappello, Rosaria Meccariello, Giovanna Della Porta, Antonietta Santoro, The Other Side of Plastics: Bioplastic-Based Nanoparticles for Drug Delivery Systems in the Brain, 2023, 15, 1999-4923, 2549, 10.3390/pharmaceutics15112549 | |
63. | Muhammad Tariq, Babar Iqbal, Ismail Khan, Ali Raza Khan, Eun Hea Jho, Abdul Salam, Huan Zhou, Xin Zhao, Guanlin Li, Daolin Du, Microplastic contamination in the agricultural soil—mitigation strategies, heavy metals contamination, and impact on human health: a review, 2024, 43, 0721-7714, 10.1007/s00299-024-03162-6 | |
64. | Aref Yarahmadi, SeyedeMozhgan Heidari, Parisa Sepahvand, Hamed Afkhami, Hadis Kheradjoo, Microplastics and environmental effects: investigating the effects of microplastics on aquatic habitats and their impact on human health, 2024, 12, 2296-2565, 10.3389/fpubh.2024.1411389 | |
65. | Vethanayaham Jebashalomi, Partheeban Emmanuel Charles, Rajendran Rajaram, Paramasivam Sadayan, A critical review on nanoplastics and its future perspectives in the marine environment, 2023, 195, 0167-6369, 10.1007/s10661-023-11701-z | |
66. | Bosen Zhao, Palizhati Rehati, Zhu Yang, Zongwei Cai, Caixia Guo, Yanbo Li, The potential toxicity of microplastics on human health, 2024, 912, 00489697, 168946, 10.1016/j.scitotenv.2023.168946 | |
67. | Lan Anh Phan Thi, Van-Huy Nguyen, Xuan Duc Do, Thi Hai Linh Dang, Huu Tuan Do, Thuy Linh Nguyen, 2025, 9780443298042, 451, 10.1016/B978-0-443-29804-2.00020-2 | |
68. | Abdulkarim Hasan Rashed, Gamze Yesilay, Layla Hazeem, Suad Rashdan, Reem AlMealla, Zeynep Kilinc, Fatema Ali, Fatima Abdulrasool, Ayman H. Kamel, Micro- and Nano-Plastics Contaminants in the Environment: Sources, Fate, Toxicity, Detection, Remediation, and Sustainable Perspectives, 2023, 15, 2073-4441, 3535, 10.3390/w15203535 | |
69. | Anastasia Voronkova, Isabel Richter, Lesley Henderson, John Jamir Benzon R. Aruta, E. Dumbili, Kayleigh J. Wyles, Sabine Pahl, 2023, 9780323952279, 427, 10.1016/B978-0-323-95227-9.00011-7 | |
70. | Sofía Navarro-Espinoza, Erika Silva-Campa, Mónica Alessandra Acosta-Elías, Francisco Javier Grijalva-Noriega, Micro(nano)plásticos en el medio ambiente: una descripción de los efectos potenciales a la salud humana, 2023, 18, 2007-8196, 10.36790/epistemus.v18i35.311 | |
71. | Wanqing Zhao, Tong Ye, Jianwen Zhou, Xuan Zhang, Ke Wang, Hong Zhang, Jiansheng Cui, Shuping Zhang, Lixin Wang, Hydrogen bonding-mediated interaction underlies the enhanced membrane toxicity of chemically transformed polystyrene microplastics by cadmium, 2024, 478, 03043894, 135562, 10.1016/j.jhazmat.2024.135562 | |
72. | Buket Bakan, Nikolina Kalčec, Sijin Liu, Krunoslav Ilić, Yu Qi, Ivona Capjak, Lucija Božičević, Nikolina Peranić, Ivana Vinković Vrček, Science-based evidence on pathways and effects of human exposure to micro- and nanoplastics, 2024, 75, 1848-6312, 1, 10.2478/aiht-2024-75-3807 | |
73. | Antonio Cid-Samamed, Catarina S. E. Nunes, Cristina Lomas Martínez, Mário S. Diniz, Development of a New Aggregation Method to Remove Nanoplastics from the Ocean: Proof of Concept Using Mussel Exposure Tests, 2024, 9, 2313-7673, 303, 10.3390/biomimetics9050303 | |
74. | Ruiping Wang, Shizhong Yue, Caide Huang, Li Jia, Cevin Tibihenda, Zhenghua Li, Jiafeng Yu, Visual mapping of global nanoplastics research progresses and hotspots: a scientometric assessment analysis, 2023, 30, 1614-7499, 114739, 10.1007/s11356-023-30597-3 | |
75. | Junfang Wang, Zhichao Yan, Yulong Lu, Wei Xie, Liying Sui, Size-dependent toxicity of microplastics induced gut cell apoptosis in brine shrimp Artemia parthenogenetica through p53 signaling pathway, 2024, 2096-5508, 10.1007/s00343-024-4066-0 | |
76. | Magdalena Madeła, 2023, 9781119879503, 381, 10.1002/9781119879534.ch23 | |
77. | Shahin Shajahan, Karthikeyan Perumal, S. Selvam, 2024, 9780323909808, 43, 10.1016/B978-0-323-90980-8.00003-0 | |
78. | Sania Afrose, Thi Kim Anh Tran, Wayne O'Connor, Logeshwaran Pannerselvan, Maddison Carbery, Stewart Fielder, Suresh Subhaschandrabose, Thava Palanisami, Organ-specific distribution and size-dependent toxicity of polystyrene nanoplastics in Australian bass (Macquaria novemaculeata), 2024, 341, 02697491, 122996, 10.1016/j.envpol.2023.122996 | |
79. | Sarah Suda Petters, Eva Rosendal Kjærgaard, Freja Hasager, Andreas Massling, Marianne Glasius, Merete Bilde, Morphology and hygroscopicity of nanoplastics in sea spray, 2023, 25, 1463-9076, 32430, 10.1039/D3CP03793B | |
80. | Kerry Candlen, Gregory Reimonn, Md. Akiful Haque, Olivia Hosterman, Wan-Ting Chen, Biofilm-influenced weathering of polypropylene films submerged in field samples from freshwater and marine ecosystems, 2024, 12, 22133437, 112284, 10.1016/j.jece.2024.112284 | |
81. | Yi-Chun Chen, Ku-Fan Chen, Kun-Yi Andrew Lin, Yiu Fai Tsang, Yu-Fang Hsu, Chia-Hua Lin, Evaluation of the pulmonary toxicity of PSNPs using a Transwell-based normal human bronchial epithelial cell culture system, 2023, 895, 00489697, 165213, 10.1016/j.scitotenv.2023.165213 | |
82. | Sabina Vohl, Matjaž Kristl, Janja Stergar, Harnessing Magnetic Nanoparticles for the Effective Removal of Micro- and Nanoplastics: A Critical Review, 2024, 14, 2079-4991, 1179, 10.3390/nano14141179 | |
83. | Diyali Sil, Edin Osmanbasic, Sasthi Charan Mandal, Atanu Acharya, Chayan Dutta, Variable Non-Gaussian Transport of Nanoplastic on Supported Lipid Bilayers in Saline Conditions, 2024, 15, 1948-7185, 5428, 10.1021/acs.jpclett.4c00806 | |
84. | Shuang Li, Wenkang Peng, Yan Guo, Sumei Li, Qunhui Wang, Current status of microplastic pollution and the latest treatment technologies, 2024, 957, 00489697, 177467, 10.1016/j.scitotenv.2024.177467 | |
85. | Giulia Cesarini, Silvia Secco, Davide Taurozzi, Iole Venditti, Chiara Battocchio, Stefania Marcheggiani, Laura Mancini, Ilaria Fratoddi, Massimiliano Scalici, Camilla Puccinelli, Teratogenic effects of environmental concentration of plastic particles on freshwater organisms, 2023, 898, 00489697, 165564, 10.1016/j.scitotenv.2023.165564 | |
86. | Adrienne Jia Wen Gan, Kiat Fatt Chia, Chooi Ling Lim, Boon Keat Tan, Shew Fung Wong, Soi Moi Chye, Chee Onn Leong, Rhun Yian Koh, Neurotoxicity of nanoplastics: A review, 2024, 13, 2046-1402, 793, 10.12688/f1000research.149068.1 | |
87. | Gina M. Moreno, Tanisha Brunson-Malone, Samantha Adams, Calla Nguyen, Talia N. Seymore, Chelsea M. Cary, Marianne Polunas, Michael J. Goedken, Phoebe A. Stapleton, Identification of micro- and nanoplastic particles in postnatal sprague-dawley rat offspring after maternal inhalation exposure throughout gestation, 2024, 951, 00489697, 175350, 10.1016/j.scitotenv.2024.175350 | |
88. | Irene Brandts, Roger Solà, Marlid Garcia-Ordoñez, Alex Gella, Albert Quintana, Beatriz Martin, Anna Esteve-Codina, Mariana Teles, Nerea Roher, Polystyrene nanoplastics target lysosomes interfering with lipid metabolism through the PPAR system and affecting macrophage functionalization, 2023, 10, 2051-8153, 2245, 10.1039/D2EN01077A | |
89. | Claudio Casella, Santiago J. Ballaz, Genotoxic and neurotoxic potential of intracellular nanoplastics: A review, 2024, 44, 0260-437X, 1657, 10.1002/jat.4598 | |
90. | Julia Nieto-Sandoval, Reyhan Ammar, Carmen Sans, Enhancing nanoplastics removal by metal ion-catalyzed ozonation, 2024, 19, 26668211, 100621, 10.1016/j.ceja.2024.100621 | |
91. | Ma Carmen González-Caballero, Mercedes de Alba González, Mónica Torres-Ruiz, Patricia Iglesias-Hernández, Verónica Zapata, María C. Terrón, Martin Sachse, Mónica Morales, Raquel Martin-Folgar, Isabel Liste, Ana I. Cañas-Portilla, Internalization and toxicity of polystyrene nanoplastics on inmortalized human neural stem cells, 2024, 355, 00456535, 141815, 10.1016/j.chemosphere.2024.141815 | |
92. | Pooja Singh, Gunjan Varshney, Raminder Kaur, Primary Microplastics in the Ecosystem: Ecological Effects, Risks, and Comprehensive Perspectives on Toxicology and Detection Methods, 2024, 42, 2689-6583, 314, 10.1080/26896583.2024.2370715 | |
93. | Xinlei Huang, Suvash C. Saha, Goutam Saha, Isabella Francis, Zhen Luo, Transport and deposition of microplastics and nanoplastics in the human respiratory tract, 2024, 16, 26667657, 100525, 10.1016/j.envadv.2024.100525 | |
94. | Marissa S. Giroux, Jay R. Reichman, Troy Langknecht, Robert M. Burgess, Kay T. Ho, Using eRNA/eDNA metabarcoding to detect community-level impacts of nanoplastic exposure to benthic estuarine ecosystems, 2023, 338, 02697491, 122650, 10.1016/j.envpol.2023.122650 | |
95. | Jianzhou Shi, Xianyi Yu, Jinbing Zhao, Tiejun Wang, Na Li, Jinran Yu, Lunguang Yao, Integrated transcriptomics and metabolomics reveal the mechanism of polystyrene nanoplastics toxicity to mice, 2024, 284, 01476513, 116925, 10.1016/j.ecoenv.2024.116925 | |
96. | Kinga Malinowska, Kateryna Tarhonska, Marek Foksiński, Paulina Sicińska, Ewa Jabłońska, Edyta Reszka, Ewelina Zarakowska, Daniel Gackowski, Karolina Górecka, Aneta Balcerczyk, Bożena Bukowska, Impact of Short-Term Exposure to Non-Functionalized Polystyrene Nanoparticles on DNA Methylation and Gene Expression in Human Peripheral Blood Mononuclear Cells, 2024, 25, 1422-0067, 12786, 10.3390/ijms252312786 | |
97. | Joana Cepeda da Silva Antunes, Paula Sobral, Vasco Branco, Marta Martins, Uncovering layer by layer the risk of nanoplastics to the environment and human health, 2024, 1093-7404, 1, 10.1080/10937404.2024.2424156 | |
98. | Flavia Ruggieri, Beatrice Battistini, Angela Sorbo, Marta Senofonte, Veruscka Leso, Ivo Iavicoli, Beatrice Bocca, From food-to-human microplastics and nanoplastics exposure and health effects: a review on food, animal and human monitoring data, 2024, 02786915, 115209, 10.1016/j.fct.2024.115209 | |
99. | Xinlei Wang, Min Lv, Jin Liu, Mingtao Ba, Mingsan Man, Kun Yin, Jing Ding, Xianbo Chang, Lingxin Chen, Size-specific mediation of the physiological responses and degradation ability of microalgae to sulfamerazine by microplastics, 2025, 279, 0166445X, 107257, 10.1016/j.aquatox.2025.107257 | |
100. | Hamid Reza Moradi, Sohrab Heydarian, Sahar Abdollahinezhad, Effects of Nanoplastics and Microplastics on the Health of the Peripheral and Central Nervous System, 2024, 12, 1681-8725, 97, 10.61186/shefa.12.4.97 | |
101. | Ekemini Okon, Irene Brandts, Hayam Djafar, Asta Tvarijonaviciute, Joan Carles Balasch, Mariana Teles, Effects of Sub-Chronic Exposure to Polystyrene Nanoplastics on Lipid and Antioxidant Metabolism in Sparus aurata, 2025, 15, 2076-2615, 562, 10.3390/ani15040562 | |
102. | Carlos Rafael Silva de Oliveira, Gabriela Maestri, Gabriel André Tochetto, Julia Lemos de Oliveira, Ederson Stiegelmaier, Thomas Volkhard Fischer, Ana Paula Serafini Immich, Nanoplastics: Unveiling Contamination Routes and Toxicological Implications for Human Health, 2025, 21, 15734110, 175, 10.2174/0115734110305991240523073849 |