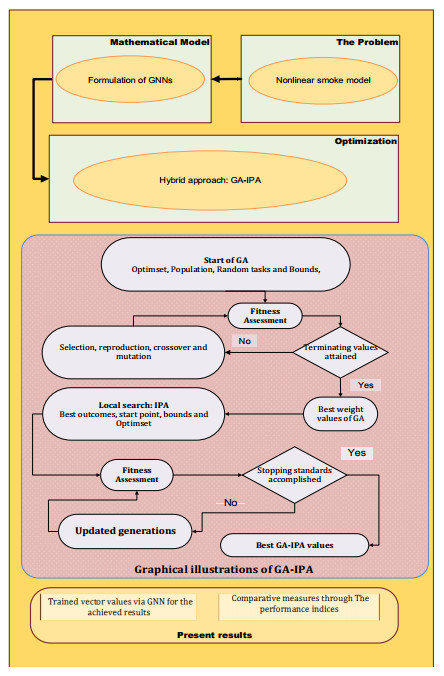
Electroluminescent (EL) and negative electroluminescent (NEL) devices are radiative thermoelectric energy converters that use electric power for refrigeration. For the EL system, we apply a forward bias to the emitter that we want to cool, whereas a reverse bias voltage is applied to the hot absorber for the NEL system. In this work, we derive the thermodynamic limits of the cooling power density and coefficient of performance (COP) of near-field EL and NEL refrigeration systems based on entropy analysis that considers near-field effects. We show numerically that operating the EL and NEL systems in the near-field regime could increase the cooling power density and the COP bounds to a certain extent. As the vacuum gap decrease from 1000 to 10 nm, the near-field effects improve the performance of the NEL system all the time, but the performance of the EL system increases to the optimal value and then decreases. In addition, the increase in temperature difference weakens the performance of both refrigeration systems greatly. Moreover, we also investigate the effects of the absence of sub-bandgap thermal radiation on the performance of the EL and NEL systems. Our work indicates significant opportunities for evaluating the performance of near-field radiative thermoelectric energy converters from the perspective of thermodynamic limits. Meanwhile, these results establish the targets for cooling power density and COP of the near-field EL and NEL systems.
Citation: Bowen Li, Qiang Cheng, Jinlin Song, Kun Zhou, Lu Lu, Zixue Luo. Thermodynamic performance of near-field electroluminescence and negative electroluminescent refrigeration systems[J]. AIMS Energy, 2021, 9(3): 465-482. doi: 10.3934/energy.2021023
[1] | Yuhua Ma, Ye Tao, Yuandan Gong, Wenhua Cui, Bo Wang . Driver identification and fatigue detection algorithm based on deep learning. Mathematical Biosciences and Engineering, 2023, 20(5): 8162-8189. doi: 10.3934/mbe.2023355 |
[2] | Zulqurnain Sabir, Hafiz Abdul Wahab, Juan L.G. Guirao . A novel design of Gudermannian function as a neural network for the singular nonlinear delayed, prediction and pantograph differential models. Mathematical Biosciences and Engineering, 2022, 19(1): 663-687. doi: 10.3934/mbe.2022030 |
[3] | Qingwei Wang, Xiaolong Zhang, Xiaofeng Li . Facial feature point recognition method for human motion image using GNN. Mathematical Biosciences and Engineering, 2022, 19(4): 3803-3819. doi: 10.3934/mbe.2022175 |
[4] | Rami AL-HAJJ, Mohamad M. Fouad, Mustafa Zeki . Evolutionary optimization framework to train multilayer perceptrons for engineering applications. Mathematical Biosciences and Engineering, 2024, 21(2): 2970-2990. doi: 10.3934/mbe.2024132 |
[5] | Biyun Hong, Yang Zhang . Research on the influence of attention and emotion of tea drinkers based on artificial neural network. Mathematical Biosciences and Engineering, 2021, 18(4): 3423-3434. doi: 10.3934/mbe.2021171 |
[6] | Ravi Agarwal, Snezhana Hristova, Donal O’Regan, Radoslava Terzieva . Stability properties of neural networks with non-instantaneous impulses. Mathematical Biosciences and Engineering, 2019, 16(3): 1210-1227. doi: 10.3934/mbe.2019058 |
[7] | Jia-Gang Qiu, Yi Li, Hao-Qi Liu, Shuang Lin, Lei Pang, Gang Sun, Ying-Zhe Song . Research on motion recognition based on multi-dimensional sensing data and deep learning algorithms. Mathematical Biosciences and Engineering, 2023, 20(8): 14578-14595. doi: 10.3934/mbe.2023652 |
[8] | Chun Li, Ying Chen, Zhijin Zhao . Frequency hopping signal detection based on optimized generalized S transform and ResNet. Mathematical Biosciences and Engineering, 2023, 20(7): 12843-12863. doi: 10.3934/mbe.2023573 |
[9] | Yongquan Zhou, Yanbiao Niu, Qifang Luo, Ming Jiang . Teaching learning-based whale optimization algorithm for multi-layer perceptron neural network training. Mathematical Biosciences and Engineering, 2020, 17(5): 5987-6025. doi: 10.3934/mbe.2020319 |
[10] | Jiliang Lv, Chenxi Qu, Shaofeng Du, Xinyu Zhao, Peng Yin, Ning Zhao, Shengguan Qu . Research on obstacle avoidance algorithm for unmanned ground vehicle based on multi-sensor information fusion. Mathematical Biosciences and Engineering, 2021, 18(2): 1022-1039. doi: 10.3934/mbe.2021055 |
Electroluminescent (EL) and negative electroluminescent (NEL) devices are radiative thermoelectric energy converters that use electric power for refrigeration. For the EL system, we apply a forward bias to the emitter that we want to cool, whereas a reverse bias voltage is applied to the hot absorber for the NEL system. In this work, we derive the thermodynamic limits of the cooling power density and coefficient of performance (COP) of near-field EL and NEL refrigeration systems based on entropy analysis that considers near-field effects. We show numerically that operating the EL and NEL systems in the near-field regime could increase the cooling power density and the COP bounds to a certain extent. As the vacuum gap decrease from 1000 to 10 nm, the near-field effects improve the performance of the NEL system all the time, but the performance of the EL system increases to the optimal value and then decreases. In addition, the increase in temperature difference weakens the performance of both refrigeration systems greatly. Moreover, we also investigate the effects of the absence of sub-bandgap thermal radiation on the performance of the EL and NEL systems. Our work indicates significant opportunities for evaluating the performance of near-field radiative thermoelectric energy converters from the perspective of thermodynamic limits. Meanwhile, these results establish the targets for cooling power density and COP of the near-field EL and NEL systems.
According to the reports of the World Health Organization (WHO), there are many deaths occur using the tobacco epidemic and its prey most of the disabled persons during some past few years. The tobacco epidemic not only disturbs the individuals, but also become a reason for increases the health care cost, delays financial development and decreases the families' budgets [1]. The chain smoking is a big reason of death due to oral cavity cancer, bladder, larynx, esophagus, lung, stomach, pancreas, renal pelvis and cervix. The smoking also creates the problems of heart, chronic obstructive, lung weakness, breathing diseases, peripheral vascular and less weight of newly born children. WHO also reports that the reasoning of unproductive pregnancies, peptic ulcer disease and increase the infant mortality rate is due to smoking [2]. The termination of smoking is an instantaneous health support and dramatically reduces the danger of many deathly diseases and improve the respiratory system of the younger. It is the obligation of the higher authorities to teach their people and aware the communities about the drawbacks of smoking as well as develop an active policy to control this habit. Castillo et al. [3] discussed the mathematical model to avoid the smoking by considering the population into two kings, i.e., smokers (S) and those individuals who left smoking permanently (QP). In addition, Shoromi et al. [4] presented a new group temporary smoker (QT) in this mathematical model, which is defined as:
{P'(Ω)=μ(1−P(Ω))−βP(Ω)S(Ω),P(0)=l1,S'(Ω)=βP(Ω)S(Ω)−(γ+μ)S(Ω)+αQT(Ω),S(0)=l2,Q'T(Ω)=γ(1−σ)S(Ω)−(μ+α)QT(Ω),QT(0)=l3,Q'P(Ω)=−μQP(Ω)+γσS(Ω),QP(0)=l4, | (1) |
where P(Ω), S(Ω), QT(Ω) and QP(Ω) indicate the Potential smoker (P) group, Smoker (S) group, Temporary smoker (QT) group and Permanent smoker (QS) group at time Ω. Whereas, σ,α,γ,β and μ represent the positive values of the constants. Furthermore, l1, l2, l3 and l4 designate the initial conditions (ICs) of the nonlinear smoke model (1).
The aim of this work is to investigate the nonlinear smoke model numerically to exploit a stochastic framework called Gudermannian neural works (GNNs) [5,6,7,8] along with the optimization procedures of global/local search terminologies based Genetic algorithm (GA) and interior-point approach (IPA), i.e., GNNs-GA-IPA. The development of the numerical solvers has been reported in various proposals for the solution of the linear/nonlinear differential models with their own applicability, stability and significance [9,10,11,12,13], however recently artificial intelligence based numerical computing platform are introduction as a promising alternatives [14,15,16,17,18]. Whereas, GNNs-GA-IPA is never been applied before to solve the nonlinear smoke system. Some recent proposals of the stochastic solvers are functional singular system [19], nonlinear SIR dengue fever model [20], mathematical models of environmental economic systems [21], prey-predator nonlinear system [22], Thomas-Fermi system [23], mosquito dispersal model [24], transmission of heat in human head [25], multi-singular fractional system [26] and nonlinear COVID-19 model [27]. Some novel prominent features of the current investigations are provided as:
● The GNNs are explored efficaciously using the hybrid optimization paradigm based on GA-IPA for solving the nonlinear smoke model.
● The consistent overlapped outcomes obtained by GNNs-GA-IPA and the Runge-Kutta numerical results validate the correctness and exactness of the proposed scheme.
● The authorization of the performance is accomplished through different statistical valuations to attain the numerical outcomes of the nonlinear smoke system.
The benefits, merits and noteworthy contributions of the GNNs-GA-IPA are simply implemented to solve the nonlinear smoke model, understanding easiness, operated efficiently and inclusive with reliable applications in diversified fields.
The remaining parts of the current work are studied as: Section 2 defines the procedures of GNNs-GA-IPA along with and statistical measures. Section 3 indicates the results simulations. Section 4 provided the final remarks and future research reports.
In this section, the proposed form of the GNNs-GA-IPA is presented in two steps to solve the nonlinear smoke model as:
● A merit function is designed using the differential system and ICs of the nonlinear smoke system.
● The necessary and essential settings are provided for the optimization procedures of GA-IPA to solve the nonlinear smoke model.
In this section, the mathematical formulations to solve the nonlinear smoke model-based groups, Potential smoker (ˆP), Temporary smoker (ˆQT), Permanent smoker (ˆQS) and Smoker (ˆS) are presented. The proposed results of these groups of the nonlinear smoke model, ˆP,ˆS,ˆQT and ˆQS are represented by ˆP,ˆS,ˆQT and ˆQS together with their derivatives are written as:
[ˆP(Ω),ˆS(Ω),ˆQT(Ω),ˆQS(Ω)]=[∑mi=1kP,iT(wP,iΩ+hP,i),∑mi=1aS,iT(wS,iΩ+hS,i),∑mi=1aQT,iT(wQT,iΩ+hQT,i),∑mi=1aQS,iT(wQS,iΩ+hQS,i)],[ˆP'(Ω),ˆS'(Ω),ˆQ'T(Ω),ˆQ'S(Ω)]=[∑mi=1kP,iT'(wP,iΩ+hP,i),∑mi=1kS,iT'(wS,iΩ+hS,i),∑mi=1kQT,iT'(wQT,iΩ+hQT,i),∑mi=1kQS,iT'(wQS,iΩ+hQS,i)]. | (2) |
where W is the unknown weight vector, written as:
W=[WP,WS,WQT,WQS],forWP=[kP,ωP,hP],WS=[kS,ωS,hS],WQT=[kQT,ωQT,hQT] |
and WQS=[kQS,ωQS,hQS], where
kP=[kP,1,kP,2,...,kP,m],kS=[kS,1,kS,2,...,kS,m],kQT=[kQT,1,kQT,2,...,kQT,m],kQS=[kQS,1,kQT,2,...,kQT,m],wP=[wP,1,wP,2,...,wP,m],wS=[wS,1,wS,2,...,wS,m]wQT=[wQT,1,wQT,2,...,wQT,m],wQS=[wQS,1,wQT,2,...,wQT,m],hP=[hP,1,hP,2,...,hP,m]hS=[hS,1,hS,2,...,hS,m],hQT=[hQT,1,hQT,2,...,hQT,m],hQS=[hQS,1,hQT,2,...,hQT,m] |
The Gudermannian function T(Ω)=2tan−1[exp(Ω)]−0.5π is applied in the above model, this GNNs have never been implemented before the solve this model.
[ˆP(Ω),ˆS(Ω),ˆQT(Ω),ˆQS(Ω)]=[∑mi=1kP,i(2tan−1e(wP,iΩ+hP,i)−π2),∑mi=1kS,i(2tan−1e(wS,iΩ+hS,i)−π2),∑mi=1kQT,i(2tan−1e(wQT,iΩ+hQT,i)−π2),∑mi=1kQP,i(2tan−1e(wQS,iΩ+hQS,i)−π2)],[ˆP'(Ω),ˆS'(Ω),ˆQ'T(Ω),ˆQ'S(Ω)]=[∑mi=12kP,iwP,i(e(wp,iΩ+np,i)1+(e(wp,iΩ+np,i))2),∑mi=12kS,iwS,i(e(wS,iΩ+nS,i)1+(e(wS,iΩ+nS,i))2),∑mi=12kQT,iwQT,i(e(wQT,iΩ+nQT,i)1+(e(wQT,iΩ+nQT,i))2),∑mi=12kQS,iwQS,i(e(wQS,iΩ+nQS,i)1+(e(wQS,iΩ+nQS,i))2)], | (3) |
For the process of optimization, a fitness function is given as:
ΞFit=Ξ1+Ξ2+Ξ3+Ξ4+Ξ5, | (4) |
Ξ2=1N∑Ni=1[ˆS'i−βˆPiˆSi+μˆSi+γˆSi−α(ˆQT)i]2, | (5) |
Ξ1=1N∑Ni=1[ˆP'i−μ+μˆPi+βˆPiˆSi]2, | (6) |
Ξ3=1N∑Ni=1[(ˆQ'T)i+γσˆSi−γˆSk+α(ˆQT)i+μ(ˆQT)i]2, | (7) |
Ξ4=1N∑Ni=1[(ˆQ'P)i−γσˆSi+μ(ˆQP)i]2, | (8) |
Ξ5=14[(ˆP0−l1)2+(ˆS0−l2)2+((ˆQT)0−l3)2++((ˆQS)0−l4)2], | (9) |
where ˆPi=P(Ωi),ˆSi=S(Ωi),(QT)i=QT(Ωi),(QS)i=QS(Ωi),TN=1, and Ωi=ih. The error functions Ξ1, Ξ2, Ξ3 and Ξ4 are related to system (1), while, Ξ5 is based on the ICs of the nonlinear smoke model (1).
In this section, the performance of the scheme is observed using the optimization process of GA-IPA to solve the nonlinear smoke system. The designed GNNs-GA-IPA methodology based on the nonlinear smoke system is illustrated in Figure 1.
GA is known as a famous global search optimization method applied to solve the solve the constrained/unconstrained models efficiently. It is commonly implemented to regulate the precise population outcomes for solving the various stiff and complex models using the optimal training process. For the best solutions of the model, GA is implemented through the process of selection, reproduction, mutation and crossover procedures. Recently, GA is applied in many famous applications that can be seen in [28,29,30,31,32] and references cited therein.
IPA is a local search, rapid and quick optimization method, implemented to solve various reputed complex and non-stiff models efficiently. IPA is implemented in various models like phase-field approach to brittle and ductile fracture [33], multistage nonlinear nonconvex programs [34], SITR model for dynamics of novel coronavirus [35], viscoplastic fluid flows [36] and security constrained optimal power flow problems [37]. To control the Laziness of the global search method GA, the process of hybridization with the IPA is applied for solving the nonlinear smoke model. The detailed pseudocode based on the GNNs-GA-IPA is provided in Table 1.
GA process starts Inputs: The chromosomes of the same network elements are denoted as: W = [k, w, h] Population: Chromosomes set is represented as: W=[WP,WS,WQT,WQS], WP=[kP,ωP,hP], WS=[kS,ωS,hS], WQT=[kQT,ωQT,hQT] and WQS=[kQS,ωQS,hQS], W is the weight vector Outputs: The best global weight values are signified as: WGA-Best Initialization: For the chromosome's assortment, adjust the W Assessment of FIT: Adjust "ΞFit" in the population (P) for each vector values using Eqs (4)–(9). ● Stopping standards: Terminate if any of the criteria is achieved [ΞFit = 10-19], [Generataions = 120] [TolCon = TolFun = 10-18], [StallLimit = 150], & [Size of population = 270]. Move to [storage] Ranking: Rank precise W in the particular population for ΞFit. Storage: Save ΞFit, iterations, WGA-Best, function counts and time. GA procedure End |
Start of IPA Inputs: WGA-Best is selected as an initial point. Output: WGA-IPA shows the best weights of GA-IPA. Initialize: WGA-Best, generations, assignments and other standards. Terminating criteria: Stop if [ΞFit = 10-20], [TolFun = 10-18], [Iterations = 700], [TolCon = TolX = 10-22] and [MaxFunEvals = 260000] attained. Evaluation of Fit: Compute the values of W and E for Eqs (4)–(9). Amendments: Normalize 'fmincon' for IPA, compute ΞFit for Eqs (4)–(9). Accumulate: Transmute WGA-IPA, time, iterations, function counts and ΞFit for the IPA trials. |
IPA process End |
The mathematical presentations using the statistical operators with "variance account for (VAF)", "Theil's inequality coefficient (TIC)", "mean absolute deviation (MAD)" and "semi interquartile (SI) range" together with the global operators G.VAF, G-TIC G-MAD are accessible to solve the nonlinear smoke model, written as:
{[V.A.FP,V.A.FS,V.A.FQT,V.A.FQS]=[(1−var(Pm−ˆPm)var(Pm))×100,(1−var(Sm−ˆSm)var(Sm))×100,(1−var((QT)m−(ˆQT)m)var(QT)m)×100,(1−var((QS)m−(ˆQS)m)var(QS)m)×100],[E-V.A.FP,E-V.A.FS,E-V.A.FQT,E-V.A.FQS]=[|100−V.A.FP,100−V.A.FS,100−V.A.FQT,100−V.A.FQS|]. | (10) |
[T.I.CP,T.I.CS,T.I.CQT,T.I.CQS]=[√1n∑nm=1(Pm−ˆPm)2(√1n∑nm=1P2m+√1n∑nm=1ˆP2m),√1n∑nm=1(Sm−ˆSm)2(√1n∑nm=1S2m+√1n∑nm=1ˆS2m),√1n∑nm=1((QT)m−(ˆQT)m)2(√1n∑nm=1(QT)2m+√1n∑nm=1(ˆQT)2m),√1n∑nm=1((QS)m−(ˆQS)m)2(√1n∑nm=1(QS)2m+√1n∑nm=1(ˆQS)2m)], | (11) |
[M.A.DP,M.A.DS, M.A.DQT, M.A.DQS]=[∑nm=1|Pm−ˆPm|,∑nm=1|Sm−ˆSm|,∑nm=1|(QT)m−(ˆQT)m|,∑nm=1|(QS)m−(ˆQS)m|] | (12) |
{S.I Range=−0.5×(Q1−Q3),Q1 = 1stquartileQ3=3rdquartile. | (13) |
ˆP,ˆS,ˆQT and ˆQS are the approximate form of the solutions.
The current investigations are associated to solve the nonlinear smoke model. The relative performance of the obtained solutions with the Runge-Kutta results is tested to show the exactness of the GNNs-GA-IPA. Moreover, the statistical operator's performances are used to validate the accuracy, reliability and precision of the proposed GNNs-GA-IPA. The updated form of the nonlinear smoke model given in the system (1) along with its ICs using the appropriate parameter values is shown as:
{P'(Ω)=20−(20P(Ω)+0.003P(Ω)S(Ω)),P(0)=0.3,S'(Ω)=0.003P(Ω)S(Ω)−20.3S(Ω)+3QT(Ω),S(0)=0.5,Q'T(Ω)=0.15S(Ω)−23QT(Ω),QT(0)=0.1,Q'P(Ω)=0.15S(Ω)−20QP(Ω),QP(0)=0.2, | (14) |
A fitness function for the nonlinear smoke model (14) is written as:
ΞFit=1N∑Nm=1([ˆP'm+20ˆPm−20+0.003ˆPmˆSm]2+[ˆS'm+20.3ˆSm−0.003ˆPmˆSm−3(QT)m]2+[(Q'T)m+23(QT)m−0.15ˆSm]2+[(Q'P)m+20(QP)m−0.15ˆSm]2)+14[(ˆP0−0.3)2+(ˆS0−0.5)2+((QT)0−0.1)2+((QP)0−0.2)2]. | (15) |
The performance of the scheme is observed based on the nonlinear smoke system using the GNNs-GA-IPA for 20 independent executions using 30 numbers of variables. The proposed form of the solution based on the nonlinear smoke model is provided in the arrangement of best weights using the below equations for each group of the nonlinear smoke model and the graphical illustrations of these weights are plotted in Figure 2.
ˆP(Ω)=7.2965(2tan−1e(−5.343Ω−18.462)−0.5π)−2.7636(2tan−1e1.2606Ω+0.5134)−0.5π)+15.294(2tan−1e(1.1551Ω+2.0601)−0.5π)+8.7324(2tan−1e(19.759Ω+2.9789)−0.5π)−7.1646(2tan−1e(2.5198Ω+5.9852)−0.5π)−0.0053(2tan−1e(−0.246Ω−1.7692)−0.5π)−2.6104(2tan−1e(0.2323Ω+1.5497)−0.5π)−1.4677(2tan−1e(02.782Ω+2.0890)−0.5π)+2.4715(2tan−1e(−18516Ω−3.253)−0.5π)−2.9189(2tan−1e(10.272Ω−7.2465)−0.5π), | (16) |
ˆS(Ω)=7.6303(2tan−1e(−0.143Ω+0.4605)−0.5π)+9.6607(2tan−1e(0.264Ω+0.8726)−0.5π)−1.8710(2tan−1e(0.3252Ω−1.0967)−0.5π)+0.0451(2tan−1e(−1.961Ω+8.4961)−0.5π)+5.4711(2tan−1e(−9.8369Ω−1.533)−0.5π)−1.7275(2tan−1e(−9.2138Ω−2.037)−0.5π)+0.2908(2tan−1e(−2.4236Ω−0.378)−0.5π)−1.9171(2tan−1e(−9.2376Ω−0.360)−0.5π)+2.7144(2tan−1e(2.8428Ω−7.8525)−0.5π)−5.3000(2tan−1e(−4.6148Ω+4.164)−0.5π), | (17) |
ˆQT(Ω)=−0.563(2tan−1e(−3.245Ω+2.3616)−0.5π)−0.022(2tan−1e(−8.9859+1.2373)−0.5π)−0.5360(2tan−1e(3.2982Ω−2.4088)−0.5π)−2.1382(2tan−1e(−3.87Ω−3.100)−0.5π)−4.7812(2tan−1e(−4.1182Ω−4.994)−0.5π)+0.9505(2tan−1e(−0.038Ω−0.241)−0.5π)+17.841(2tan−1e(−14.7536Ω−4.557)−0.5π)−3.4575(2tan−1e(4.347Ω+0.7343)−0.5π)+11.8361(2tan−1e(4.5451Ω+2.013)−0.5π)+2.717(2tan−1e(3.6697Ω+7.33831)−0.5π), | (18) |
ˆQS(Ω)=0.0762(2tan−1e(2.9990Ω+2.8605)−0.5π)+2.8390(2tan−1e(8.3177Ω+0.6804)−0.5π)+7.6702(2tan−1e(−0.0481Ω+1.9373)−0.5π)−0.4142(2tan−1e(−0.309Ω−0.401)−0.5π)−6.2827(2tan−1e(1.2291Ω+3.5918)−0.5π)+0.5077(2tan−1e(1.0574Ω+1.0166)−0.5π)+2.2061(2tan−1e(1.5133Ω+10.7487)−0.5π)−0.2776(2tan−1e(1.7337Ω+5.1928)−0.5π)−5.3623(2tan−1e(8.4564Ω+1.3100)−0.5π)+0.0684(2tan−1e(−4.1583Ω−1.6646)−0.5π), | (19) |
A fitness function shown in the model (15) is optimized along with the hybridization of GA-IPA for the nonlinear smoke system. The proposed form of the outcomes is found using the above systems (16)–(19) for 30 variables between 0 to 1 input along with step size 0.1. The solutions of the nonlinear smoke model along with the best weight vector values are illustrated in Figures 2(a–d). The comparison of the mean and best outcomes with the reference Runge-Kutta solutions is provided in Figures 2(e–h) to solve the nonlinear smoke system. It is noticed that the mean and best results obtained by the GNNs-GA-IPA are overlapped with the reference results to solve each group of the nonlinear smoke model, which authenticate the exactness of the designed GNNs-GA-IPA. Figure 3 illustrates the values of the absolute error (AE) for each group of the nonlinear smoke model. It is observed that the values of the best AE for the group of potential smokers, smoker; temporary smoker and permanent smoker lie around 10-05-10-07, 10-05-10-06, 10-04-10-07 and 10-04-10-06, respectively. While, the mean AE values for these groups of the nonlinear smoke model found around 10-03-10-04, 10-03-10-05, 10-02-10-04 and 10-03-10-04, respectively. Figure 4 signifies the performance measures based on the operators EVAF, MAD and TIC to solve each group of the nonlinear smoke model. It is specified in the plots that the best values of the EVAF, MAD and TIC performances of each group of the nonlinear smoke model lie around 10-04-10-08, 10-03-10-05 and 10-08-10-09, respectively. The best performances of the EVAF, MAD and TIC for the ˆP(Ω) and ˆS(Ω) groups lie around 10-08-10-09, 10-05-10-06 and 10-09-10-10, respectively. The best performances of the EVAF, MAD and TIC for the ˆQT(Ω) group of the nonlinear smoke model found around 10-05-10-06, 10-04-10-06 and 10-08-10-10 and the best performances of the EVAF, MAD and TIC for the ˆQP(Ω) group of the nonlinear smoke model found around 10-07-10-08, 10-05-10-06 and 10-09-10-10. One can accomplish from the indications that the designed GNNs-GA-IPA is precise and accurate.
The graphic illustrations based on the statistical performances are provided in Figures 5–7 to find the convergence along with the boxplots and the histograms to solve the nonlinear smoke model. Figure 5 shows the performance of TIC for twenty runs to solve each group of the nonlinear smoke model. It is observed that most of the executions for the ˆP,ˆS,ˆQT and ˆQS groups lie around 10-07-10-10. The MAD performances are illustrated in Figure 6 that depicts most of the executions for the ˆP,ˆS,ˆQT and ˆQS groups lie around 10-03-10-05. The EVAF performances are illustrated in Figure 7 that depicts most of the executions for the ˆP,ˆS,ˆQT and ˆQS groups lie around 10-04-10-08. The best trial performances using the GNNs-GA-IPA are calculated suitable for the TIC, EVAF and MAD operators.
The routines for different statistical operators, Maximum (Max), Median, Minimum (Min), standard deviation (STD) and SIR are provided in Tables 2–5 to validate the accurateness and precision to solve the ˆP,ˆS,ˆQT and ˆQS groups of the nonlinear smoke system. The Max operators indicate the worst solutions, whereas the Min operators show the best results using 20 independent runs. For the group P(Ω), S(Ω), QT(Ω) and QS(Ω) of the nonlinear smoke model, the 'Max' and 'Min' standards lie around 10-03-10-04 and 10-06-10-08, while the Median, SIR, STD and Mean standards lie around 10-04-10-05. These small values designate the worth and values of the GNNs-GA-IPA to solve each group of the nonlinear smoke model. One can observe through these calculate measures, that the designed GNNs-GA-IPA is precise, accurate and stable.
Ω | P(Ω) | |||||
Max | Min | Median | STD | SIR | Mean | |
0 | 1.9698E-03 | 1.5128E-07 | 1.3179E-05 | 4.3988E-04 | 2.8322E-05 | 1.5255E-04 |
0.1 | 2.2927E-03 | 4.0452E-05 | 3.7091E-04 | 7.5870E-04 | 3.5757E-04 | 7.1580E-04 |
0.2 | 2.8517E-04 | 2.5715E-06 | 8.0076E-05 | 1.0291E-04 | 8.7298E-05 | 1.0480E-04 |
0.3 | 3.7540E-04 | 5.0221E-06 | 4.6631E-05 | 9.6615E-05 | 5.7759E-05 | 8.0291E-05 |
0.4 | 3.1095E-04 | 7.2215E-07 | 2.5169E-05 | 7.9915E-05 | 4.8322E-05 | 6.4497E-05 |
0.5 | 3.7039E-04 | 1.0187E-07 | 4.0958E-05 | 9.9187E-05 | 4.0049E-05 | 7.4737E-05 |
0.6 | 3.4788E-04 | 2.7497E-06 | 3.6783E-05 | 8.6814E-05 | 5.3154E-05 | 7.3405E-05 |
0.7 | 7.2524E-04 | 3.8591E-06 | 2.6741E-05 | 1.6887E-04 | 5.3778E-05 | 9.7130E-05 |
0.8 | 3.1439E-04 | 2.3193E-06 | 3.0315E-05 | 8.9765E-05 | 3.8282E-05 | 6.8701E-05 |
0.9 | 8.2385E-04 | 3.6579E-06 | 3.6581E-05 | 1.8035E-04 | 2.2583E-05 | 8.9806E-05 |
1 | 3.9210E-04 | 8.9543E-07 | 1.4825E-05 | 1.1497E-04 | 3.4368E-05 | 6.7763E-05 |
Ω | S(Ω) | |||||
Max | Min | Median | STD | SIR | Mean | |
0 | 2.2510E-03 | 8.8331E-07 | 1.5049E-05 | 4.3988E-04 | 6.5800E-05 | 2.4625E-04 |
0.1 | 3.5749E-03 | 1.2621E-05 | 4.2711E-04 | 7.5870E-04 | 5.9138E-04 | 9.2693E-04 |
0.2 | 5.8967E-04 | 6.8508E-06 | 5.0534E-05 | 1.0291E-04 | 3.8159E-05 | 1.0389E-04 |
0.3 | 3.9412E-04 | 4.5897E-07 | 5.5860E-05 | 9.6615E-05 | 4.8082E-05 | 9.7091E-05 |
0.4 | 1.8148E-04 | 1.4261E-06 | 3.2627E-05 | 7.9915E-05 | 4.6977E-05 | 6.1366E-05 |
0.5 | 3.8406E-04 | 3.0499E-06 | 5.0425E-05 | 9.9187E-05 | 4.5676E-05 | 9.6411E-05 |
0.6 | 3.3693E-04 | 1.0786E-06 | 3.2525E-05 | 8.6814E-05 | 2.6474E-05 | 5.9807E-05 |
0.7 | 3.8976E-04 | 5.9658E-08 | 3.4605E-05 | 1.6887E-04 | 3.0731E-05 | 7.1943E-05 |
0.8 | 4.6451E-04 | 1.8083E-07 | 2.9100E-05 | 8.9765E-05 | 1.9639E-05 | 5.1752E-05 |
0.9 | 3.7056E-04 | 5.8454E-07 | 3.1691E-05 | 1.8035E-04 | 4.5808E-05 | 7.8891E-05 |
1 | 3.4425E-04 | 3.5833E-08 | 3.7245E-05 | 1.1497E-04 | 2.5924E-05 | 6.3636E-05 |
Ω | QT(Ω) | |||||
Max | Min | Median | STD | SIR | Mean | |
0 | 6.7379E-02 | 2.0435E-06 | 7.4367E-05 | 4.3988E-04 | 1.2547E-03 | 6.6320E-03 |
0.1 | 8.8212E-03 | 1.5933E-04 | 6.6896E-04 | 7.5870E-04 | 6.4736E-04 | 1.4858E-03 |
0.2 | 1.2208E-03 | 1.0177E-06 | 1.6681E-04 | 1.0291E-04 | 1.3929E-04 | 2.7755E-04 |
0.3 | 1.2039E-03 | 6.2163E-06 | 3.5966E-05 | 9.6615E-05 | 7.5659E-05 | 2.0075E-04 |
0.4 | 5.2048E-04 | 1.5004E-06 | 9.3199E-05 | 7.9915E-05 | 1.4812E-04 | 1.7703E-04 |
0.5 | 7.9163E-04 | 4.1214E-06 | 7.5760E-05 | 9.9187E-05 | 9.4190E-05 | 1.7072E-04 |
0.6 | 7.5113E-04 | 3.4495E-06 | 5.6836E-05 | 8.6814E-05 | 1.5865E-04 | 1.8051E-04 |
0.7 | 6.9564E-04 | 2.1601E-06 | 8.7570E-05 | 1.6887E-04 | 1.2891E-04 | 1.6565E-04 |
0.8 | 8.5659E-04 | 2.3356E-06 | 5.6424E-05 | 8.9765E-05 | 6.5358E-05 | 1.2238E-04 |
0.9 | 8.5832E-04 | 1.8869E-06 | 8.6636E-05 | 1.8035E-04 | 1.2103E-04 | 1.8340E-04 |
1 | 5.9922E-04 | 9.8962E-07 | 3.9188E-05 | 1.1497E-04 | 4.8965E-05 | 1.1550E-04 |
Ω | QS(Ω) | |||||
Max | Min | Median | STD | SIR | Mean | |
0 | 1.4249E-02 | 4.6038E-07 | 1.0193E-05 | 3.1752E-03 | 5.3007E-05 | 7.6325E-04 |
0.1 | 7.1456E-03 | 9.4140E-06 | 2.0718E-04 | 1.6018E-03 | 3.9882E-04 | 8.1152E-04 |
0.2 | 1.4449E-03 | 2.1615E-07 | 2.8536E-05 | 3.6835E-04 | 3.0447E-05 | 1.7480E-04 |
0.3 | 3.7091E-04 | 3.2754E-07 | 2.1791E-05 | 8.7324E-05 | 2.6186E-05 | 5.6609E-05 |
0.4 | 1.5545E-03 | 1.8846E-07 | 2.2389E-05 | 3.7612E-04 | 6.1143E-05 | 1.5810E-04 |
0.5 | 3.7972E-04 | 3.6651E-06 | 1.7656E-05 | 9.8654E-05 | 3.1268E-05 | 6.2521E-05 |
0.6 | 1.2224E-03 | 2.4032E-07 | 2.1050E-05 | 2.9478E-04 | 3.3053E-05 | 1.2732E-04 |
0.7 | 7.4101E-04 | 1.7539E-07 | 2.4276E-05 | 1.6625E-04 | 2.5398E-05 | 7.7130E-05 |
0.8 | 1.0726E-03 | 2.5213E-06 | 1.4100E-05 | 2.3771E-04 | 3.0541E-05 | 9.1329E-05 |
0.9 | 7.4407E-04 | 2.5770E-06 | 3.5282E-05 | 1.6258E-04 | 2.8865E-05 | 7.9482E-05 |
1 | 9.7616E-04 | 7.0693E-07 | 8.9328E-06 | 2.1858E-04 | 1.0034E-05 | 8.0351E-05 |
The global performances of the G.EVAF, G.MAD and G.TIC operators for twenty runs to solve the designed GNNs-GA-IPA are provided in Table 6 to solve each group of the nonlinear smoke model. These Min global G.MAD, G.TIC and G.EVAF performances found around 10-04-10-05, 10-08-10-09 and 10-05-10-07, whereas the SIR global values lie in the ranges of 10-04-10-05, 10-08-10-09 and 10-04-10-07 for all groups of the nonlinear smoke model. These close optimal global measures values demonstrate the correctness, accurateness and precision of the proposed GNNs-GA-IPA.
Class | (G.MAD) | (G.TIC) | (G.EVAF) | |||
Min | SIR | Min | SIR | Min | SIR | |
P(Ω) | 1.2499E-04 | 9.6058E-05 | 7.2597E-09 | 7.4459E-09 | 2.6155E-07 | 7.5331E-07 |
S(Ω) | 9.7570E-05 | 1.3353E-04 | 8.3068E-08 | 1.0766E-08 | 1.3879E-06 | 5.2293E-06 |
QT(Ω) | 1.4111E-04 | 2.5334E-04 | 9.1566E-09 | 1.8187E-08 | 3.9553E-05 | 5.1487E-04 |
QS(Ω) | 6.0979E-05 | 4.1189E-05 | 3.6367E-09 | 4.8515E-09 | 1.1015E-06 | 9.3296E-06 |
The current investigations are related to solve the nonlinear smoke model by exploiting the Gudermannian neural networks using the global and local search methodologies, i.e., GNNs-GA-IPA. The smoke model is a system of nonlinear equations contain four groups temporary smokers, potential smokers, permanent smokers and smokers. For the numerical outcomes, a fitness function is established using all groups of the nonlinear smoke model and its corresponding ICs. The optimization of the fitness function using the hybrid computing framework of GNNs-GA-IPA for solving each group of the nonlinear smoke model. The Gudermannian function is designed as a merit function along with 30 numbers of variables. The overlapping of the proposed mean and best outcomes is performed with the Runge-Kutta reference results for each group of the nonlinear smoke model. These matching and reliable results to solve the nonlinear smoke model indicate the exactness of the designed GNNs-GA-IPA. In order to show the precision and accuracy of the proposed GNNs-GA-IPA, the statistical performances based on the TIC, MAD and EVAF operators have been accessible for twenty trials using 10 numbers of neurons. To check the performance analysis, most of the runs based on the statistical TIC, MAD and EVAF performances show a higher level of accuracy to solve each group of the nonlinear smoke model. The valuations using the statistical gages of Max, Min, Mean, STD, Med and SIR further validate the value of the proposed GNNs-GA-IPA. Furthermore, global presentations through SIR and Min have been applied for the nonlinear smoke model.
In future, the designed GNNs-GA-IPA is accomplished to solve the biological nonlinear systems [38], singular higher order model [39], fluid dynamics nonlinear models [40] and fractional differential model [41].
The authors extend their appreciation to the Deanship of Scientific Research at Imam Mohammad Ibn Saud Islamic University for funding this work through Research Group no. RG-21-09-12.
All authors of the manuscript declare that there have no potential conflicts of interest.
[1] |
Polder D, Van Hove M (1971) Theory of Radiative Heat Transfer between Closely Spaced Bodies. Phys Rev B 4: 3303-3314. doi: 10.1103/PhysRevB.4.3303
![]() |
[2] |
Loomis JJ, Maris HJ (1994) Theory of heat transfer by evanescent electromagnetic waves. Phys Rev B 50: 18517-18524. doi: 10.1103/PhysRevB.50.18517
![]() |
[3] |
Pendry JB (1999) Radiative exchange of heat between nanostructures. J Phys-Condens Mat 11: 6621-6633. doi: 10.1088/0953-8984/11/35/301
![]() |
[4] |
Fu CJ, Zhang ZM (2006) Nanoscale radiation heat transfer for silicon at different doping levels. Int J Heat Mass Tran 49: 1703-1718. doi: 10.1016/j.ijheatmasstransfer.2005.09.037
![]() |
[5] |
Volokitin AI, Persson BNJ (2007) Near-field radiative heat transfer and noncontact friction. Rev Mod Phys 79: 1291-1329. doi: 10.1103/RevModPhys.79.1291
![]() |
[6] |
Hu L, Narayanaswamy A, Chen X, et al. (2008) Near-field thermal radiation between two closely spaced glass plates exceeding Planck's blackbody radiation law. Appl Phys Lett 92: 133106. doi: 10.1063/1.2905286
![]() |
[7] |
Basu S, Zhang ZM, Fu CJ (2009) Review of near-field thermal radiation and its application to energy conversion. Int J Energ Res 33: 1203-1232. doi: 10.1002/er.1607
![]() |
[8] |
Rousseau E, Siria A, Jourdan G, et al. (2009) Radiative heat transfer at the nanoscale. Nat Photonics 3: 514-517. doi: 10.1038/nphoton.2009.144
![]() |
[9] |
Shen S, Narayanaswamy A, Chen G (2009) Surface Phonon Polaritons Mediated Energy Transfer between Nanoscale Gaps. Nano Lett 9: 2909-2913. doi: 10.1021/nl901208v
![]() |
[10] |
Qu W, Mudawar I (2002) Experimental and numerical study of pressure drop and heat transfer in a single-phase micro-channel heat sink. Int J Heat Mass Tran 45: 2549-2565. doi: 10.1016/S0017-9310(01)00337-4
![]() |
[11] |
Kittel A, Muller-Hirsch W, Parisi J, et al. (2005) Near-field heat transfer in a scanning thermal microscope. Phys Rev Lett 95: 224301. doi: 10.1103/PhysRevLett.95.224301
![]() |
[12] |
Ottens RS, Quetschke V, Wise S, et al. (2011) Near-field radiative heat transfer between macroscopic planar surfaces. Phys Rev Lett 107: 014301. doi: 10.1103/PhysRevLett.107.014301
![]() |
[13] |
St-Gelais R, Guha B, Zhu L, et al. (2014) Demonstration of strong near-field radiative heat transfer between integrated nanostructures. Nano Lett 14: 6971-6975. doi: 10.1021/nl503236k
![]() |
[14] |
Song B, Fiorino A, Meyhofer E, et al. (2015) Near-field radiative thermal transport: From theory to experiment. Aip Adv 5: 053503. doi: 10.1063/1.4919048
![]() |
[15] |
Laroche M, Carminati R, Greffet JJ (2006) Near-field thermophotovoltaic energy conversion. J Appl Phys 100: 063704. doi: 10.1063/1.2234560
![]() |
[16] |
Svetovoy VB, Palasantzas G (2014) Graphene-on-Silicon near-field thermophotovoltaic cell. Phys Rev Appl 2: 034006 doi: 10.1103/PhysRevApplied.2.034006
![]() |
[17] |
Chang JY, Yang Y, Wang LP (2015) Tungsten nanowire based hyperbolic metamaterial emitters for near-field thermophotovoltaic applications. Int J Heat Mass Tran 87: 237-247. doi: 10.1016/j.ijheatmasstransfer.2015.03.087
![]() |
[18] |
Lenert A, Bierman DM, Nam Y, et al. (2014) A nanophotonic solar thermophotovoltaic device. Nat Nanotechnol 9: 126-130. doi: 10.1038/nnano.2013.286
![]() |
[19] |
Fiorino A, Zhu L, Thompson D, et al. (2018) Nanogap near-field thermophotovoltaics. Nat Nanotechnol 13: 806-811. doi: 10.1038/s41565-018-0172-5
![]() |
[20] |
Lin C, Wang B, Teo KH, et al. (2017) Near-field enhancement of thermoradiative devices. J Appl Phys 122: 143102. doi: 10.1063/1.5007036
![]() |
[21] |
Wang B, Lin C, Teo KH, et al. (2017) Thermoradiative device enhanced by near-field coupled structures. J. Quant Spectros Radiat Transfer 196: 10-16. doi: 10.1016/j.jqsrt.2017.03.038
![]() |
[22] |
Liao T, Zhang X, Yang Z, et al. (2019) Near-Field Thermoradiative Electron Device. IEEE Trans Electron Devices 66: 3099-3102. doi: 10.1109/TED.2019.2915765
![]() |
[23] |
Chen K, Santhanam P, Sandhu S, et al. (2015) Heat-flux control and solid-state cooling by regulating chemical potential of photons in near-field electromagnetic heat transfer. Phys Rev B 91: 134301. doi: 10.1103/PhysRevB.91.134301
![]() |
[24] |
Liu X, Zhang ZM (2016) High-performance electroluminescent refrigeration enabled by photon tunneling. Nano Energy 26: 353-359. doi: 10.1016/j.nanoen.2016.05.049
![]() |
[25] |
Liao T, Tao C, Chen X, et al. (2019) Parametric optimum design of a near-field electroluminescent refrigerator. J Phys D: Appl Phys 52: 325108. doi: 10.1088/1361-6463/ab2341
![]() |
[26] |
Chen K, Santhanam P, Fan S (2016) Near-Field enhanced negative luminescent refrigeration. Phys Rev Appl 6: 024014. doi: 10.1103/PhysRevApplied.6.024014
![]() |
[27] |
Lin C, Wang B, Teo KH, et al. (2018) A coherent description of thermal radiative devices and its application on the near-field negative electroluminescent cooling. Energy 147: 177-186. doi: 10.1016/j.energy.2018.01.005
![]() |
[28] |
Zhou C, Zhang Y, Qu L, et al. (2020) Near-field negative electroluminescent cooling via nanoparticle doping. J Quant Spectrosc Radiat Transfer 245: 106889-106897. doi: 10.1016/j.jqsrt.2020.106889
![]() |
[29] |
Wurfel P (1982) The chemical potential of radiation. J Phys C Solid State Phys 15: 3967. doi: 10.1088/0022-3719/15/18/012
![]() |
[30] |
Tervo E, Bagherisereshki E, Zhang Z (2017) Near-field radiative thermoelectric energy converters: a review. Front Energy 12: 5-21. doi: 10.1007/s11708-017-0517-z
![]() |
[31] | Tauc J (1957) The share of thermal energy taken from the surroundings in the electro-luminescent energy radiated from a p-n junction. Cechoslovackij Fiziceskij Zurnal 7: 275-276. |
[32] | Dousmanis G, Mueller C, Nelson H, et al. (1964) Evidence of refrigerating action by means of photon emission in semiconductor diodes. Phys Rev 133: A316-A318. |
[33] |
Berdahl P (1985) Radiant refrigeration by semiconductor diodes. J Appl Phys 58: 1369-1374. doi: 10.1063/1.336309
![]() |
[34] |
Mal'Shukov A, Chao K (2001) Opto-thermionic refrigeration in semiconductor heterostructures. Phys Rev Lett 86: 5570. doi: 10.1103/PhysRevLett.86.5570
![]() |
[35] |
Han P, Jin KJ, Zhou YL, et al. (2006) Analysis of optothermionic refrigeration based on semiconductor heterojunction. J Appl Phys 99: 074504. doi: 10.1063/1.2188249
![]() |
[36] |
Yen ST, Lee KC (2010) Analysis of heterostructures for electroluminescent refrigeration and light emitting without heat generation. J Appl Phys 107: 054513. doi: 10.1063/1.3326944
![]() |
[37] |
Oksanen J, Tulkki J (2010) Thermophotonic heat pump—a theoretical model and numerical simulations. J Appl Phys 107: 093106. doi: 10.1063/1.3419716
![]() |
[38] | Santhanam P, Huang D, Gray Jr DJ, et al. (2013) Electro-luminescent cooling: light emitting diodes above unity efficiency. Laser Refrigeration of Solids VI, 863807. |
[39] |
Santhanam P, Gray DJ Jr, Ram RJ (2012) Thermoelectrically pumped light-emitting diodes operating above unity efficiency. Phys Rev Lett 108: 097403. doi: 10.1103/PhysRevLett.108.097403
![]() |
[40] |
Guha B, Otey C, Poitras CB, et al. (2012) Near-field radiative cooling of nanostructures. Nano Lett 12: 4546-4550. doi: 10.1021/nl301708e
![]() |
[41] |
Bewley WW, Jurkovic MJ, Felix CL, et al. (2001) HgCdTe photodetectors with negative luminescent efficiencies > 80%. Appl Phys Lett 78: 3082-3084. doi: 10.1063/1.1370539
![]() |
[42] |
Nash GR, Ashby MK, Lindle JR, et al. (2003) Long wavelength infrared negative luminescent devices with strong Auger suppression. J Appl Phys 94: 7300-7304. doi: 10.1063/1.1625094
![]() |
[43] |
Ashley T, Gordon NT, Nash GR, et al. (2001) Long-wavelength HgCdTe negative luminescent devices. Appl Phys Lett 79: 1136-1138. doi: 10.1063/1.1395521
![]() |
[44] |
Hoffman D, Hood A, Wei Y, et al. (2005) Negative luminescence of long-wavelength InAs∕GaSb superlattice photodiodes. Appl Phys Lett 87: 201103. doi: 10.1063/1.2130536
![]() |
[45] |
Ashley T, Elliott CT, Gordon NT, et al. (1995) Negative luminescence from In1−xAlxSb and CdxHg1−xTe diodes. Infrared Phys Technol 36: 1037-1044. doi: 10.1016/1350-4495(95)00043-7
![]() |
[46] |
Zhu L, Fiorino A, Thompson D, et al. (2019) Near-field photonic cooling through control of the chemical potential of photons. Nature 566: 239-244. doi: 10.1038/s41586-019-0918-8
![]() |
[47] |
Rawat R, Lamba R, Kaushik SC (2017) Thermodynamic study of solar photovoltaic energy conversion: An overview. Renewable Sustainable Energy Rev 71: 630-638. doi: 10.1016/j.rser.2016.12.089
![]() |
[48] |
Ruan XL, Rand SC, Kaviany M (2007) Entropy and efficiency in laser cooling of solids. Phys Rev B 75: 214304. doi: 10.1103/PhysRevB.75.214304
![]() |
[49] |
Hsu WC, Tong JK, Liao B, et al. (2016) Entropic and near-field improvements of thermoradiative cells. Sci Rep 6: 34837. doi: 10.1038/srep34837
![]() |
[50] |
Gribik J, Osterle J (1984) The second law efficiency of solar energy conversion. J Sol Energy Eng 106: 16-21. doi: 10.1115/1.3267555
![]() |
[51] |
Pusch A, Gordon JM, Mellor A, et al. (2019) Fundamental efficiency bounds for the conversion of a radiative heat engine's own emission into work. Phys Rev Appl 12: 064018. doi: 10.1103/PhysRevApplied.12.064018
![]() |
[52] |
Li W, Buddhiraju S, Fan S (2020) Thermodynamic limits for simultaneous energy harvesting from the hot sun and cold outer space. Light Sci Appl 9: 68. doi: 10.1038/s41377-020-0296-x
![]() |
[53] |
Dorofeyev I (2011) Thermodynamic functions of fluctuating electromagnetic fields within a heterogeneous system. Phys Scr 84: 055003. doi: 10.1088/0031-8949/84/05/055003
![]() |
[54] |
Perez-Madrid A, Lapas LC, Rubi JM (2009) Heat exchange between two interacting nanoparticles beyond the fluctuation-dissipation regime. Phys Rev Lett 103: 048301. doi: 10.1103/PhysRevLett.103.048301
![]() |
[55] |
Pérez-Madrid A, Rubí JM, Lapas LC (2008) Heat transfer between nanoparticles: Thermal conductance for near-field interactions. Phys Rev B 77: 155417 doi: 10.1103/PhysRevB.77.155417
![]() |
[56] |
Latella I, Pérez-Madrid A, Lapas LC, et al. (2014) Near-field thermodynamics: Useful work, efficiency, and energy harvesting. J Appl Phys 115: 124307. doi: 10.1063/1.4869744
![]() |
[57] |
Narayanaswamy A, Zheng Y (2013) Theory of thermal nonequilibrium entropy in near-field thermal radiation. Phys Rev B 88: 075412. doi: 10.1103/PhysRevB.88.075412
![]() |
[58] |
Li B, Cheng Q, Song J, et al. (2020) Evaluation of performance of near-field thermophotovoltaic systems based on entropy analysis. J Appl Phys 127: 063103. doi: 10.1063/1.5135729
![]() |
[59] |
Ravindra NM, Srivastava VK (1979) Temperature dependence of the energy gap in semiconductors. J Phys Chem Solids 40: 791-793. doi: 10.1016/0022-3697(79)90162-8
![]() |
[60] | Palik ED (1985) Handbook of Optical Constants of Solids, Orlando: Academic Press. |
[61] |
Johnson PB, Christy RW (1972) Optical Constants of the Noble Metals. Phys Rev B 6: 4370-4379. doi: 10.1103/PhysRevB.6.4370
![]() |
[62] |
Kruger M, Emig T, Kardar M (2011) Nonequilibrium electromagnetic fluctuations: heat transfer and interactions. Phys Rev Lett 106: 210404. doi: 10.1103/PhysRevLett.106.210404
![]() |
[63] |
Otey C, Fan S (2011) Numerically exact calculation of electromagnetic heat transfer between a dielectric sphere and plate. Phys Rev B 84: 245431. doi: 10.1103/PhysRevB.84.245431
![]() |
[64] | Chew WC (1995) Waves and Fields in Inhomogeneous Media, New York: IEEE. |
[65] |
Basu S, Lee BJ, Zhang ZM (2010) Infrared radiative properties of heavily doped silicon at room temperature. J Heat Transfer 132: 023301. doi: 10.1115/1.4000171
![]() |
[66] |
Smith GB (1990) Theory of angular selective transmittance in oblique columnar thin films containing metal and voids. Appl Opt 29: 3685-3693. doi: 10.1364/AO.29.003685
![]() |
[67] | Zhang Z (2007) Nano/microscale heat transfer, New York: McGraw-Hill. |
1. | Wajaree Weera, Thongchai Botmart, Samina Zuhra, Zulqurnain Sabir, Muhammad Asif Zahoor Raja, Salem Ben Said, A Neural Study of the Fractional Heroin Epidemic Model, 2023, 74, 1546-2226, 4453, 10.32604/cmc.2023.033232 | |
2. | Nabeela Anwar, Iftikhar Ahmad, Adiqa Kausar Kiani, Shafaq Naz, Muhammad Shoaib, Muhammad Asif Zahoor Raja, Intelligent solution predictive control strategy for nonlinear hepatitis B epidemic model with delay, 2023, 1745-5030, 1, 10.1080/17455030.2023.2178827 | |
3. | Yue Li, Jianyou Zhao, Zenghua Chen, Gang Xiong, Sheng Liu, A Robot Path Planning Method Based on Improved Genetic Algorithm and Improved Dynamic Window Approach, 2023, 15, 2071-1050, 4656, 10.3390/su15054656 | |
4. | Muneerah AL Nuwairan, Zulqurnain Sabir, Muhammad Asif Zahoor Raja, Anwar Aldhafeeri, An advance artificial neural network scheme to examine the waste plastic management in the ocean, 2022, 12, 2158-3226, 045211, 10.1063/5.0085737 | |
5. | Zulqurnain Sabir, Muhammad Asif Zahoor Raja, Nadia Mumtaz, Irwan Fathurrochman, R. Sadat, Mohamed R. Ali, An Investigation Through Stochastic Procedures for Solving the Fractional Order Computer Virus Propagation Mathematical Model with Kill Signals, 2022, 1370-4621, 10.1007/s11063-022-10963-x | |
6. | Wajaree Weera, Zulqurnain Sabir, Muhammad Asif Zahoor Raja, Salem Ben Said, Maria Emilia Camargo, Chantapish Zamart, Thongchai Botmart, An Artificial Approach for the Fractional Order Rape and Its Control Model, 2023, 74, 1546-2226, 3421, 10.32604/cmc.2023.030996 | |
7. | Zulqurnain Sabir, Dumitru Baleanu, Muhammad Asif Zahoor Raja, Evren Hincal, A hybrid computing approach to design the novel second order singular perturbed delay differential Lane-Emden model, 2022, 97, 0031-8949, 085002, 10.1088/1402-4896/ac7a6a | |
8. | Thongchai Botmart, Zulqurnain Sabir, Muhammad Asif Zahoor Raja, Wajaree weera, Mohamed R. Ali, R. Sadat, Ayman A. Aly, Ali Saad, A hybrid swarming computing approach to solve the biological nonlinear Leptospirosis system, 2022, 77, 17468094, 103789, 10.1016/j.bspc.2022.103789 | |
9. | J. F. Gómez-Aguilar, Zulqurnain Sabir, Manal Alqhtani, Muhammad Umar, Khaled M. Saad, Neuro-Evolutionary Computing Paradigm for the SIR Model Based on Infection Spread and Treatment, 2022, 1370-4621, 10.1007/s11063-022-11045-8 | |
10. | Watcharaporn Cholamjiak, Zulqurnain Sabir, Muhammad Asif Zahoor Raja, Manuel Sánchez-Chero, Dulio Oseda Gago, José Antonio Sánchez-Chero, María-Verónica Seminario-Morales, Marco Antonio Oseda Gago, Cesar Augusto Agurto Cherre, Gilder Cieza Altamirano, Mohamed R. Ali, Artificial intelligent investigations for the dynamics of the bone transformation mathematical model, 2022, 34, 23529148, 101105, 10.1016/j.imu.2022.101105 | |
11. | Zulqurnain Sabir, Salem Ben Said, Qasem Al-Mdallal, Mohamed R. Ali, A neuro swarm procedure to solve the novel second order perturbed delay Lane-Emden model arising in astrophysics, 2022, 12, 2045-2322, 10.1038/s41598-022-26566-4 | |
12. | Tianqi Yu, Lei Liu, Yan-Jun Liu, Observer-based adaptive fuzzy output feedback control for functional constraint systems with dead-zone input, 2022, 20, 1551-0018, 2628, 10.3934/mbe.2023123 | |
13. | Prem Junsawang, Zulqurnain Sabir, Muhammad Asif Zahoor Raja, Soheil Salahshour, Thongchai Botmart, Wajaree Weera, Novel Computing for the Delay Differential Two-Prey and One-Predator System, 2022, 73, 1546-2226, 249, 10.32604/cmc.2022.028513 | |
14. | Chenyang Hu, Yuelin Gao, Fuping Tian, Suxia Ma, A Relaxed and Bound Algorithm Based on Auxiliary Variables for Quadratically Constrained Quadratic Programming Problem, 2022, 10, 2227-7390, 270, 10.3390/math10020270 | |
15. | Rahman Ullah, Qasem Al Mdallal, Tahir Khan, Roman Ullah, Basem Al Alwan, Faizullah Faiz, Quanxin Zhu, The dynamics of novel corona virus disease via stochastic epidemiological model with vaccination, 2023, 13, 2045-2322, 10.1038/s41598-023-30647-3 | |
16. | Thanasak Mouktonglang, Zulqurnain Sabir, Muhammad Asif Zahoor Raja, Saira Bhatti, Thongchai Botmart, Wajaree Weera, Chantapish Zamart, Designing Meyer wavelet neural networks for the three-species food chain model, 2023, 8, 2473-6988, 61, 10.3934/math.2023003 | |
17. | Lihe Hu, Yi Zhang, Yang Wang, Qin Jiang, Gengyu Ge, Wei Wang, A simple information fusion method provides the obstacle with saliency labeling as a landmark in robotic mapping, 2022, 61, 11100168, 12061, 10.1016/j.aej.2022.06.002 | |
18. | Sakda Noinang, Zulqurnain Sabir, Shumaila Javeed, Muhammad Asif Zahoor Raja, Dostdar Ali, Wajaree Weera, Thongchai Botmart, A Novel Stochastic Framework for the MHD Generator in Ocean, 2022, 73, 1546-2226, 3383, 10.32604/cmc.2022.029166 | |
19. | Suthep Suantai, Zulqurnain Sabir, Muhammad Asif Zahoor Raja, Watcharaporn Cholamjiak, Numerical Computation of SEIR Model for the Zika Virus Spreading, 2023, 75, 1546-2226, 2155, 10.32604/cmc.2023.034699 | |
20. | Zulqurnain Sabir, Juan L. G. Guirao, A Soft Computing Scaled Conjugate Gradient Procedure for the Fractional Order Majnun and Layla Romantic Story, 2023, 11, 2227-7390, 835, 10.3390/math11040835 | |
21. | Kanit Mukdasai, Zulqurnain Sabir, Muhammad Asif Zahoor Raja, Peerapongpat Singkibud, R. Sadat, Mohamed R. Ali, A computational supervised neural network procedure for the fractional SIQ mathematical model, 2023, 1951-6355, 10.1140/epjs/s11734-022-00738-9 | |
22. | Jingzhi Tu, Gang Mei, Francesco Piccialli, An improved Nyström spectral graph clustering using k-core decomposition as a sampling strategy for large networks, 2022, 34, 13191578, 3673, 10.1016/j.jksuci.2022.04.009 | |
23. | Thongchai Botmart, Qusain Hiader, Zulqurnain Sabir, Muhammad Asif Zahoor Raja, Wajaree Weera, Stochastic Investigations for the Fractional Vector-Host Diseased Based Saturated Function of Treatment Model, 2023, 74, 1546-2226, 559, 10.32604/cmc.2023.031871 | |
24. | Zulqurnain Sabir, Neuron analysis through the swarming procedures for the singular two-point boundary value problems arising in the theory of thermal explosion, 2022, 137, 2190-5444, 10.1140/epjp/s13360-022-02869-3 | |
25. | Muhammad Umar, Fazli Amin, Soheil Salahshour, Thongchai Botmart, Wajaree Weera, Prem Junswang, Zulqurnain Sabir, Swarming Computational Approach for the Heartbeat Van Der Pol Nonlinear System, 2022, 72, 1546-2226, 6185, 10.32604/cmc.2022.027970 | |
26. | Thongchai Botmart, Zulqurnain Sabir, Afaf S. Alwabli, Salem Ben Said, Qasem Al-Mdallal, Maria Emilia Camargo, Wajaree Weera, Computational Stochastic Investigations for the Socio-Ecological Dynamics with Reef Ecosystems, 2022, 73, 1546-2226, 5589, 10.32604/cmc.2022.032087 | |
27. | Qusain Haider, Ali Hassan, Sayed M. Eldin, Artificial neural network scheme to solve the hepatitis B virus model, 2023, 9, 2297-4687, 10.3389/fams.2023.1072447 | |
28. | Farhad Muhammad Riaz, Shafiq Ahmad, Juniad Ali Khan, Saud Altaf, Zia Ur Rehman, Shuaib Karim Memon, Numerical Treatment of Non-Linear System for Latently Infected CD4+T Cells: A Swarm- Optimized Neural Network Approach, 2024, 12, 2169-3536, 103119, 10.1109/ACCESS.2024.3429279 | |
29. | Zulqurnain Sabir, Shahid Ahmad Bhat, Muhammad Asif Zahoor Raja, Sharifah E. Alhazmi, A swarming neural network computing approach to solve the Zika virus model, 2023, 126, 09521976, 106924, 10.1016/j.engappai.2023.106924 | |
30. | Chenxu Wang, Mengqin Wang, Xiaoguang Wang, Luyue Zhang, Yi Long, Multi-Relational Graph Representation Learning for Financial Statement Fraud Detection, 2024, 7, 2096-0654, 920, 10.26599/BDMA.2024.9020013 | |
31. | ZULQURNAIN SABIR, DUMITRU BALEANU, MUHAMMAD ASIF ZAHOOR RAJA, ALI S. ALSHOMRANI, EVREN HINCAL, COMPUTATIONAL PERFORMANCES OF MORLET WAVELET NEURAL NETWORK FOR SOLVING A NONLINEAR DYNAMIC BASED ON THE MATHEMATICAL MODEL OF THE AFFECTION OF LAYLA AND MAJNUN, 2023, 31, 0218-348X, 10.1142/S0218348X23400169 | |
32. | Zulqurnain Sabir, Mohamed R. Ali, R. Sadat, Simulation of fractional order mathematical model of robots for detection of coronavirus using Levenberg–Marquardt backpropagation neural network, 2024, 0941-0643, 10.1007/s00521-024-10361-5 | |
33. | MUHAMMAD SHOAIB, RAFIA TABASSUM, KOTTAKKARAN SOOPPY NISAR, MUHAMMAD ASIF ZAHOOR RAJA, FAROOQ AHMED SHAH, MOHAMMED S. ALQAHTANI, C. AHAMED SALEEL, H. M. ALMOHIY, DESIGN OF BIO-INSPIRED HEURISTIC TECHNIQUE INTEGRATED WITH SEQUENTIAL QUADRATIC PROGRAMMING FOR NONLINEAR MODEL OF PINE WILT DISEASE, 2023, 31, 0218-348X, 10.1142/S0218348X23401485 | |
34. | Manal Alqhtani, J.F. Gómez-Aguilar, Khaled M. Saad, Zulqurnain Sabir, Eduardo Pérez-Careta, A scale conjugate neural network learning process for the nonlinear malaria disease model, 2023, 8, 2473-6988, 21106, 10.3934/math.20231075 | |
35. | Soumen Pal, Manojit Bhattacharya, Snehasish Dash, Sang-Soo Lee, Chiranjib Chakraborty, A next-generation dynamic programming language Julia: Its features and applications in biological science, 2024, 64, 20901232, 143, 10.1016/j.jare.2023.11.015 | |
36. | Syed T. R. Rizvi, Aly R. Seadawy, Samia Ahmed, Homoclinic breather, periodic wave, lump solution, and M-shaped rational solutions for cold bosonic atoms in a zig-zag optical lattice, 2023, 12, 2192-8029, 10.1515/nleng-2022-0337 | |
37. | Juan Luis García Guirao, On the stochastic observation for the nonlinear system of the emigration and migration effects via artificial neural networks, 2023, 1, 2956-7068, 177, 10.2478/ijmce-2023-0014 | |
38. | Honghua Liu, Chang She, Zhiliang Huang, Lei Wei, Qian Li, Han Peng, Mailan Liu, Uncertainty analysis and optimization of laser thermal pain treatment, 2023, 13, 2045-2322, 10.1038/s41598-023-38672-y | |
39. | Zulqurnain Sabir, Salem Ben Said, Qasem Al-Mdallal, An artificial neural network approach for the language learning model, 2023, 13, 2045-2322, 10.1038/s41598-023-50219-9 | |
40. | Nabeela Anwar, Iftikhar Ahmad, Adiqa Kausar Kiani, Muhammad Shoaib, Muhammad Asif Zahoor Raja, Intelligent solution predictive networks for non-linear tumor-immune delayed model, 2024, 27, 1025-5842, 1091, 10.1080/10255842.2023.2227751 | |
41. | Manoj Gupta, Achyuth Sarkar, Stochastic intelligent computing solvers for the SIR dynamical prototype epidemic model using the impacts of the hospital bed, 2024, 1025-5842, 1, 10.1080/10255842.2023.2300684 | |
42. | Zulqurnain Sabir, Atef Hashem, Adnène Arbi, Mohamed Abdelkawy, Designing a Bayesian Regularization Approach to Solve the Fractional Layla and Majnun System, 2023, 11, 2227-7390, 3792, 10.3390/math11173792 | |
43. | Juan Luis García Guirao, On the stochastic observation for the nonlinear system of the emigration and migration effects via artificial neural networks, 2023, 0, 2956-7068, 10.2478/ijmce-2023-00014 | |
44. | A. A. Alderremy, J. F. Gómez-Aguilar, Zulqurnain Sabir, Muhammad Asif Zahoor Raja, Shaban Aly, Numerical investigations of the fractional order derivative-based accelerating universe in the modified gravity, 2024, 39, 0217-7323, 10.1142/S0217732323501808 | |
45. | A. A. Alderremy, J. F. Gómez-Aguilar, Zulqurnain Sabir, Shaban Aly, J. E. Lavín-Delgado, José R. Razo-Hernández, Numerical performances based artificial neural networks to deal with the computer viruses spread on the complex networks, 2024, 101, 0020-7160, 314, 10.1080/00207160.2024.2326926 | |
46. | Abdulgafor Alfares, Yusuf Abubakar Sha'aban, Ahmed Alhumoud, Machine learning -driven predictions of lattice constants in ABX3 Perovskite Materials, 2025, 141, 09521976, 109747, 10.1016/j.engappai.2024.109747 | |
47. | Kamel Guedri, Rahat Zarin, Mowffaq Oreijah, Samaher Khalaf Alharbi, Hamiden Abd El-Wahed Khalifa, Artificial neural network-driven modeling of Ebola transmission dynamics with delays and disability outcomes, 2025, 115, 14769271, 108350, 10.1016/j.compbiolchem.2025.108350 | |
48. | Farhad Muhammad Riaz, Junaid Ali Khan, A Swarm Optimized ANN-based Numerical Treatment of Nonlinear SEIR System based on Zika Virus, 2025, 2147-9429, 1, 10.2339/politeknik.1543179 | |
49. | Kamel Guedri, Rahat Zarin, Mowffaq Oreijah, Samaher Khalaf Alharbi, Hamiden Abd El-Wahed Khalifa, Modeling syphilis progression and disability risk with neural networks, 2025, 314, 09507051, 113220, 10.1016/j.knosys.2025.113220 | |
50. | Zulqurnain Sabir, Basma Souayeh, Zahraa Zaiour, Alyn Nazal, Mir Waqas Alam, Huda Alfannakh, A machine learning neural network architecture for the accelerating universe based modified gravity, 2025, 29, 11108665, 100635, 10.1016/j.eij.2025.100635 | |
51. | Zhou Yang, Zhi Li, Xikun Jin, Yimin Zhang, Sensitivity analysis of subjective and objective reliability of disc brakes based on the parallel efficient global optimization algorithm, 2025, 0305-215X, 1, 10.1080/0305215X.2025.2475000 |
GA process starts Inputs: The chromosomes of the same network elements are denoted as: W = [k, w, h] Population: Chromosomes set is represented as: W=[WP,WS,WQT,WQS], WP=[kP,ωP,hP], WS=[kS,ωS,hS], WQT=[kQT,ωQT,hQT] and WQS=[kQS,ωQS,hQS], W is the weight vector Outputs: The best global weight values are signified as: WGA-Best Initialization: For the chromosome's assortment, adjust the W Assessment of FIT: Adjust "ΞFit" in the population (P) for each vector values using Eqs (4)–(9). ● Stopping standards: Terminate if any of the criteria is achieved [ΞFit = 10-19], [Generataions = 120] [TolCon = TolFun = 10-18], [StallLimit = 150], & [Size of population = 270]. Move to [storage] Ranking: Rank precise W in the particular population for ΞFit. Storage: Save ΞFit, iterations, WGA-Best, function counts and time. GA procedure End |
Start of IPA Inputs: WGA-Best is selected as an initial point. Output: WGA-IPA shows the best weights of GA-IPA. Initialize: WGA-Best, generations, assignments and other standards. Terminating criteria: Stop if [ΞFit = 10-20], [TolFun = 10-18], [Iterations = 700], [TolCon = TolX = 10-22] and [MaxFunEvals = 260000] attained. Evaluation of Fit: Compute the values of W and E for Eqs (4)–(9). Amendments: Normalize 'fmincon' for IPA, compute ΞFit for Eqs (4)–(9). Accumulate: Transmute WGA-IPA, time, iterations, function counts and ΞFit for the IPA trials. |
IPA process End |
Ω | P(Ω) | |||||
Max | Min | Median | STD | SIR | Mean | |
0 | 1.9698E-03 | 1.5128E-07 | 1.3179E-05 | 4.3988E-04 | 2.8322E-05 | 1.5255E-04 |
0.1 | 2.2927E-03 | 4.0452E-05 | 3.7091E-04 | 7.5870E-04 | 3.5757E-04 | 7.1580E-04 |
0.2 | 2.8517E-04 | 2.5715E-06 | 8.0076E-05 | 1.0291E-04 | 8.7298E-05 | 1.0480E-04 |
0.3 | 3.7540E-04 | 5.0221E-06 | 4.6631E-05 | 9.6615E-05 | 5.7759E-05 | 8.0291E-05 |
0.4 | 3.1095E-04 | 7.2215E-07 | 2.5169E-05 | 7.9915E-05 | 4.8322E-05 | 6.4497E-05 |
0.5 | 3.7039E-04 | 1.0187E-07 | 4.0958E-05 | 9.9187E-05 | 4.0049E-05 | 7.4737E-05 |
0.6 | 3.4788E-04 | 2.7497E-06 | 3.6783E-05 | 8.6814E-05 | 5.3154E-05 | 7.3405E-05 |
0.7 | 7.2524E-04 | 3.8591E-06 | 2.6741E-05 | 1.6887E-04 | 5.3778E-05 | 9.7130E-05 |
0.8 | 3.1439E-04 | 2.3193E-06 | 3.0315E-05 | 8.9765E-05 | 3.8282E-05 | 6.8701E-05 |
0.9 | 8.2385E-04 | 3.6579E-06 | 3.6581E-05 | 1.8035E-04 | 2.2583E-05 | 8.9806E-05 |
1 | 3.9210E-04 | 8.9543E-07 | 1.4825E-05 | 1.1497E-04 | 3.4368E-05 | 6.7763E-05 |
Ω | S(Ω) | |||||
Max | Min | Median | STD | SIR | Mean | |
0 | 2.2510E-03 | 8.8331E-07 | 1.5049E-05 | 4.3988E-04 | 6.5800E-05 | 2.4625E-04 |
0.1 | 3.5749E-03 | 1.2621E-05 | 4.2711E-04 | 7.5870E-04 | 5.9138E-04 | 9.2693E-04 |
0.2 | 5.8967E-04 | 6.8508E-06 | 5.0534E-05 | 1.0291E-04 | 3.8159E-05 | 1.0389E-04 |
0.3 | 3.9412E-04 | 4.5897E-07 | 5.5860E-05 | 9.6615E-05 | 4.8082E-05 | 9.7091E-05 |
0.4 | 1.8148E-04 | 1.4261E-06 | 3.2627E-05 | 7.9915E-05 | 4.6977E-05 | 6.1366E-05 |
0.5 | 3.8406E-04 | 3.0499E-06 | 5.0425E-05 | 9.9187E-05 | 4.5676E-05 | 9.6411E-05 |
0.6 | 3.3693E-04 | 1.0786E-06 | 3.2525E-05 | 8.6814E-05 | 2.6474E-05 | 5.9807E-05 |
0.7 | 3.8976E-04 | 5.9658E-08 | 3.4605E-05 | 1.6887E-04 | 3.0731E-05 | 7.1943E-05 |
0.8 | 4.6451E-04 | 1.8083E-07 | 2.9100E-05 | 8.9765E-05 | 1.9639E-05 | 5.1752E-05 |
0.9 | 3.7056E-04 | 5.8454E-07 | 3.1691E-05 | 1.8035E-04 | 4.5808E-05 | 7.8891E-05 |
1 | 3.4425E-04 | 3.5833E-08 | 3.7245E-05 | 1.1497E-04 | 2.5924E-05 | 6.3636E-05 |
Ω | QT(Ω) | |||||
Max | Min | Median | STD | SIR | Mean | |
0 | 6.7379E-02 | 2.0435E-06 | 7.4367E-05 | 4.3988E-04 | 1.2547E-03 | 6.6320E-03 |
0.1 | 8.8212E-03 | 1.5933E-04 | 6.6896E-04 | 7.5870E-04 | 6.4736E-04 | 1.4858E-03 |
0.2 | 1.2208E-03 | 1.0177E-06 | 1.6681E-04 | 1.0291E-04 | 1.3929E-04 | 2.7755E-04 |
0.3 | 1.2039E-03 | 6.2163E-06 | 3.5966E-05 | 9.6615E-05 | 7.5659E-05 | 2.0075E-04 |
0.4 | 5.2048E-04 | 1.5004E-06 | 9.3199E-05 | 7.9915E-05 | 1.4812E-04 | 1.7703E-04 |
0.5 | 7.9163E-04 | 4.1214E-06 | 7.5760E-05 | 9.9187E-05 | 9.4190E-05 | 1.7072E-04 |
0.6 | 7.5113E-04 | 3.4495E-06 | 5.6836E-05 | 8.6814E-05 | 1.5865E-04 | 1.8051E-04 |
0.7 | 6.9564E-04 | 2.1601E-06 | 8.7570E-05 | 1.6887E-04 | 1.2891E-04 | 1.6565E-04 |
0.8 | 8.5659E-04 | 2.3356E-06 | 5.6424E-05 | 8.9765E-05 | 6.5358E-05 | 1.2238E-04 |
0.9 | 8.5832E-04 | 1.8869E-06 | 8.6636E-05 | 1.8035E-04 | 1.2103E-04 | 1.8340E-04 |
1 | 5.9922E-04 | 9.8962E-07 | 3.9188E-05 | 1.1497E-04 | 4.8965E-05 | 1.1550E-04 |
Ω | QS(Ω) | |||||
Max | Min | Median | STD | SIR | Mean | |
0 | 1.4249E-02 | 4.6038E-07 | 1.0193E-05 | 3.1752E-03 | 5.3007E-05 | 7.6325E-04 |
0.1 | 7.1456E-03 | 9.4140E-06 | 2.0718E-04 | 1.6018E-03 | 3.9882E-04 | 8.1152E-04 |
0.2 | 1.4449E-03 | 2.1615E-07 | 2.8536E-05 | 3.6835E-04 | 3.0447E-05 | 1.7480E-04 |
0.3 | 3.7091E-04 | 3.2754E-07 | 2.1791E-05 | 8.7324E-05 | 2.6186E-05 | 5.6609E-05 |
0.4 | 1.5545E-03 | 1.8846E-07 | 2.2389E-05 | 3.7612E-04 | 6.1143E-05 | 1.5810E-04 |
0.5 | 3.7972E-04 | 3.6651E-06 | 1.7656E-05 | 9.8654E-05 | 3.1268E-05 | 6.2521E-05 |
0.6 | 1.2224E-03 | 2.4032E-07 | 2.1050E-05 | 2.9478E-04 | 3.3053E-05 | 1.2732E-04 |
0.7 | 7.4101E-04 | 1.7539E-07 | 2.4276E-05 | 1.6625E-04 | 2.5398E-05 | 7.7130E-05 |
0.8 | 1.0726E-03 | 2.5213E-06 | 1.4100E-05 | 2.3771E-04 | 3.0541E-05 | 9.1329E-05 |
0.9 | 7.4407E-04 | 2.5770E-06 | 3.5282E-05 | 1.6258E-04 | 2.8865E-05 | 7.9482E-05 |
1 | 9.7616E-04 | 7.0693E-07 | 8.9328E-06 | 2.1858E-04 | 1.0034E-05 | 8.0351E-05 |
Class | (G.MAD) | (G.TIC) | (G.EVAF) | |||
Min | SIR | Min | SIR | Min | SIR | |
P(Ω) | 1.2499E-04 | 9.6058E-05 | 7.2597E-09 | 7.4459E-09 | 2.6155E-07 | 7.5331E-07 |
S(Ω) | 9.7570E-05 | 1.3353E-04 | 8.3068E-08 | 1.0766E-08 | 1.3879E-06 | 5.2293E-06 |
QT(Ω) | 1.4111E-04 | 2.5334E-04 | 9.1566E-09 | 1.8187E-08 | 3.9553E-05 | 5.1487E-04 |
QS(Ω) | 6.0979E-05 | 4.1189E-05 | 3.6367E-09 | 4.8515E-09 | 1.1015E-06 | 9.3296E-06 |
GA process starts Inputs: The chromosomes of the same network elements are denoted as: W = [k, w, h] Population: Chromosomes set is represented as: W=[WP,WS,WQT,WQS], WP=[kP,ωP,hP], WS=[kS,ωS,hS], WQT=[kQT,ωQT,hQT] and WQS=[kQS,ωQS,hQS], W is the weight vector Outputs: The best global weight values are signified as: WGA-Best Initialization: For the chromosome's assortment, adjust the W Assessment of FIT: Adjust "ΞFit" in the population (P) for each vector values using Eqs (4)–(9). ● Stopping standards: Terminate if any of the criteria is achieved [ΞFit = 10-19], [Generataions = 120] [TolCon = TolFun = 10-18], [StallLimit = 150], & [Size of population = 270]. Move to [storage] Ranking: Rank precise W in the particular population for ΞFit. Storage: Save ΞFit, iterations, WGA-Best, function counts and time. GA procedure End |
Start of IPA Inputs: WGA-Best is selected as an initial point. Output: WGA-IPA shows the best weights of GA-IPA. Initialize: WGA-Best, generations, assignments and other standards. Terminating criteria: Stop if [ΞFit = 10-20], [TolFun = 10-18], [Iterations = 700], [TolCon = TolX = 10-22] and [MaxFunEvals = 260000] attained. Evaluation of Fit: Compute the values of W and E for Eqs (4)–(9). Amendments: Normalize 'fmincon' for IPA, compute ΞFit for Eqs (4)–(9). Accumulate: Transmute WGA-IPA, time, iterations, function counts and ΞFit for the IPA trials. |
IPA process End |
Ω | P(Ω) | |||||
Max | Min | Median | STD | SIR | Mean | |
0 | 1.9698E-03 | 1.5128E-07 | 1.3179E-05 | 4.3988E-04 | 2.8322E-05 | 1.5255E-04 |
0.1 | 2.2927E-03 | 4.0452E-05 | 3.7091E-04 | 7.5870E-04 | 3.5757E-04 | 7.1580E-04 |
0.2 | 2.8517E-04 | 2.5715E-06 | 8.0076E-05 | 1.0291E-04 | 8.7298E-05 | 1.0480E-04 |
0.3 | 3.7540E-04 | 5.0221E-06 | 4.6631E-05 | 9.6615E-05 | 5.7759E-05 | 8.0291E-05 |
0.4 | 3.1095E-04 | 7.2215E-07 | 2.5169E-05 | 7.9915E-05 | 4.8322E-05 | 6.4497E-05 |
0.5 | 3.7039E-04 | 1.0187E-07 | 4.0958E-05 | 9.9187E-05 | 4.0049E-05 | 7.4737E-05 |
0.6 | 3.4788E-04 | 2.7497E-06 | 3.6783E-05 | 8.6814E-05 | 5.3154E-05 | 7.3405E-05 |
0.7 | 7.2524E-04 | 3.8591E-06 | 2.6741E-05 | 1.6887E-04 | 5.3778E-05 | 9.7130E-05 |
0.8 | 3.1439E-04 | 2.3193E-06 | 3.0315E-05 | 8.9765E-05 | 3.8282E-05 | 6.8701E-05 |
0.9 | 8.2385E-04 | 3.6579E-06 | 3.6581E-05 | 1.8035E-04 | 2.2583E-05 | 8.9806E-05 |
1 | 3.9210E-04 | 8.9543E-07 | 1.4825E-05 | 1.1497E-04 | 3.4368E-05 | 6.7763E-05 |
Ω | S(Ω) | |||||
Max | Min | Median | STD | SIR | Mean | |
0 | 2.2510E-03 | 8.8331E-07 | 1.5049E-05 | 4.3988E-04 | 6.5800E-05 | 2.4625E-04 |
0.1 | 3.5749E-03 | 1.2621E-05 | 4.2711E-04 | 7.5870E-04 | 5.9138E-04 | 9.2693E-04 |
0.2 | 5.8967E-04 | 6.8508E-06 | 5.0534E-05 | 1.0291E-04 | 3.8159E-05 | 1.0389E-04 |
0.3 | 3.9412E-04 | 4.5897E-07 | 5.5860E-05 | 9.6615E-05 | 4.8082E-05 | 9.7091E-05 |
0.4 | 1.8148E-04 | 1.4261E-06 | 3.2627E-05 | 7.9915E-05 | 4.6977E-05 | 6.1366E-05 |
0.5 | 3.8406E-04 | 3.0499E-06 | 5.0425E-05 | 9.9187E-05 | 4.5676E-05 | 9.6411E-05 |
0.6 | 3.3693E-04 | 1.0786E-06 | 3.2525E-05 | 8.6814E-05 | 2.6474E-05 | 5.9807E-05 |
0.7 | 3.8976E-04 | 5.9658E-08 | 3.4605E-05 | 1.6887E-04 | 3.0731E-05 | 7.1943E-05 |
0.8 | 4.6451E-04 | 1.8083E-07 | 2.9100E-05 | 8.9765E-05 | 1.9639E-05 | 5.1752E-05 |
0.9 | 3.7056E-04 | 5.8454E-07 | 3.1691E-05 | 1.8035E-04 | 4.5808E-05 | 7.8891E-05 |
1 | 3.4425E-04 | 3.5833E-08 | 3.7245E-05 | 1.1497E-04 | 2.5924E-05 | 6.3636E-05 |
Ω | QT(Ω) | |||||
Max | Min | Median | STD | SIR | Mean | |
0 | 6.7379E-02 | 2.0435E-06 | 7.4367E-05 | 4.3988E-04 | 1.2547E-03 | 6.6320E-03 |
0.1 | 8.8212E-03 | 1.5933E-04 | 6.6896E-04 | 7.5870E-04 | 6.4736E-04 | 1.4858E-03 |
0.2 | 1.2208E-03 | 1.0177E-06 | 1.6681E-04 | 1.0291E-04 | 1.3929E-04 | 2.7755E-04 |
0.3 | 1.2039E-03 | 6.2163E-06 | 3.5966E-05 | 9.6615E-05 | 7.5659E-05 | 2.0075E-04 |
0.4 | 5.2048E-04 | 1.5004E-06 | 9.3199E-05 | 7.9915E-05 | 1.4812E-04 | 1.7703E-04 |
0.5 | 7.9163E-04 | 4.1214E-06 | 7.5760E-05 | 9.9187E-05 | 9.4190E-05 | 1.7072E-04 |
0.6 | 7.5113E-04 | 3.4495E-06 | 5.6836E-05 | 8.6814E-05 | 1.5865E-04 | 1.8051E-04 |
0.7 | 6.9564E-04 | 2.1601E-06 | 8.7570E-05 | 1.6887E-04 | 1.2891E-04 | 1.6565E-04 |
0.8 | 8.5659E-04 | 2.3356E-06 | 5.6424E-05 | 8.9765E-05 | 6.5358E-05 | 1.2238E-04 |
0.9 | 8.5832E-04 | 1.8869E-06 | 8.6636E-05 | 1.8035E-04 | 1.2103E-04 | 1.8340E-04 |
1 | 5.9922E-04 | 9.8962E-07 | 3.9188E-05 | 1.1497E-04 | 4.8965E-05 | 1.1550E-04 |
Ω | QS(Ω) | |||||
Max | Min | Median | STD | SIR | Mean | |
0 | 1.4249E-02 | 4.6038E-07 | 1.0193E-05 | 3.1752E-03 | 5.3007E-05 | 7.6325E-04 |
0.1 | 7.1456E-03 | 9.4140E-06 | 2.0718E-04 | 1.6018E-03 | 3.9882E-04 | 8.1152E-04 |
0.2 | 1.4449E-03 | 2.1615E-07 | 2.8536E-05 | 3.6835E-04 | 3.0447E-05 | 1.7480E-04 |
0.3 | 3.7091E-04 | 3.2754E-07 | 2.1791E-05 | 8.7324E-05 | 2.6186E-05 | 5.6609E-05 |
0.4 | 1.5545E-03 | 1.8846E-07 | 2.2389E-05 | 3.7612E-04 | 6.1143E-05 | 1.5810E-04 |
0.5 | 3.7972E-04 | 3.6651E-06 | 1.7656E-05 | 9.8654E-05 | 3.1268E-05 | 6.2521E-05 |
0.6 | 1.2224E-03 | 2.4032E-07 | 2.1050E-05 | 2.9478E-04 | 3.3053E-05 | 1.2732E-04 |
0.7 | 7.4101E-04 | 1.7539E-07 | 2.4276E-05 | 1.6625E-04 | 2.5398E-05 | 7.7130E-05 |
0.8 | 1.0726E-03 | 2.5213E-06 | 1.4100E-05 | 2.3771E-04 | 3.0541E-05 | 9.1329E-05 |
0.9 | 7.4407E-04 | 2.5770E-06 | 3.5282E-05 | 1.6258E-04 | 2.8865E-05 | 7.9482E-05 |
1 | 9.7616E-04 | 7.0693E-07 | 8.9328E-06 | 2.1858E-04 | 1.0034E-05 | 8.0351E-05 |
Class | (G.MAD) | (G.TIC) | (G.EVAF) | |||
Min | SIR | Min | SIR | Min | SIR | |
P(Ω) | 1.2499E-04 | 9.6058E-05 | 7.2597E-09 | 7.4459E-09 | 2.6155E-07 | 7.5331E-07 |
S(Ω) | 9.7570E-05 | 1.3353E-04 | 8.3068E-08 | 1.0766E-08 | 1.3879E-06 | 5.2293E-06 |
QT(Ω) | 1.4111E-04 | 2.5334E-04 | 9.1566E-09 | 1.8187E-08 | 3.9553E-05 | 5.1487E-04 |
QS(Ω) | 6.0979E-05 | 4.1189E-05 | 3.6367E-09 | 4.8515E-09 | 1.1015E-06 | 9.3296E-06 |