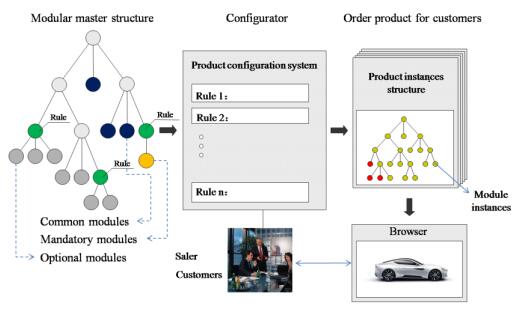
Citation: Arqum Shahid, Ahsan Ali, Faheem Ashiq, Umer Amir Khan, Muhammad Ilyas Menhas, Sajjad Manzoor. Comparison of resistive and inductive superconductor fault current limiters in AC and DC micro-grids[J]. AIMS Energy, 2020, 8(6): 1199-1211. doi: 10.3934/energy.2020.6.1199
[1] | Tingting Yang, Yi He . Design of intelligent robots for tourism management service based on green computing. Mathematical Biosciences and Engineering, 2023, 20(3): 4798-4815. doi: 10.3934/mbe.2023222 |
[2] | Changwei Gong, Bing Xue, Changhong Jing, Chun-Hui He, Guo-Cheng Wu, Baiying Lei, Shuqiang Wang . Time-sequential graph adversarial learning for brain modularity community detection. Mathematical Biosciences and Engineering, 2022, 19(12): 13276-13293. doi: 10.3934/mbe.2022621 |
[3] | Orit Lavi, Doron Ginsberg, Yoram Louzoun . Regulation of modular Cyclin and CDK feedback loops by an E2F transcription oscillator in the mammalian cell cycle. Mathematical Biosciences and Engineering, 2011, 8(2): 445-461. doi: 10.3934/mbe.2011.8.445 |
[4] | Gheorghe Craciun, Baltazar Aguda, Avner Friedman . Mathematical Analysis Of A Modular Network Coordinating The Cell Cycle And Apoptosis. Mathematical Biosciences and Engineering, 2005, 2(3): 473-485. doi: 10.3934/mbe.2005.2.473 |
[5] | Maide Bucolo, Federica Di Grazia, Luigi Fortuna, Mattia Frasca, Francesca Sapuppo . An environment for complex behaviour detection in bio-potential experiments. Mathematical Biosciences and Engineering, 2008, 5(2): 261-276. doi: 10.3934/mbe.2008.5.261 |
[6] | Xiaodong Zhang, Dawei Ren . Modeling and simulation of task rescheduling strategy with resource substitution in cloud manufacturing. Mathematical Biosciences and Engineering, 2023, 20(2): 3120-3145. doi: 10.3934/mbe.2023147 |
[7] | Zhen Liu, Song Yang, Chen Cheng, Tao Ding, Ruimin Chai . Study on modeling and dynamic performance of a planar flexible parallel manipulator based on finite element method. Mathematical Biosciences and Engineering, 2023, 20(1): 807-836. doi: 10.3934/mbe.2023037 |
[8] | Jing Zhang, Ting Fan, Ding Lang, Yuguang Xu, Hong-an Li, Xuewen Li . Intelligent crowd sensing pickpocketing group identification using remote sensing data for secure smart cities. Mathematical Biosciences and Engineering, 2023, 20(8): 13777-13797. doi: 10.3934/mbe.2023613 |
[9] | Roman Gumzej . Intelligent logistics systems in E-commerce and transportation. Mathematical Biosciences and Engineering, 2023, 20(2): 2348-2363. doi: 10.3934/mbe.2023110 |
[10] | Zhijun Yang, Wenjie Huang, Hongwei Ding, Zheng Guan, Zongshan Wang . Performance analysis of a two-level polling control system based on LSTM and attention mechanism for wireless sensor networks. Mathematical Biosciences and Engineering, 2023, 20(11): 20155-20187. doi: 10.3934/mbe.2023893 |
Intelligent manufacturing is reforming the design methods, technical systems, and production processes of modern manufacturing industry, and is thereby driving the global manufacturing industry stepping forward a new stage of development [1,2]. Product-service system (PSS) is an important way of the transformation and upgrading of modern manufacturing industry, it is also one of core development trends of intelligent manufacturing [3]. In particular, the intelligent design of a Product-service system (PSS) is one of the core development trends of intelligent manufacturing. PSS aims to offer a leverage of products and services to jointly fulfill individual customer needs [4]. With the emergence of PSS strategy, the personalization and variability of PSS inevitably lead to the increase of the cost in stages such as management, design, manufacturing, supply and implementation for enterprises [5]. PSS contains a physical product core, which is supplemented by specific non-physical services [6]. The modularization for realizing technical PSS needs both physical (product) and non-physical (technical service) constituents [7]. Through the establishment of a series of standard physical modules and service modules, the less diversification for internal modules can be realized, which helps to reduce production costs and the impact on the environment. Through the configuration design, external personalization and variability of PSS can be achieved, which helps to meet the customer's individual requirements [8,9,10].
PSS mainly contains three types: product-oriented PSS, use-oriented PSS and result-oriented PSS [11,12]. The product-oriented PSS is a PSS where the ownership of the tangible product is transferred to the consumer, and additional basic services are provided. The use-oriented PSS is a PSS where the ownership of the tangible product is retained by the service provider who sells the functions of the product via modified distribution and payment systems. The result-oriented PSS is a PSS where products are replaced by services, which are to say, selling the service result or capability of a product. The modularization process of PSS includes two levels, namely are services solution layer and generic part layer [9,13]. In the process of PSS modular optimization configuration design, the service solution modules are configured in services solution layer firstly; in this stage, the service solution layer mainly focuses on the benefits of customer's needs, and takes the customer's maximum satisfaction as the optimization objective. Then, the generic part modules are configured based on the service solutions of services solution layer; in this stage, the modular design in generic part layer mainly focus on the manufacturer's benefits, the optimization goal is to get the maximum cost performance of PSS module and the highest profit margins for manufacturers. It is worth to note that the module configuration in service solution layer is a modular design process with coarse granularity modules (includes physical modules and/or service modules), and decisions are made by the user. Granularity refers to the size of particles; module granularity reflects the size and level of modules. Coarse granularity modules generally consist of sub-modules of lower layers (fine granularity). Compared to the services solution layer, modules in generic part layer are with fine granularity, including physical modules and/or service modules, and decisions are made by the manufacturer. Therefore, PSS modular optimization configuration design consists of two different levels, the first level is user's decision-making configuration design, and the second level is the manufacturer's configuration design. Essentially, the two levels have different decision-making subjects, and their decision-making goals are also different.
PSS modular configuration design can be achieved via PPS design platform, the PPS design platform mainly consists of a universal modular master structure, which is a tree structure [7,14]. Tree nodes are composed of different types of modules (common modules, mandatory modules, and optional modules), the relationships among nodes are constrained by configuration rules [9,13]. When a PSS is configured, according to customer's needs, different types of modules (common modules, mandatory modules, and optional modules) are selected and configured based on the product platform, and forms a series of product instances. However, before the implementation of the modular design, module partition must be finished firstly, coarse granularity modules are divided into fine granularity modules, and a modular coarse structure is built. After the module partition, the types of modules must be identified, as well as modules of customer's decision-making and its properties (services solution layer) and modules of manufacturer's decision-making and its properties (generic part layer). This is a bi-level (service solution level and generic part level) decision-making process for the module types and customization degree. Therefore, this is the basis to build the master structure and modular design platform of PSS.
For PSS providers (manufacturers), the decision-making on PSS's bi-level module types and customization degree are very important, which directly determine the PSS modular structure and also affect the degree of personalization and manufacturing costs of PSS. The bi-level module type has two levels, the modules of the first level are configured according to the user's decision-making, and the modules of the second level are configured according to the manufacturer's decision-making. The main structure of this paper is as follows. The second section summarizes the research progress on PSS modular design methods and decision-making methods of module types; the third section proposes a modular planning methodology for PSS services solution layer and generic part layer; the fourth section acquires and classifies the PSS service needs; the fifth section determines PSS module types; the sixth section determines the generalized module instance; the seventh section plans the PSS module types; the eighth section establishes the bi-level master structure of PSS; the ninth section takes transformer equipment as an example to verify the proposed methodology. The final part is discussion and summary.
The research on the modular design of PSS mainly includes: (1) design framework, architecture or product platform, (2) module partition, and (3) configuration design.
In the field of design framework, architecture or product platform. Tilo proposed how the concept of modular service architectures addressed external factors and varying service level requirements, and pointed out that modular service architecture could improve the customization of IT services [15]. Then, Aurich firstly proposed a modular design framework for PSS, he put forward a two-step method, and built up the principle of modularization for realizing technical PSS. He also proposed a process library to design and manufacture technical PSS, also to select, combine and adapt appropriate process modules [6,7,16]. Then, Geum put forward a framework for service modularization, by employing and modifying the House of Quality (HoQ) structure in Quality Function Deployment (QFD) using two ways: driver-based approach and interrelationship-based approach, a clustering analysis was conducted to identify the module candidates [17]. Wang proposed a framework of modular development for PSS. He pointed out the process of modular development were divided into three parts by order: functional modularization, product modularization, and service modularization [18].
In the field of module partition. Hara analyzed and modeled eight types of PSS from three viewpoints: The state of receivers, functions, and attributes of entities, which contributed to configuring modules of product-service combination toward a design of new PSS [19]. Tuunanen proposed the concept of service process modularization, and enabled service process to be reused and combined modular service offerings with good customer satisfaction [20]. In order to clarify the relationships between the physical module and service module, and identify the interactive design process, Li proposed a three-phase module partition method for integrated service product, the services can be classified into functional and non-functional services, the interaction relationships between physical modules and service modules were analyzed [8,9,14]. Sun proposed a fuzzy clustering algorithm to identify functional requirements of PSS, and classify the modules into different clusters [21]. Aiming at the PSS redesigning, Chen proposed an approach of identifying PSS redesign modules based on user experience, the approach includes three steps: Obtain the relationships between user experience and experience dimensions, build the relationships between experience dimensions and modules, and acquire the experience reduction risk model [22]. Zhang divided PSS into multiple-modules associated with function characteristics, and evaluated it by using the outputs of parallel houses of quality [23].
In the field of configuration design. To improve the sharing and reusability of configuration model, Dong suggested a modular modeling and configuration method based on ontology technology. He used the reachability matrix to finish the service module division, and proposed the ontology technology and Java expert system shell inference engine to generate configuration solution [24]. Li proposed a bi-level coordinated optimization framework to support PSS configuration design. An upper-level optimization problem was formulated for service configuration to act as a leader to achieve customer satisfaction, whilst subject to a lower-level optimization problem for product configuration to perform as a follower to enhance the sales profit [10]. Fadeyi developed an optimization model that identified the module variants that should be included in product configuration at an early phase of PSS development [25].
PSS modular configuration design can be achieved via PPS design platform [7]. However, there are still several key problems in building PPS modular design platform, such as the PSS module types is still needed to be classified, and decision-making on modules and its properties need to be finished, the solving of which are the basis for building the PPS modular structure and design platform.
On the decision-making of module types, Chang proposed a clustering model based on the gray syst em, and got the basic function modules and auxiliary function modules from the analysis of customer needs [26]. Li finished the demand classification based on the fuzzy Kano model, and established a product function model with the expression of material flow, energy flow and signal flow [8].
In the field of customization degree analysis, several frameworks that described different customization degree were proposed in some literature [27,28,29]. However, these studies did not provide quantitative calculation methods for the mass customization degree. One of views in these studies was that: The module customization level needed to be determined from the perspective of customer's demands. Stone described an approach for identifying modules for product architectures, the functional basis and time ordered function chains were used to derive functional models of products. Three heuristic methods for identifying modules from functional models were presented [30]. The demand model for customized products, standard products and total products as well as the model for manufacturer's profit were established by Zhou, according to the models, the influence of customization degree and price gap between customized product and standard products on market demand were analyzed [31]. On decision making about module property choice, Yi used conjoint analysis model to quantify the utility of feature level of qualitative and quantitative components, and determined product customization degree and the ownership of configuration [32]. Then, Xu established a measurement model of customer preference based on utility, proposed a product customization degree model based on attribute important degree, and built a regression model between the customization degree and customer satisfaction [33]. The above proposed methods provided a strong support to module types and module attribute decision making of physical products.
The above research overviews provide us with some information: In order to build the PPS modular structure and design platform, after the PSS module partition, two levels of module class need to be established, which is the service solution layer and generic part layer. The two aspects need further research: (1) identify the module types (common module, mandatory module, and optional module) for different types of PSS. (2) Find out the customer's decision-making modules and their properties as well as the manufacturer's decision-making modules and their properties for different types of PSS.
The configuration process of PSS modular design is given in Figure 1; it mainly consists of three steps: Modular master structure, configurator, and customized product. The modular master structure includes common module, mandatory modules, optional modules, and rules. The configurator is a product configuration system; it can configure a customized product according to customer's personalized requirements. Customized product consists of product instance structure and module instances [14].
In the modular master structure tree, a module may have several variants, each variation module is an instance module, and all the variation modules belong to the same module class. Based on the rule to select instance module in the module class, and forms a product instance structure, this is the process of modular configuration design.
In a modular master structure tree, there are three types of modules. The first is common module, common modules are those which need not be selected or customized according to different requirements, but are necessary default modules in terms of ensuring normal operation and use for a specific PSS. The second is mandatory module, mandatory modules are those which must be selected by customers, but their application should be customized according to different customer requirements. They are necessary, but not default, modules used for ensuring the normal operation and use of PSS. The third is optional module, optional modules are those which can be selected according to customer's personalized requirements; they represent additional products or service modules within a PSS [10,14].
As shown in Figure 2, the modular planning method for PSS services solution layer and generic part layer consists of five stages, respectively are collect service needs, identify generalized module types, find feasible solution for each module, make decisions on module layers, and establish modular coarse and master structure. Each phase has its motivations, and can be carried out by several methods; the specific steps are as follows.
Step 1: Collect service needs. In order to obtain and classify service needs, several methods should be applied to obtain customer's service needs from different levels and groups. Firstly, quantitative customer's service needs information usually can be obtained through questionnaires [34]. Then, Kano model can be used to understand and classify customer needs, customer's service needs will be divided into basic needs, performance needs and excitement needs, which provides support for product planning of different types of PSS [35].
Step 2: Identify generalized module types. For the same module in different types of PSS (product-oriented PSS, use-oriented PSS, and result-oriented PSS), the module types (common module, mandatory module, and optional module) may be different. In order to identify generalized module types in different types of PSS. In this paper, based on the Kano model, six different kinds of needs will be calculated, and then gets the total evaluation value of each module class, according to which to determine the types of module.
Step 3: Find the feasible solution. For each module class, it has several module solutions, and the principle solution should be found, and forms a set that contains several principle solutions. Solution set can be managed by the morphological box method [36].
Step 4: Make decisions on module layers. In order to evaluate modules should belong to services solution layer or generic part layer, conjoint analysis method is applied. The module class and instances of three types of PSS portfolio are evaluated, and gets the indicators of utility value and importance degree. Finally, customer's decision-making modules and manufacturer's decision-making modules are evaluated according to the indicators.
Step 5: Establish the modular coarse and master structure for PSS. Establish PSS coarse structure according to the optimizing result of PSS service solution modules, and build the PSS modular master structure according to the result of the module planning for services solution layer and generic part layer.
Service requirement information includes three parts: Service requirement description, the importance degree of the service requirement and customer satisfaction. Among them, the service requirement description is qualitative requirement information, and the others are quantitative requirement information [34,37,38]. The collection of PSS service requirements consists of four steps: determine respondent, determine survey methods, carry out market research, and finish customer needs. All the service requirement information from the survey can be analyzed and classified by using the Kano model.
Assume that the PSS has been divided into physical and service modules. Customer needs can be divided into basic needs, performance needs and excitement needs [35]. Customer needs are collected and classified for each module of PSS, Tables 1, 2 can be used to set up questions and evaluate it, which is based on the Kano model. In Table 1, the positive questions and negative questions are set up, and the answer set are also given. In Table 2, the interaction matrix of forward and negative questions is built. The interaction results can be comprehensive expressed as three types: Basic needs (B), performance needs (A), and excitement needs (E). And the other results are as follows: Neutral results (I), opposite results (P), and confusion results (D).
Questions | Answers |
Positive questions: If you can customize the ** module of PSS, how do you feel about it? | Very satisfied, satisfied, neutral, acceptable, not satisfied |
Negative questions: If you cannot customize the ** module of PSS, how do you feel about it? |
Decision-making needs of modules and attributes | Negative questions | |||||
Very satisfied | Satisfied | Neutral | Acceptable | Not satisfied | ||
Positive questions | Very satisfied | D | E | E | E | A |
Satisfied | P | I | I | I | B | |
Neutral | P | I | I | I | B | |
Acceptable | P | I | I | I | B | |
Not satisfied | P | P | P | P | D |
For the same module in different types of PSS, the module types (common module, mandatory module, and optional module) may be different. For example, for the lock module of bikes, when renting a bike by public self-service, it is common module and cannot be chosen. But it is optional module when the bike is used for sales. So when identifying the types of PSS modules, the scoring should be finished separately for product-oriented PSS, use-oriented PSS and result-oriented PSS.
In order to realize the quantitative calculation of module types, according to the principle of Kano evaluation table (see Table 2), the survey results of module needs for three types of PSS can be set. The values in Table 2 are expressed as follows: basic needs (B), performance needs (A), excitement needs (E), neutral results (I), opposite results (P) and confusion results (D) are expressed respectively C(B), C(A), C(E), C(I), C(P) and C(D).
If the PSS module collection is represented as
PD=n∑i=1(C(B)i⋅mi1+C(A)i⋅mi2+C(E)i⋅mi3+C(I)i⋅mi4+C(P)i⋅mi5+C(D)i⋅mi6) | (1) |
For three different types of PSS, calculate the value of PD in each module respectively, and build the columnar figure of the values of PD. According to the analysis of the practical situation, set the range of PD value to identify module types. Generally, modules in the lower section of the PD value are common modules; modules in the higher section of the PD value are optional modules.
For the module class that its category has been determined, the module instance (feasible solution) must be found as much as possible to plan module configuration and attribute values. Many module instances only have the problems of structure design, so it is not necessary to look for new physical effect. Some tasks can be solved by one service business, but some tasks are carried out by several physical products, or the integration of physical products and services. So for the principles that we are looking for, it contains both the geometry and material characteristics of physical products and some service business [36]. Establish the module instances (solution domain) through the variations of physical effects, geometric and material characteristics and service characteristics. In order to achieve a function of one module class, several physical or service effects may play on one or more function carriers. The methods that we use to build the module instances are as follows: Literature searching method, analyzing natural systems, analyzing existing technology systems, analogy method, brain storming method, association method, etc. The module instances are stored in a two-dimensional table, which consists of rows and columns, the rows and columns are used to fill in the summarized parameters. The two-dimensional table is used as directory solution in all phases, and the solutions are stored by the type and complexity [36].
The more module classes and module instances that can be configured by customers, the higher the degree of customization. In view of customers, they expect more module instances to meet more personalized needs; this is a decision-making on the services solution layer which mainly focuses on the benefits of customers. However, in view of enterprise manufacturing costs, the increase of module instances will cause the increase of category of configurable product, which will necessarily bring the increase of product cost and service complexity sharply. For the manufacturers, if they want to configure more types of module instances, they need to provide higher-profit modules to the customer to maximize the benefits; this is a decision-making on the generic part layer. Therefore, it is an important issue to make reasonably module decision on services solution layer and generic part layer.
In the stage of the PSS module levels planning, the customer preferences of the PSS modules should be considered, only providing the products and services that customers like, customers can have a desire to purchase. PSS mainly consists of three types: Product-oriented PSS, use-oriented PSS and result-oriented PSS [11,12]. For the types of products-provide are different, and the decision-making levels are different, so the results of the module planning of the three types of PSS are also different. Therefore, the three different types of PSS should be planned respectively, which helps to build the reasonable modular structure for each type of PSS.
Customer preferences can be realized by conjoint analysis method [39]. Conjoint analysis is widely used in the market analysis, product platform planning, product line planning and conceptual design etc, it can be used to get customer preference or utility of attribute and attribute level quantitatively, and also be used to look for the best combination of product/service module instances for customers [40,41]. The main steps of conjoint analysis are as follows.
(1) Determine module class and module instances of products or services
It needs to determine the attributes and attribute levels of product/services in conjoint analysis, ensure that the attribute and attribute level of product/services must be significant factors that affect customer purchase. In the planning of PSS service solution layer, the attributes are function needs of PSS modules, attribute levels are module instances collections of function needs.
(2) The simulation of PSS
Conjoint analysis consider all module classes and instances of PSS together, it uses the orthogonal design method to combine module classes and instances, and generates a series of simulation products. Conjoint analysis usually uses full-profile method, a combination which is constituted by all attributes in a certain level is called a profile, and each profile can be represented by a card. Because the amount of all the service solution combinations may be very huge, but it is unnecessary to evaluate all combination solution. In this paper, the orthogonal design method is adopted to reduce the numbers of combination solution and reflect the main effects much more.
(3) The market survey on customer preference
Research the customer's comments on all simulation product (profile), surveying the preference of simulation products, and by the method of scoring and sorting methods to survey the possibility of purchasing for customers.
(4) Calculate the utility of each solution's attributes
Get the customer preference values of each module class and module instances from the information that has been collected, these preference values are the utility value of the module classes. There are several methods to calculate the utility of module class, the method are as follows: ordinary least square regression model, multivariate analysis of variance model, LOGIT regression model. In this paper, the ordinary least square regression model is used to solve the utility value of module class. The utility function is given in formula 2.
U(X)=m∑i=1ki∑j=1βijXij | (2) |
Formula 2 shows that a PSS has
The conjoint analysis can estimate the utility value of each module class by establishing the equation between each module instance and user's scoring. For the conjoint analysis on m module classes and the i th module class has
The value of module instance which is estimated in this way shows the differences in the reference levels. If the value is positive, it suggests that the utility of this attribute level is higher than reference level; but if the value is negative, it suggests that the utility of attribute level is lower than reference level. Assuming that
Yhs=β0h+β1hX1hs+β2hX2hs+...+βthXths+ehs | (3) |
Among them,
The matrix is
According to the basic principles of OLS, minimizing the sum of square of the distance between straight line and each scattered point, that is minimizing the residual, as shown in type 4.
RSS=t∑i=1(Yths−ˆYths)2=t∑i=1(Yths−ˆα−Xthsˆβth) | (4) |
According to the minimize first-order conditions, get the partial derivative of
ˆβ=∑XthsYths−t¯XhsYhs∑X2ths−TX2hs,ˆα=ˉYhs−ˆβˉXhs | (5) |
(5) The importance of module instance
The importance degree of i th module instance is
Ii={max(aij)−min(aij)},i=1...m,j=1...ki | (16) |
In all module instances, the relative importance degree
Wi=Ii/m∑i=1Ii | (7) |
In the quantitative calculation of customer preferences of module instances, the module function of "Conjoint" of the Statistics Package for Social Science (SPSS) is usually used to do orthogonal design. Create the required orthogonal table, and make conjoint analysis on the possible principle combination.
Based on the qualitative analysis of customer's needs, score for these demands and get a number of parameter values of module classes and instances (utility value, deviation and importance degree), we can make decisions on module instances and module levels based on these parameter values. Specific principles are as follows:
(1) According to practical situation, the modules that have lower important degree can be classified into generic part layer; the modules that have higher important degree can be classified into services layer.
(2) Remove the redundant modules. Reduce some module instances of common modules, mandatory modules and optional modules before the decision-making; mainly remove some modules that have lower utility values.
(3) Remove common modules that are not involved in the decision-making, mandatory modules and optional modules in services solution layer are usually customer's decision-making modules, while mandatory modules and optional modules in generic part layer are usually manufacturer's decision-making modules.
Based on the module planning results of PSS services solution layer and generic part layer, the module constitution, module types, module instances in two layers (bi-level) can be determined. By using these module instances, the coarse structure and master structure of PSS can be established.
The modular coarse structure of PSS is mainly used for the module structure planning of services solution layer, it consists of one or two layers, and mainly composed of following modules: Core physical product modules, optional physical modules, free or supporting service modules, value-added service modules etc, the customer can get satisfied collection of products and services through the PSS coarse structure. In PSS modular coarse structure of Figure 3, dashed lines express the modular combinations of pure physical products, product-oriented PSS, use-oriented PSS and result-oriented PSS. The types of PSS are different, the module structures and its properties are also different. It is worth to point that: some modules are common modules, such as physical module ontology, free or supporting service modules etc., they are necessary for each type of PSS, and the other modules are optional modules.
The modular master structure of PSS is a complex hierarchy tree, it consists of J1 common module classes, J2 mandatory module classes, and J3 optional module classes (in Figure 4), and complex relationships among modules. Each class includes several module instances, these module instances can be selected and configured by customers, and forms a modular product instance or customized product [10]. In the PSS modular master structure, there are significant difference on module types, quantity and size in two levels for different types of PSS. The granularity and levels of different types of PSS are shown in Figure 3. Line ① is the separation line of bi-level modules of product-oriented PSS, line ② is the separation line of bi-level modules of use-oriented PSS, line ③ is the separation line of bi-level modules of result-oriented PSS. The modules above the dashed line belong to the upper level; while below the dashed line belong to the lower level. For the product-oriented PSS, the ownership of the tangible product is transferred to the consumer, but additional services are also provided. In module planning of product-oriented PSS, the customers will buy physical products and services, so the module granularity that customers participate in selection (decision making) is very fine. In the module planning of use-oriented PSS, the customers mainly rent physical products and services; the module granularity that the customers can choose is coarse, because they do not care much about the product internal structure, they care more about the reliability of products and services; but the module granularity that manufacturers can make decision is fine, because the reasonable product and service configuration will maximize their interests. In module planning of the result-oriented PSS, the customers just use the results of the products and services; they only care about whether the combination effects of products and services can maximize their service experience. So for the result-oriented PSS, the module granularity that customers participate in selecting is very coarse.
The power transformer has a long life cycle, and its effective life is about 20 years. In stages of sales, operation, maintenance, recycling and remanufacturing of the transformer, there are many types of product services, such as testing service, remote fault diagnosis service, monitoring service etc. PSS can also bring higher added value for the manufacturer, and improve the product's profitability and sustainability.
Use the questionnaire to collect the customer's service needs of the transformer, and use the Kano model to segment and understand customer needs, and divide customer needs into basic needs, performance needs and excitement needs.
Based on the module partition results from the Li's literature [8], 28 generalized module classes were got for the generalized transformer (includes service modules). These generalized module classes are as following: {Ontology transformer service transformer (including three kinds of PSS: Buy transformer and services, leasing transformer, buying the power services) (F1), wire box and control cable (F2), cooling unit (F3), temperature controller (F4), butterfly valve (F5), oil storage tank (F6), high pressure bushing (F7), low pressure bushing (F8), online filter oil machine (F9), gas relay (F10), pressure relief valve (F11), high and low voltage switch control cabinet (F12), chromatography monitoring IED components (F13), partial discharge monitoring IED (F14), casing insulation monitoring IED components (F15), core monitoring components (F16), winding temperature fiber monitoring IED components (F17), cooling unit monitoring IED (F18), routine testing (F19), type testing (F20), special testing (F21), the financial service (F22), transportation service (F23), installation service (F24), warranty maintenance service (F25), non-warranty maintenance services (F26), recycling service (F27), spare parts (F28)}. 60 potential customers and customers who have been used transformers are researched, the survey results are that: 35 customers choose to buy the transformer, 15 customers choose to lease transformer, and 10 customers choose to buy the power that after transformer services.
(1) Product-oriented transformer PSS
Kano scoring method is used in Tables 1, 2, the collection results of customer's demands of transformer PSS modules are shown in Table 3. F1 is the transformer ontology when buying the transformer.
Modules | Basic needs (B) | Performance needs (A) | Excitement needs (E) | Neutral results (I) | Opposite results (P) | Confusion results (D) |
F1 | 35 | |||||
F2 | 35 | |||||
F3 | 35 | |||||
F4 | 35 | |||||
F5 | 35 | |||||
F6 | 35 | |||||
F7 | 5 | 30 | ||||
F8 | 5 | 30 | ||||
F9 | 5 | 30 | ||||
F10 | 5 | 30 | ||||
F11 | 5 | 30 | ||||
F12 | 5 | 30 | ||||
F13 | 8 | 25 | 2 | |||
F14 | 8 | 25 | 2 | |||
F15 | 8 | 25 | 2 | |||
F16 | 8 | 25 | 2 | |||
F17 | 8 | 25 | 2 | |||
F18 | 8 | 25 | 2 | |||
F19 | 25 | 10 | ||||
F20 | 25 | 10 | ||||
F21 | 25 | 10 | ||||
F22 | 25 | 10 | ||||
F23 | 35 | |||||
F24 | 10 | 22 | 3 | |||
F25 | 25 | 10 | ||||
F26 | 10 | 20 | 5 | |||
F27 | 5 | 10 | 20 | |||
F28 | 30 | 5 |
(2) Use-oriented transformer services
According to the demand analysis of use-oriented PSS, some modules are not necessary to be configured; the modules that can be provided are shown in Table 4. F1 is the transformer ontology when leasing the transformer.
Modules | Basic needs (B) | Performance needs (A) | Excitement needs (E) | Neutral results (I) | Opposite results (P) | Confusion results (D) |
F1 | 15 | |||||
F2 | 15 | |||||
F3 | 15 | |||||
F4 | 15 | |||||
F5 | 15 | |||||
F6 | 15 | |||||
F7 | 15 | |||||
F8 | 15 | |||||
F9 | 15 | |||||
F10 | 15 | |||||
F11 | 15 | |||||
F12 | 5 | 10 | ||||
F13 | 3 | 10 | 2 | |||
F14 | 3 | 10 | 2 | |||
F15 | 3 | 10 | 2 | |||
F16 | 3 | 10 | 2 | |||
F17 | 3 | 10 | 2 | |||
F18 | 3 | 10 | 2 | |||
F19 | 3 | 10 | 2 | |||
F20 | 3 | 10 | 2 | |||
F21 | 3 | 10 | 2 | |||
F23 | 13 | 2 | ||||
F24 | 5 | 10 | ||||
F25 | 5 | 10 | ||||
F26 | 1 | 5 | 9 | |||
F28 | 10 | 5 |
(3) Result-oriented transformer services
On the module demand analysis of result-oriented PSS, some modules are not necessary to be configured, there are 10 investigators to participate in the survey among 60 investigators, the modules that can be provided are shown in Table 5. F1 is transformer ontology when selling transforming services.
Modules | Basic needs (B) | Performance needs (A) | Excitement needs (E) | Neutral results (I) | Opposite results (P) | Confusion results (D) |
F1 | 2 | 6 | 3 | |||
F2 | 10 | |||||
F3 | 10 | |||||
F4 | 10 | |||||
F5 | 10 | |||||
F6 | 10 | |||||
F7 | 8 | 2 | ||||
F8 | 8 | 2 | ||||
F9 | 8 | 2 | ||||
F10 | 8 | 2 | ||||
F11 | 8 | 2 | ||||
F12 | 7 | 3 | ||||
F13 | 6 | 2 | 2 | |||
F14 | 6 | 2 | 2 | |||
F15 | 6 | 2 | 2 | |||
F16 | 6 | 2 | 2 | |||
F17 | 6 | 2 | 2 | |||
F18 | 6 | 2 | 2 | |||
F19 | 7 | 2 | 1 | |||
F20 | 7 | 2 | 1 | |||
F21 | 7 | 2 | 1 | |||
F23 | 8 | 2 | ||||
F24 | 9 | 1 | ||||
F26 | 8 | 2 | ||||
F28 | 9 | 1 |
Scoring for the six kinds of research results, the scores are as follows: C(B) = 0.2, C(A) = 0.3, C(E) = 0.4, C(I) = −0.1, C(P) = C(D) = 0. The customer preference values of each module class can be got according to the formula 1, and are shown in Figure 5, Figure 6 and Figure 7 respectively.
According to the customer preference value analysis of product-oriented transformer in Figure 5, the values of S can be set as 7.5 and 10.0. For the customer, the modules that below 7.0 are common modules, the modules that between 7.5–10.0 are mandatory modules, the modules that above 10.0 are optional modules. Therefore, common modules of product-oriented transformer are as follows: Transformer ontology, wire box and control cable, cooling unit, temperature controller, butterfly valve, oil storage tank, transportation services, spare parts etc. Mandatory modules are as follows: High pressure bushing, low pressure bushing, online filter oil machine, gas relay, pressure relief valve, installation services, warranty maintenance services, non-warranty maintenance services. The optional service modules include: high and low voltage switch control cabinet, chromatographic monitoring IED components, partial discharge monitoring IED components, casing insulation monitoring IED components, core monitoring IED components, winding temperature fiber monitoring IED components, cooling unit monitoring IED components, routine testing, type testing, special testing, financial service and recycling service etc.
According to the customer preference values analysis of use-oriented transformer in Figure 6, the values of S can be set as 3.5 and 4.0. For the customer, the modules below 3.5 are basic modules, the modules between 3.5–4.0 are mandatory modules, the modules that above 4.0 are optional modules. So common modules of use-oriented transformer services are as follows: Transformer ontology, wire box and control cable, cooling unit, temperature controller, butterfly valve, oil storage tank, high pressure bushing, low pressure bushing, online filter oil machine, gas relay, pressure relief valve, transportation services, spare parts etc. Mandatory modules are as follows: High and low voltage switch control cabinet, warranty maintenance services. Optional modules include: chromatographic monitoring IED components, partial discharge monitoring IED components, casing insulation monitoring IED components, core monitoring IED components, winding temperature fiber monitoring IED components, cooling unit monitoring IED components, routine testing, type testing, special testing, installation service and non-warranty maintenance services etc.
According to the customer preference values analysis of result-oriented transformer in Figure 7, the value of S can be set as 2.7. For the customer, the modules that below 2.7 are common modules, the modules that above 2.7 are mandatory modules. So the mandatory module of result-oriented transformer services is transformer ontology. Common modules are as follows: Wire box and control cable, heat sinks, temperature controller, butterfly valve, oil storage tank, high pressure bushing, low pressure bushing, online filter oil machine, gas relay, pressure relief valve, transportation services, spare parts, high and low voltage switch control cabinet, chromatographic monitoring IED component, partial discharge monitoring IED components, casing insulation monitoring IED components, core monitoring IED components, winding temperature fiber monitoring IED components, cooling unit monitoring IED components, routine testing, type testing, special testing, installation services and non-warranty maintenance services etc.
Assume that, all types of PSS that the customers need can be provided by transformer manufacturers. Currently, the mainstream category of transformer for small and medium-sized group customers are 10 KV power transformers, the main types of 10 KV power transformers are as follows: S9, S9-M, SG10, S11, S11-M. Among them, the type of S9 is more energy consumption; the type of S11 is the most energy-efficient. When customers buy transformers, they also can choose to lease transformer (result-oriented) or buy the electricity services (use-oriented), but the two business models that lease transformer or buy the electricity services both need the support of auxiliary physical and service modules, such as high and low voltage switchgear etc.
Several methods can be used to seek the module instances for module classes, such as analogy method, brain storming method, association method etc. Finish the collection of instance modules, and get the solution set shown in Table 6.
![]() |
MI1 | MI2 | MI3 | ||
N1 | Transforming service | Purchase transformer | S9 | S10 | S11 |
Lease transformer | S9 | S10 | S11 | ||
Purchase electric power | S9 | S10 | S11 | ||
N2 | Basic auxiliary modules of the transformer | Wire box and control cable | JC1 | JC2 | |
Cooling unit | |||||
Temperature controller | |||||
Butterfly valve | |||||
Oil storage tank | |||||
N3 | High pressure bushing | COT-550/800 | COT-551/800 | COT-552/800 | |
N4 | Low pressure bushing | BD-20/3150 | BD-21/3151 | BD-22/3152 | |
N5 | Online filter oil machine | R1 | R2 | ||
N6 | Gas relay | BF-80/10 | BF-88/10 | ||
N7 | Pressure relief valve | YSF8-55/130KJ | YSF8-56/130KJ | ||
N8 | High and low voltage switch control cabinet | K1 | K2 | K3 | |
N9 | Monitoring services | Chromatography monitoring | iMGA2020 | iMGA2021 | |
N10 | Partial discharge monitoring | iPDM2020T | iPDM2021T | ||
N11 | Casing insulation monitoring | iIMM2020 | iIMM2021 | ||
N12 | Core monitoring | iOCM2020 | iOCM2021 | ||
N13 | Winding temperature fiber monitoring | iOFT2020 | iOFT2021 | ||
N14 | Cooling unit monitoring | iCSM2020 | iCSM2021 | ||
N15 | Testing services | Routine testing | Routine testing | ||
N16 | Typical testing | Typical testing | |||
N17 | Special testing | Special testing | |||
N18 | Financial services | Manufacturer loan | The third party loan | Bank installment loan | |
N19 | Transportation services | The third party transporting | Seller transporting | Customer transporting | |
N20 | Installation services | The third party installation | Seller installation | ||
N21 | Maintenance services | Warranty maintenance services | One-year warranty | Two-year warranty | Three-year warranty |
N22 | Non-warranty maintenance services | Routine maintenance | Full services | ||
N23 | Recycling services | Old for new | Full recovery | Non-recovery | |
N24 | Spare parts | SP1 | SP2 |
(1) The instance module collection for customers needs
The combination planning and analysis of function solution for transformer is the combination of all products and services in the product life cycle. For the solution of all instance modules, we need to add the option of "no choice" and the price factor of customer's service needs, and form the most complete collection of module instances for customer's needs, as shown in Table 7.
![]() |
MI1 | MI2 | MI3 | MI4 | |||
N1 | Transforming service | Purchase transformer | S9 | S10 | S11 | ||
Lease transformer | S9 | S10 | S11 | ||||
Purchase electric power | S9 | S10 | S11 | ||||
N2 | Basic auxiliary modules of the transformer | JC1 | JC2 | ||||
N3 | High pressure bushing | COT-550/800 | COT-551/800 | COT-552/800 | |||
N4 | Low pressure bushing | BD-20/3150 | BD-21/3151 | BD-22/3152 | |||
N5 | Online filter oil machine | R1 | R2 | ||||
N6 | Gas relay | BF-80/10 | BF-88/10 | ||||
N7 | Pressure relief valve | YSF8-55/130KJ | YSF8-56/130KJ | ||||
N8 | High and low voltage switch control cabinet | K1 | K2 | K3 | Not supply | ||
N9 | Monitoring services | Chromatography monitoring IED component | iMGA2020 | iMGA2021 | Not monitoring | ||
N10 | Partial discharge monitoring IED component | iPDM2020T | iPDM2021T | Not monitoring | |||
N11 | Casing insulation monitoring IED component | iIMM2020 | iIMM2021 | Not monitoring | |||
N12 | Core monitoring component | iOCM2020 | iOCM2021 | Not monitoring | |||
N13 | Winding temperature fiber monitoring IED component | iOFT2020 | iOFT2021 | Not monitoring | |||
N14 | Cooling unit monitoring IED | iCSM2020 | iCSM2021 | Not monitoring | |||
N15 | Testing services | Routine testing | Routine testing | Not testing | |||
N16 | Testing services Routine testing Typical testing Special testing |
Typical testing | Typical testing | Not testing | |||
N17 | Testing services Routine testing Typical testing Special testing |
Special testing | Special testing | Not testing | |||
N18 | Financial services | Manufacturer loan | The third party loan | Bank installment loan | Not loan | ||
N19 | Transportation service s | The third party transporting | Seller transporting | Customer transporting | |||
N20 | Installation services | The third party installation | Seller installation | ||||
N21 | Maintenance services | Warranty maintenance services | One-year warranty | Two-year warranty | Three-year warranty | ||
N22 | Non-warranty maintenance services | Routine maintenance | Full services | ||||
N23 | Recycling services | Old for new | Full recovery | Non-recovery | |||
N24 | Spare parts | SP1 | SP2 |
To simplify the calculation, the module instances can be symbolic expression (in Table 8). Eij is the j th module instance in the i th module class.
![]() |
S1 | S2 | S3 | S4 | ![]() |
S1 | S2 | S3 | S4 |
N1 | E11 | E12 | E13 | N13 | E131 | E132 | E133 | ||
N2 | E21 | E22 | N14 | E141 | E142 | E143 | |||
N3 | E31 | E32 | E33 | N15 | E151 | E152 | |||
N4 | E41 | E42 | E43 | N16 | E161 | E162 | |||
N5 | E51 | E52 | N17 | E171 | E172 | ||||
N6 | E61 | E62 | N18 | E181 | E182 | E183 | E184 | ||
N7 | E71 | E72 | N19 | E191 | E192 | E193 | |||
N8 | E81 | E82 | E83 | E84 | N20 | E201 | E202 | ||
N9 | E91 | E92 | E93 | N21 | E211 | E212 | E213 | ||
N10 | E101 | E102 | E103 | N22 | E221 | E222 | |||
N11 | E111 | E112 | E113 | N23 | E231 | E232 | E231 | ||
N12 | E121 | E122 | E123 | N24 | E241 | E242 |
(2) Analyze the conflicts and eliminate redundant principle solution
There are 13060694016 kinds of combination from the theoretical calculation. But in the actual product planning, there are some conflicts between modules, so that they are not suitable to form solutions, so it is necessary to analyze the conflicts between different modules to eliminate redundant combination. When customers choose "variable pressure services", they can only choose one type from three types in F1, and after the selection, the choice for the other service modules will be strict restrained. So three types of PSS should be chosen separately, it is worth to note that the module types and their properties will be different for different types of PSS. The possible module instances collections are shown in Tables 9, 10 and 11.
Instances Module classes |
S1 | S2 | S3 | S4 | Instances Module classes |
S1 | S2 | S3 | S4 |
N1 | E11 | E12 | E13 | N13 | E131 | E132 | E133 | ||
N2 | E21 | E22 | N14 | E141 | E142 | E143 | |||
N3 | E31 | E32 | E33 | N15 | E151 | E152 | |||
N4 | E41 | E42 | E43 | N16 | E161 | E162 | |||
N5 | E51 | E52 | N17 | E171 | E172 | ||||
N6 | E61 | E62 | N18 | E181 | E182 | E183 | E184 | ||
N7 | E71 | E72 | N19 | E191 | E192 | E193 | |||
N8 | E81 | E82 | E83 | E84 | N20 | E201 | E202 | ||
N9 | E91 | E92 | E93 | N21 | E211 | E212 | E213 | ||
N10 | E101 | E102 | E103 | N22 | E221 | E222 | |||
N11 | E111 | E112 | E113 | N23 | E231 | E232 | E231 | ||
N12 | E121 | E122 | E123 | N24 | E241 | E242 |
![]() |
S1 | S2 | S3 | S4 | ![]() |
S1 | S2 | S3 | S4 |
N1 | E11 | E12 | E13 | N12 | E121 | E122 | E123 | ||
N2 | E21 | E22 | N13 | E131 | E132 | E133 | |||
N3 | E31 | E32 | E33 | N14 | E141 | E142 | E143 | ||
N4 | E41 | E42 | E43 | N15 | E151 | E152 | |||
N5 | E51 | E52 | N16 | E161 | E162 | ||||
N6 | E61 | E62 | N17 | E171 | E172 | ||||
N7 | E71 | E72 | N19 | E191 | E192 | E193 | |||
N8 | E81 | E82 | E83 | E84 | N20 | E201 | E202 | ||
N9 | E91 | E92 | E93 | N21 | E211 | E212 | E213 | ||
N10 | E101 | E102 | E103 | N22 | E221 | E222 | |||
N11 | E111 | E112 | E113 | N24 | E241 | E242 |
Instances Module classes |
S1 | S2 | S3 | Instances Module classes |
S1 | S2 | S3 |
N1 | E11 | E12 | E13 | N12 | E121 | E122 | |
N2 | E21 | E22 | N13 | E131 | E132 | ||
N3 | E31 | E32 | E33 | N14 | E141 | E142 | |
N4 | E41 | E42 | E43 | N15 | E151 | E152 | |
N5 | E51 | E52 | N16 | E161 | E162 | ||
N6 | E61 | E62 | N17 | E171 | E172 | ||
N7 | E71 | E72 | N19 | E191 | E192 | E193 | |
N8 | E81 | E82 | E83 | N20 | E201 | E202 | |
N9 | E91 | E92 | N22 | E221 | E222 | ||
N10 | E101 | E102 | N24 | E241 | E242 | ||
N11 | E111 | E112 |
(1) Calculate the utility value and importance degree of PSS
The SPSS software can be used to calculate the utility value and importance degree of PSS, each service solution need to be scored by investigators. Scores range are as shown in Table 12, 9 means "buy", 1 means "not buy", from 9 to 1, the possibility of buying is gradually reduced. According to the random survey from potential customers, and obtain scores for each solution, then the utility value of the solutions can be calculated.
Not buy | 1 | 2 | 3 | 4 | 5 | 6 | 7 | 8 | 9 | Buy |
A total of 60 potential customers are selected for the survey, they are requested to score for every service solution independently. The orthogonal experimental design of service solutions F1, F2 and F3 that are chose by users can respectively generate 64 random card sets. In 60 potential customers, 35 customers choose to purchase transformers (F1), 15 customers choose to lease transformer (F2), and 10 customers choose to purchase transforming service directly (F3). The orthogonal card and scoring results of F1 services solution are shown in Table 13.
Card No. | Buy transformer ontology y | Basic auxiliary modules | High pressure bushing | Low pressure bushing | Online filter oil machine | Gas relay | Pressure relief valve | High and low voltage switch control cabinet | … | Spare parts | Scoring 1 | Scoring 2 | Scoring 3 | Scoring 4 | … | Scoring 34 | Scoring 35 |
1 | E11 | E21 | E32 | E41 | E51 | E62 | E72 | E82 | … | E242 | 6 | 7 | 5 | 5 | … | 5 | 4 |
2 | E12 | E21 | E31 | E42 | E51 | E62 | E72 | E84 | … | E242 | 7 | 8 | 7 | 8 | … | 4 | 6 |
3 | E11 | E21 | E32 | E41 | E51 | E61 | E72 | E82 | … | E242 | 5 | 6 | 6 | 6 | … | 6 | 5 |
4 | E11 | E21 | E32 | E41 | E51 | E62 | E72 | E82 | … | E242 | 7 | 7 | 7 | 8 | … | 5 | 6 |
5 | E11 | E21 | E32 | E43 | E52 | E61 | E71 | E82 | … | E241 | 8 | 6 | 8 | 8 | … | 7 | 6 |
6 | E12 | E21 | E31 | E43 | E51 | E61 | E72 | E82 | … | E242 | 6 | 6 | 5 | 5 | … | 6 | 6 |
7 | E12 | E21 | E32 | E42 | E52 | E62 | E71 | E83 | … | E241 | 4 | 5 | 3 | 3 | … | 5 | 2 |
8 | E11 | E21 | E32 | E41 | E52 | E62 | E72 | E83 | … | E241 | 5 | 5 | 6 | 6 | … | 4 | 1 |
9 | E11 | E21 | E33 | E43 | E52 | E62 | E72 | E84 | … | E242 | 8 | 9 | 7 | 7 | … | 6 | 8 |
10 | E12 | E21 | E31 | E42 | E51 | E61 | E71 | E82 | … | E242 | 6 | 6 | 7 | 5 | … | 5 | 6 |
11 | E11 | E21 | E32 | E43 | E51 | E61 | E71 | E83 | … | E242 | 4 | 3 | 5 | 5 | … | 5 | 5 |
12 | E11 | E21 | E31 | E43 | E52 | E62 | E71 | E82 | … | E242 | 5 | 3 | 5 | 6 | … | 6 | 4 |
13 | E13 | E21 | E32 | E41 | E51 | E62 | E72 | E82 | … | E242 | 6 | 6 | 7 | 5 | … | 5 | 6 |
… | … | … | … | … | … | … | … | … | … | … | … | … | … | … | … | … | … |
63 | E13 | E21 | E31 | E43 | E52 | E61 | E72 | E84 | … | E242 | 4 | 5 | 5 | 5 | … | 4 | 4 |
64 | E11 | E21 | E32 | E41 | E52 | E62 | E71 | E84 | … | E242 | 6 | 7 | 5 | 5 | … | 6 | 6 |
Scoring for 64 solutions of F1, and the scoring results are put in the conjoint analysis program of SPSS software, the utility values and importance degree of the module classes are obtained finally, as shown in Figure 8.
Scoring for F2 and F3 in accordance with the above steps respectively, we can get the utility values and important degree of each module instances and module class. The final attribute values are shown in Tables 14, 15 and 16.
Modules | The utility | Standard error | The importance degree | Modules | The utility | Standard error | The importance degree | ||
Buy transformer | S9 | 0.127 | 0.13 | 1.976 | Winding temperature fiber monitoring | iOFT2020 | −0.086 | 0.13 | 4.703 |
S10 | −0.069 | 0.152 | iOFT2021 | 0.233 | 0.152 | ||||
S11 | −0.058 | 0.152 | Not monitoring | −0.147 | 0.152 | ||||
Basic auxiliary modules of the transformer | JC1 | 0.047 | 0.097 | 1.643 | Cooling unit monitoring | iCSM2020 | 0.196 | 0.13 | 6.094 |
JC2 | −0.047 | 0.097 | iCSM2021 | 0.111 | 0.152 | ||||
High pressure bushing | COT-550/800 | 0.028 | 0.13 | 4.738 | Not monitoring | −0.307 | 0.152 | ||
COT-551/800 | 0.046 | 0.152 | Routine testing | Routine testing | 0.173 | 0.097 | 4.317 | ||
COT-552/800 | −0.074 | 0.152 | Not testing | −0.173 | 0.097 | ||||
Low pressure bushing | BD-20/3150 | −0.243 | 0.13 | 6.611 | Typical testing | Typical testing | 0.162 | 0.097 | 4.028 |
BD-21/3151 | 0.433 | 0.152 | Not testing | −0.162 | 0.097 | ||||
BD-22/3152 | −0.19 | 0.152 | Special testing | Special testing | 0.358 | 0.097 | 6.469 | ||
Online filter oil machine | R1 | 0.066 | 0.097 | 4.63 | Not testing | −0.358 | 0.097 | ||
R2 | −0.066 | 0.097 | Financial services | Manufacturer loan | 0.085 | 0.168 | 4.774 | ||
Gas relay | BF-80/10 | −0.01 | 0.097 | 2.335 | The third party loan | 0.16 | 0.168 | ||
BF-88/10 | 0.01 | 0.097 | Bank installment loan | −0.15 | 0.168 | ||||
Pressure relief valve | YSF8-55/130KJ | 0.017 | 0.097 | 2.684 | Not loan | −0.095 | 0.168 | ||
YSF8-56/130KJ | −0.017 | 0.097 | Transportation services | The third party transporting | −0.186 | 0.13 | 4.666 | ||
High and low voltage switch control cabinet | K1 | −0.388 | 0.168 | 5.314 | Seller transporting | 0.021 | 0.152 | ||
K2 | 0.271 | 0.168 | Customer transporting | 0.165 | 0.152 | ||||
K3 | 0.169 | 0.168 | Installation services | The third party installation | −0.06 | 0.097 | 3.485 | ||
Not provided | −0.052 | 0.168 | Seller installation | 0.06 | 0.097 | ||||
Chromatography monitoring | iMGA2020 | −0.157 | 0.13 | 5.241 | Warranty maintenance services | One-year warranty | 0.185 | 0.13 | 5.039 |
iMGA2021 | 0.114 | 0.152 | Two-year warranty | −0.236 | 0.152 | ||||
Not monitoring | 0.043 | 0.152 | Three-year warranty | 0.051 | 0.152 | ||||
Partial discharge monitoring | iPDM2020T | −0.216 | 0.13 | 4.778 | Non-warranty maintenance services | Routine maintenance | 0.233 | 0.097 | 4.272 |
iPDM2021T | 0.028 | 0.152 | Full services | −0.233 | 0.097 | ||||
Not monitoring | 0.188 | 0.152 | Recycling services | Old for new | 0.017 | 0.13 | 1.555 | ||
Casing insulation monitoring | iIMM2020 | −0.042 | 0.13 | 4.468 | Full recovery | 0.4 | 0.152 | ||
iIMM2021 | 0.016 | 0.152 | Non-recovery | −0.418 | 0.152 | ||||
Not monitoring | 0.026 | 0.152 | Spare parts | SP1 | 0.125 | 0.097 | 1.425 | ||
Core monitoring | iOCM2020 | 0.051 | 0.13 | 4.755 | SP2 | −0.125 | 0.097 | ||
iOCM2021 | 0.155 | 0.152 | |||||||
Not monitoring | −0.206 | 0.152 | |||||||
(constant) | 5.75 | 0.148 | (constant) | 5.75 | 0.148 |
Modules | The utility | Standard error | The importance degree | Modules | The utility | Standard error | The importance degree | ||
Lease transformer | S9 | −0.206 | 0.261 | 2.062 | Core monitoring | iOCM2020 | 0.108 | 0.261 | 6.721 |
S10 | −0.51 | 0.307 | iOCM2021 | 0.31 | 0.307 | ||||
S11 | 0.715 | 0.307 | Not monitoring | −0.419 | 0.307 | ||||
Basic auxiliary modules of the transformer | JC1 | −0.077 | 0.196 | 2.173 | Winding temperature fiber monitoring | iOFT2020 | 0.114 | 0.261 | 3.857 |
JC2 | 0.077 | 0.196 | iOFT2021 | −0.205 | 0.307 | ||||
High pressure bushing | COT-550/800 | −0.175 | 0.261 | 5.197 | Not monitoring | 0.091 | 0.307 | ||
COT-551/800 | 0.327 | 0.307 | Cooling unit monitoring | iCSM2020 | −0.05 | 0.261 | 3.28 | ||
COT-552/800 | −0.152 | 0.307 | iCSM2021 | −0.058 | 0.307 | ||||
Low pressure bushing | BD-20/3150 | −0.481 | 0.261 | 7.479 | Not monitoring | 0.108 | 0.307 | ||
BD-21/3151 | 0.317 | 0.307 | Routine testing | Routine testing | −0.081 | 0.196 | 4.904 | ||
BD-22/3152 | 0.163 | 0.307 | Not testing | 0.081 | 0.196 | ||||
Online filter oil machine | R1 | −0.085 | 0.196 | 2.263 | Typical testing | Typical testing | −0.281 | 0.196 | 4.85 |
R2 | 0.085 | 0.196 | Not testing | 0.281 | 0.196 | ||||
Gas relay | BF-80/10 | −0.013 | 0.196 | 2.167 | Special testing | Special testing | 0.027 | 0.196 | 4.971 |
BF-88/10 | 0.013 | 0.196 | Not testing | −0.027 | 0.196 | ||||
Pressure relief valve | YSF8-55/130KJ | −0.142 | 0.196 | 2.771 | Transportation services | The third party transporting | 0.133 | 0.261 | 3.362 |
YSF8-56/130KJ | 0.142 | 0.196 | Seller transporting | −0.079 | 0.307 | ||||
High and low voltage switch control cabinet | K1 | 0.25 | 0.34 | 7.549 | Customer transporting | −0.054 | 0.307 | ||
K2 | −0.4 | 0.34 | Installation services | The third party installation | 0.044 | 0.196 | 4.592 | ||
K3 | 0.142 | 0.34 | Seller installation | −0.044 | 0.196 | ||||
Not providing | 0.008 | 0.34 | Maintenance services | One-year warranty | −0.292 | 0.261 | 6.278 | ||
Chromatography monitoring | iMGA2020 | −0.178 | 0.261 | 4.384 | Two-year warranty | 0.31 | 0.307 | ||
iMGA2021 | −0.09 | 0.307 | Three-year warranty | −0.019 | 0.307 | ||||
Not monitoring | 0.268 | 0.307 | Non-warranty maintenance services | Routine maintenance | 0.127 | 0.196 | 2.935 | ||
Partial discharge monitoring | iPDM2020T | 0.169 | 0.261 | 8.459 | Full services | −0.127 | 0.196 | ||
iPDM2021T | −0.541 | 0.307 | Spare parts | SP1 | −0.004 | 0.196 | 2.313 | ||
Not monitoring | 0.372 | 0.307 | SP2 | 0.004 | 0.196 | ||||
Casing insulation monitoring | iIMM2020 | −0.044 | 0.261 | 7.433 | |||||
iIMM2021 | −0.374 | 0.307 | |||||||
Not monitoring | 0.418 | 0.307 | |||||||
(constant) | 5.587 | 0.292 | (constant) | 5.587 | 0.292 |
Modules | The utility | Standard error | The importance degree | Modules | The utility | Standard error | The importance degree | ||
Purchase electric power | S9 | −0.16 | 0.16 | 11.606 | Casing insulation monitoring | iIMM2020 | −0.048 | 0.12 | 5.825 |
S10 | 0.068 | 0.188 | iIMM2021 | 0.048 | 0.12 | ||||
S11 | 0.093 | 0.188 | Core monitoring | iOCM2020 | 0.183 | 0.12 | 4.038 | ||
Basic auxiliary modules of the transformer | JC1 | 0.114 | 0.12 | 3.013 | iOCM2021 | −0.183 | 0.12 | ||
JC2 | −0.114 | 0.12 | Winding temperature fiber monitoring | iOFT2020 | 0.117 | 0.12 | 3.555 | ||
High pressure bushing | COT-550/800 | −0.198 | 0.16 | 5.665 | iOFT2021 | −0.117 | 0.12 | ||
COT-551/800 | 0.252 | 0.188 | Cooling unit monitoring | iCSM2020 | −0.089 | 0.12 | 4.352 | ||
COT-552/800 | −0.054 | 0.188 | iCSM2021 | 0.089 | 0.12 | ||||
Low pressure bushing | BD-20/3150 | −0.035 | 0.16 | 5.875 | Routine testing | Routine testing | −0.011 | 0.12 | 4.021 |
BD-21/3151 | 0.099 | 0.188 | Not testing | 0.011 | 0.12 | ||||
BD-22/3152 | −0.064 | 0.188 | Typical testing | Typical testing | 0.008 | 0.12 | 4.636 | ||
Online filter oil machine | R1 | 0.123 | 0.12 | 4.376 | Not testing | −0.008 | 0.12 | ||
R2 | −0.123 | 0.12 | Special testing | Special testing | 0.205 | 0.12 | 4.405 | ||
Gas relay | BF-80/10 | −0.092 | 0.12 | 5.005 | Not testing | −0.205 | 0.12 | ||
BF-88/10 | 0.092 | 0.12 | Transportation services | The third party transporting | 0.352 | 0.16 | 4.170 | ||
Pressure relief valve | YSF8-55/130KJ | 0.142 | 0.12 | 4.986 | Seller transporting | −0.31 | 0.188 | ||
YSF8-56/130KJ | −0.142 | 0.12 | Customer transporting | −0.042 | 0.188 | ||||
High and low voltage switch control cabinet | K1 | 0.458 | 0.208 | 4.098 | Installation services | The third party installation | −0.027 | 0.12 | 3.521 |
K2 | −0.405 | 0.208 | Seller installation | 0.027 | 0.12 | ||||
K3 | −0.211 | 0.208 | Non-warranty maintenance services | S1 | −0.014 | 0.12 | 3.801 | ||
Not providing | 0.158 | 0.208 | S2 | 0.014 | 0.12 | ||||
Chromatography monitoring | iMGA2020 | 0.002 | 0.12 | 4.412 | Spare parts | SP1 | 0.18 | 0.12 | 4.248 |
iMGA2021 | −0.002 | 0.12 | SP2 | −0.18 | 0.12 | ||||
Partial discharge monitoring | iPDM2020T | −0.202 | 0.12 | 4.392 | |||||
iPDM2021T | 0.202 | 0.12 | |||||||
(constant) | 5.621 | 0.144 |
(2) The decision-making planning of module levels for generalized transformer PSS
Based on the module planning principles of services solution layer and generic part layer, the decision-making on module instances and module levels can be carried out respectively.
1) Selling the transformer (product-oriented PSS)
In Table 14, the importance degree of transformer ontology, recycling service, basic auxiliary module and spare parts in customers choices are in lower level (less than or equal to 1.976), customers have low interests on them, so they belong to decision-making modules of generic part layer. Because there are not two or more instances for the basic auxiliary module, so they can be classified as product basic modules and do not participate in the customer's choice (decision making). The modules that the customer makes decision are these have high importance degree (more than or equal to 2.335), such as: Auxiliary transformer ontology modules (the high pressure bushing, low pressure bushing, online filter oil machine, gas relay, pressure release valve), spare parts, transportation services, high and low voltage switch control cabinet, monitoring services, testing services, financial services, installation service, warranty maintenance services, no-warranty maintenance services, etc.
According to the practical situation of modules, remove some module instances that have low utility values (the modules that have negative utility values) appropriately, which can reduce the customer's option on module instances and the cost of production. The instance modules that can be removed are follows: The JC2 instance in basic auxiliary transformer module, "not recycling" instance in recycling requirements, the SP2 of spare parts, the "iMGA2020" in chromatographic monitoring IED components, the "iPDM2020T" in partial discharge monitoring IED components, the "iIMM2020" in casing insulation monitoring IED components, the "iOFT2020" in winding temperature fiber monitoring IED components, the "iOMM2020" in core monitoring components, "the third party enterprise installation" in installation service module, "bank payment by installment" in the financial service module, "user transportation" and "sales enterprise transportation" in transportation service, etc.
Remove some common modules that do not participation in decision-making, such as: Basic auxiliary modules, spare parts etc.
Based on the above analysis, transformer ontology, old change new services and fully recycling service are modules in the lower level that the decision are made by manufacturers. Customer's decision-making modules include: basic auxiliary modules (the high pressure bushing, low pressure bushing, online filter oil machine, gas relay, pressure release valve), spare parts, transportation services, warranty maintenance services, high and low voltage switch control cabinet, monitoring services, the other optional services, etc. Common modules that do not participate in decision-making are directly passed from the service solution layer to the generic parts layer. After the exclusion of some modules, the rest modules form module instances collection.
2) Use-oriented transformer
According to the decision-making process of selling transformer(product-oriented PSS) and Table 15, when providing use-oriented transformer, we can get the upper level modules of customer's decision-making are follows: high pressure bushing, low pressure bushing, online filter oil machine, gas relay, pressure release valve, high and low voltage switch control cabinet, warranty maintenance services, no-warranty maintenance services, installation services, financial services, testing services, monitoring services, recycling services, etc. The lower level modules of manufacturer's decision-making include transformer ontology, mandatory modules, etc. The common modules that do not participate in the decision-making include: basic auxiliary modules, spare parts, transportation services.
The instance modules that can be excluded include: The instance"JC1" in basic auxiliary modules, "SP1" in spare parts, "iMGA2020" in chromatographic monitoring IED components, "iPDM2021T" in partial discharge monitoring IED component, "iIMM2021" in casing insulation monitoring IED components, "iOMM2020" in core monitoring components, "iOFT2021" in winding temperature fiber monitoring IED components, "the third party enterprise installation" in installation services, "user transportation" and "sales enterprise transportation" in transportation services, etc.
3) Result-oriented transformer
Based on the above decision-making process of selling transformer (product-oriented PSS) and Table 16, when providing result-oriented transformer for the manufacturer, customers buy the electricity and not buy transformer equipment. The upper level decision-making for the customer is the types of transformer, such as (S9, S10 and S11), the types of transformer directly determine the price of result-oriented transformer. The modules in generic part layer include: high pressure bushing, low pressure bushing, online filter oil machine, gas relay, pressure release valve, high and low voltage switch control cabinet, etc. The basic auxiliary modules, spare parts, transportation services, testing services, monitoring services and the other optional services belong to common modules. Only the left instance modules in common service modules that have the highest utility values.
Instance modules that are removed include: "JC2" in basic auxiliary module, "SP2" in spare parts, "iMGA2020" in chromatographic monitoring IED components, "iPDM2020T" in partial discharge monitoring IED components, "iIMM2020" in casing insulation monitoring IED components, "iOMM2020" in core monitoring components, "iOFT2021" in winding temperature fiber monitoring IED components, "the third party enterprise installation" in installation services, "user transportation" and "sales enterprise transportation" in transportation services, etc.
The coarse structure in services solution layer consists of decision-making modules and basic modules, which is mainly used for services solution configuration for customers. Based on the analysis results of the module types and level planning, we can get the coarse structure of generalized transformer. The coarse structure and master structure of product-oriented transformer are shown in Figures 9 and 10. The master structure of use-oriented transformer and result-oriented transformer are shown in Figures 11 and 12.
In order to build the PSS modular master structure, the PSS module types should be determined, as well as the customer's decision-making modules and their attributes in services solution layer and the manufacturer's decision-making modules and their attributes in generic part layer. This is premise and foundation work of establishing master structure of PSS and carrying out modular configuration design.
In Figures 10, 11 and 12, we can find that module granularity and number are different in upper level and lower for types of PSS. In the upper level, from the product-oriented PSS to result-oriented PSS, the module granularity becomes increasingly coarse, and more and more service modules become common modules, this indicates that users are increasingly not concerned about the internal structure of product; instead, they pay more attention to service experiences. In the lower level, from the product-oriented PSS to result-oriented PSS, manufacturers make more decision on the physical modules; this indicates that manufacturers should lower manufacturing costs in response to result-oriented PSS.
For the results we can conclude in two aspects. (1) In the configuration design process of different types of PSS, we must identify module categories respectively, because modules are different for different types of PSS. (2) The decision-making on module levels and properties are very important, which will decide who (customers or manufacturers) can choose more module instances; this will balance the manufacturer's profit and customer satisfaction on different types of PSS.
This paper proposes a method for the planning of PSS bi-level modular architecture, the methodology consists of five steps, respectively: Obtain service requirements, determine the types of generalized module, find the feasible solution for each module class, the module planning of service solution layer and generic part layer, establish the modular coarse structure and master structure, etc. There are two main steps in this methodology: (1) the module types of different types of PSS are classified based on the Kano model. (2) Based on conjoint analysis, the customer's decision-making modules and their properties and the manufacturer's decision-making modules and their properties in different types of PSS are planned.
The limitations of this paper are as follows: (1) a typical modular master structure not only includes different types of module classes and instances, but also includes module descriptions, configuration and constraint rules. However, how to describe the service module in modular master structure needs further research. (2) In order to build an effective modular master structure, it may take a lot of time and effort to carry out these methods and requires extensive participation by the designers and customers.
This work has been supported by the National Natural Science Foundation of China (No. 51775517), the Plan for Scientific Innovation Talent of Henan Province (No. 184100510007).
All authors declare no conflicts of interest in this paper.
[1] | EIA (2010) Annual energy outlook 2010: with projections to 2035. Government Printing Office. |
[2] | Santacana E, Rackliffe G, Tang Le, et al. (2010) Getting smart. IEEE Power Energy Mag 8: 41-48. |
[3] | Jiayi H, Jiang C, Xu R (2008) A review on distributed energy resources and MicroGrid. Renewable Sustainable Energy Rev 12: 2472-2483. |
[4] | Driesen J, Vermeyen P, Belmans R (2007) Protection issues in microgrids with multiple distributed generation units. Power Conversion Conference, Nagoya 646-653. |
[5] | Gopalan SA, Victor S, Herbert HI (2014) A review of coordination strategies and protection schemes for microgrids. Renewable Sustainable Energy Rev 32: 222-228. |
[6] | Sarwagya K, Paresh KN (2015) An extensive review on the state-of-art on microgrid protection. 2015 IEEE Power, Communication and Information Technology Conference 862-868. |
[7] | Zhang Y, Dougal RA (2012) State of the art of fault current limiters and their applications in smart grid. 2012 IEEE Power and Energy Society General Meeting 1-6. |
[8] | Morandi A (2013) State of the art of superconducting fault current limiters and their application to the electric power system. Phys C: Supercond 484: 242247. |
[9] | Aly MM, Emad AM (2012) Comparison between resistive and inductive superconducting fault current limiters for fault current limiting. 2012 Seventh International Conference on Computer Engineering & Systems (ICCES) 227-232. |
[10] | Choudhary NK, Mohanty SR, Singh RK, et al. (2014) A review on microgrid protection. 2014 International Electrical Engineering Congress (iEECON) 1-4. |
[11] | Zheng F, Deng C, Chen L, et al. (2015) Transient performance improvement of microgrid by a resistive superconducting fault current limiter. IEEE Trans Appl Supercond 25: 1-5. |
[12] | He H, Chen L, Yin T, et al. (2016) Application of a SFCL for fault ride-through capability enhancement of DG in a microgrid system and relay protection coordination. IEEE Trans Appl Supercond 26: 1-8. |
[13] | Yehia DM, Mansour D-EA, Yuan W (2018) Fault ride-through enhancement of PMSG wind turbines with DC microgrids using resistive-type SFCL. IEEE Trans Appl Supercond 28: 1-5. |
[14] | Chen L, Chen H, Yang J, et al. (2017) Comparison of superconducting fault current limiter and dynamic voltage restorer for LVRT improvement of high penetration microgrid. IEEE Trans Appl Supercond 27: 1-7. |
[15] | Choi D-H, Yoo J-I, Kim D, et al. (2017) Analysis on effect of SFCL applied to an isolated microgrid with a dynamic load model. IEEE Trans Appl Supercond 27: 1-4. |
[16] | Chen L, Chen H, Li G, et al. (2018) Coordination of SMES, SFCL and distributed generation units for micro-grid stability enhancement via wireless communications. IEEE Access 6: 36699-36710. |
[17] | Khan UA, Seong JK, Lee SH, et al. (2010) Feasibility analysis of the positioning of superconducting fault current limiters for the smart grid application using simulink and simpowersystem. IEEE Trans Appl Supercond 21: 2165-2169. |
[18] | Khan UA, Hwang JS, Seong JK, et al. (2011) Application and positioning analysis of a resistive type Superconducting Fault Current Limiter in AC and DC microgrids using Simulink and SimPowerSystem. 2011 1st International Conference on Electric Power Equipment-Switching Technology 348-351. |
[19] | Jae-Sang H, Khan UA, Woo-Ju Shin, et al. (2012) Validity analysis on the positioning of superconducting fault current limiter in neighboring AC and DC microgrid. IEEE Trans Appl Supercond 23: 5600204. |
[20] | Srilatha S, Venkata RK (2012) Analysis of positioning of superconducting fault current limiter in smart grid application. Int J Adv Innovative Res 24-31. |
[21] | Zhang X, Ruiz HS, Zhong Z, et al. (2015) Implementation of resistive type superconducting fault current limiters in electrical grids, performance analysis and measuring of optimal locations. arXiv preprint 1508: 01162. |
[22] | Zhang X, Ruiz HS, Geng J, et al. (2017) Optimal location and minimum number of superconducting fault current limiters for the protection of power grids. Int J Electr Power Energy Syst 87: 136-143. |
[23] | Shirai Y, Taguchi M, Shiotsu M, et al. (2003) Simulation study on operating characteristics of superconducting fault current limiter in one machine infinite bus power system. IEEE Trans Appl Supercond 13: 1822-1827. |
[24] | Vilhena N, Arsenio P, Murta-Pina J, et al. (2014) Development of a simulink model of a saturated cores superconducting fault current limiter. Doctoral Conference on Computing, Electrical and Industrial Systems 415-422. |
[25] | Vilhena N, Arsenio P, Murta-Pina J, et al. (2014) A methodology for modeling and simulation of saturated cores fault current limiters. IEEE Trans Appl Supercond 25: 1-4. |
1. | Maryam Farsi, John Ahmet Erkoyuncu, An agent-based approach to quantify the uncertainty in Product-Service System contract decisions: A case study in the machine tool industry, 2021, 233, 09255273, 108014, 10.1016/j.ijpe.2020.108014 | |
2. | Camila Kolling, Janine Fleith de Medeiros, José Luis Duarte Ribeiro, Donato Morea, A conceptual model to support sustainable Product-Service System implementation in the Brazilian agricultural machinery industry, 2022, 355, 09596526, 131733, 10.1016/j.jclepro.2022.131733 | |
3. | Chunlong Wu, Hanyu Lv, 2023, Research on the Construction Method of Digital Twin Functional Model for Intelligent Product Service Systems, 979-8-3503-4378-6, 1, 10.1109/ICDS59539.2023.10311460 | |
4. | Alejandra García Lechuga, Guillermo Cortés Robles, Karina Cecilia Arredondo Soto, Marco Augusto Miranda Ackerman, The integration of the business model canvas and the service blueprinting to assist the conceptual design of new product-service systems, 2023, 415, 09596526, 137801, 10.1016/j.jclepro.2023.137801 | |
5. | Alejandra Garcia-Lechuga, Marco A. Miranda-Ackerman, Karina Arredondo-Soto, Mayra Corona-Andrade, 2024, Chapter 19, 978-3-031-54484-2, 427, 10.1007/978-3-031-54485-9_19 |
Questions | Answers |
Positive questions: If you can customize the ** module of PSS, how do you feel about it? | Very satisfied, satisfied, neutral, acceptable, not satisfied |
Negative questions: If you cannot customize the ** module of PSS, how do you feel about it? |
Decision-making needs of modules and attributes | Negative questions | |||||
Very satisfied | Satisfied | Neutral | Acceptable | Not satisfied | ||
Positive questions | Very satisfied | D | E | E | E | A |
Satisfied | P | I | I | I | B | |
Neutral | P | I | I | I | B | |
Acceptable | P | I | I | I | B | |
Not satisfied | P | P | P | P | D |
Modules | Basic needs (B) | Performance needs (A) | Excitement needs (E) | Neutral results (I) | Opposite results (P) | Confusion results (D) |
F1 | 35 | |||||
F2 | 35 | |||||
F3 | 35 | |||||
F4 | 35 | |||||
F5 | 35 | |||||
F6 | 35 | |||||
F7 | 5 | 30 | ||||
F8 | 5 | 30 | ||||
F9 | 5 | 30 | ||||
F10 | 5 | 30 | ||||
F11 | 5 | 30 | ||||
F12 | 5 | 30 | ||||
F13 | 8 | 25 | 2 | |||
F14 | 8 | 25 | 2 | |||
F15 | 8 | 25 | 2 | |||
F16 | 8 | 25 | 2 | |||
F17 | 8 | 25 | 2 | |||
F18 | 8 | 25 | 2 | |||
F19 | 25 | 10 | ||||
F20 | 25 | 10 | ||||
F21 | 25 | 10 | ||||
F22 | 25 | 10 | ||||
F23 | 35 | |||||
F24 | 10 | 22 | 3 | |||
F25 | 25 | 10 | ||||
F26 | 10 | 20 | 5 | |||
F27 | 5 | 10 | 20 | |||
F28 | 30 | 5 |
Modules | Basic needs (B) | Performance needs (A) | Excitement needs (E) | Neutral results (I) | Opposite results (P) | Confusion results (D) |
F1 | 15 | |||||
F2 | 15 | |||||
F3 | 15 | |||||
F4 | 15 | |||||
F5 | 15 | |||||
F6 | 15 | |||||
F7 | 15 | |||||
F8 | 15 | |||||
F9 | 15 | |||||
F10 | 15 | |||||
F11 | 15 | |||||
F12 | 5 | 10 | ||||
F13 | 3 | 10 | 2 | |||
F14 | 3 | 10 | 2 | |||
F15 | 3 | 10 | 2 | |||
F16 | 3 | 10 | 2 | |||
F17 | 3 | 10 | 2 | |||
F18 | 3 | 10 | 2 | |||
F19 | 3 | 10 | 2 | |||
F20 | 3 | 10 | 2 | |||
F21 | 3 | 10 | 2 | |||
F23 | 13 | 2 | ||||
F24 | 5 | 10 | ||||
F25 | 5 | 10 | ||||
F26 | 1 | 5 | 9 | |||
F28 | 10 | 5 |
Modules | Basic needs (B) | Performance needs (A) | Excitement needs (E) | Neutral results (I) | Opposite results (P) | Confusion results (D) |
F1 | 2 | 6 | 3 | |||
F2 | 10 | |||||
F3 | 10 | |||||
F4 | 10 | |||||
F5 | 10 | |||||
F6 | 10 | |||||
F7 | 8 | 2 | ||||
F8 | 8 | 2 | ||||
F9 | 8 | 2 | ||||
F10 | 8 | 2 | ||||
F11 | 8 | 2 | ||||
F12 | 7 | 3 | ||||
F13 | 6 | 2 | 2 | |||
F14 | 6 | 2 | 2 | |||
F15 | 6 | 2 | 2 | |||
F16 | 6 | 2 | 2 | |||
F17 | 6 | 2 | 2 | |||
F18 | 6 | 2 | 2 | |||
F19 | 7 | 2 | 1 | |||
F20 | 7 | 2 | 1 | |||
F21 | 7 | 2 | 1 | |||
F23 | 8 | 2 | ||||
F24 | 9 | 1 | ||||
F26 | 8 | 2 | ||||
F28 | 9 | 1 |
![]() |
MI1 | MI2 | MI3 | ||
N1 | Transforming service | Purchase transformer | S9 | S10 | S11 |
Lease transformer | S9 | S10 | S11 | ||
Purchase electric power | S9 | S10 | S11 | ||
N2 | Basic auxiliary modules of the transformer | Wire box and control cable | JC1 | JC2 | |
Cooling unit | |||||
Temperature controller | |||||
Butterfly valve | |||||
Oil storage tank | |||||
N3 | High pressure bushing | COT-550/800 | COT-551/800 | COT-552/800 | |
N4 | Low pressure bushing | BD-20/3150 | BD-21/3151 | BD-22/3152 | |
N5 | Online filter oil machine | R1 | R2 | ||
N6 | Gas relay | BF-80/10 | BF-88/10 | ||
N7 | Pressure relief valve | YSF8-55/130KJ | YSF8-56/130KJ | ||
N8 | High and low voltage switch control cabinet | K1 | K2 | K3 | |
N9 | Monitoring services | Chromatography monitoring | iMGA2020 | iMGA2021 | |
N10 | Partial discharge monitoring | iPDM2020T | iPDM2021T | ||
N11 | Casing insulation monitoring | iIMM2020 | iIMM2021 | ||
N12 | Core monitoring | iOCM2020 | iOCM2021 | ||
N13 | Winding temperature fiber monitoring | iOFT2020 | iOFT2021 | ||
N14 | Cooling unit monitoring | iCSM2020 | iCSM2021 | ||
N15 | Testing services | Routine testing | Routine testing | ||
N16 | Typical testing | Typical testing | |||
N17 | Special testing | Special testing | |||
N18 | Financial services | Manufacturer loan | The third party loan | Bank installment loan | |
N19 | Transportation services | The third party transporting | Seller transporting | Customer transporting | |
N20 | Installation services | The third party installation | Seller installation | ||
N21 | Maintenance services | Warranty maintenance services | One-year warranty | Two-year warranty | Three-year warranty |
N22 | Non-warranty maintenance services | Routine maintenance | Full services | ||
N23 | Recycling services | Old for new | Full recovery | Non-recovery | |
N24 | Spare parts | SP1 | SP2 |
![]() |
MI1 | MI2 | MI3 | MI4 | |||
N1 | Transforming service | Purchase transformer | S9 | S10 | S11 | ||
Lease transformer | S9 | S10 | S11 | ||||
Purchase electric power | S9 | S10 | S11 | ||||
N2 | Basic auxiliary modules of the transformer | JC1 | JC2 | ||||
N3 | High pressure bushing | COT-550/800 | COT-551/800 | COT-552/800 | |||
N4 | Low pressure bushing | BD-20/3150 | BD-21/3151 | BD-22/3152 | |||
N5 | Online filter oil machine | R1 | R2 | ||||
N6 | Gas relay | BF-80/10 | BF-88/10 | ||||
N7 | Pressure relief valve | YSF8-55/130KJ | YSF8-56/130KJ | ||||
N8 | High and low voltage switch control cabinet | K1 | K2 | K3 | Not supply | ||
N9 | Monitoring services | Chromatography monitoring IED component | iMGA2020 | iMGA2021 | Not monitoring | ||
N10 | Partial discharge monitoring IED component | iPDM2020T | iPDM2021T | Not monitoring | |||
N11 | Casing insulation monitoring IED component | iIMM2020 | iIMM2021 | Not monitoring | |||
N12 | Core monitoring component | iOCM2020 | iOCM2021 | Not monitoring | |||
N13 | Winding temperature fiber monitoring IED component | iOFT2020 | iOFT2021 | Not monitoring | |||
N14 | Cooling unit monitoring IED | iCSM2020 | iCSM2021 | Not monitoring | |||
N15 | Testing services | Routine testing | Routine testing | Not testing | |||
N16 | Testing services Routine testing Typical testing Special testing |
Typical testing | Typical testing | Not testing | |||
N17 | Testing services Routine testing Typical testing Special testing |
Special testing | Special testing | Not testing | |||
N18 | Financial services | Manufacturer loan | The third party loan | Bank installment loan | Not loan | ||
N19 | Transportation service s | The third party transporting | Seller transporting | Customer transporting | |||
N20 | Installation services | The third party installation | Seller installation | ||||
N21 | Maintenance services | Warranty maintenance services | One-year warranty | Two-year warranty | Three-year warranty | ||
N22 | Non-warranty maintenance services | Routine maintenance | Full services | ||||
N23 | Recycling services | Old for new | Full recovery | Non-recovery | |||
N24 | Spare parts | SP1 | SP2 |
![]() |
S1 | S2 | S3 | S4 | ![]() |
S1 | S2 | S3 | S4 |
N1 | E11 | E12 | E13 | N13 | E131 | E132 | E133 | ||
N2 | E21 | E22 | N14 | E141 | E142 | E143 | |||
N3 | E31 | E32 | E33 | N15 | E151 | E152 | |||
N4 | E41 | E42 | E43 | N16 | E161 | E162 | |||
N5 | E51 | E52 | N17 | E171 | E172 | ||||
N6 | E61 | E62 | N18 | E181 | E182 | E183 | E184 | ||
N7 | E71 | E72 | N19 | E191 | E192 | E193 | |||
N8 | E81 | E82 | E83 | E84 | N20 | E201 | E202 | ||
N9 | E91 | E92 | E93 | N21 | E211 | E212 | E213 | ||
N10 | E101 | E102 | E103 | N22 | E221 | E222 | |||
N11 | E111 | E112 | E113 | N23 | E231 | E232 | E231 | ||
N12 | E121 | E122 | E123 | N24 | E241 | E242 |
Instances Module classes |
S1 | S2 | S3 | S4 | Instances Module classes |
S1 | S2 | S3 | S4 |
N1 | E11 | E12 | E13 | N13 | E131 | E132 | E133 | ||
N2 | E21 | E22 | N14 | E141 | E142 | E143 | |||
N3 | E31 | E32 | E33 | N15 | E151 | E152 | |||
N4 | E41 | E42 | E43 | N16 | E161 | E162 | |||
N5 | E51 | E52 | N17 | E171 | E172 | ||||
N6 | E61 | E62 | N18 | E181 | E182 | E183 | E184 | ||
N7 | E71 | E72 | N19 | E191 | E192 | E193 | |||
N8 | E81 | E82 | E83 | E84 | N20 | E201 | E202 | ||
N9 | E91 | E92 | E93 | N21 | E211 | E212 | E213 | ||
N10 | E101 | E102 | E103 | N22 | E221 | E222 | |||
N11 | E111 | E112 | E113 | N23 | E231 | E232 | E231 | ||
N12 | E121 | E122 | E123 | N24 | E241 | E242 |
![]() |
S1 | S2 | S3 | S4 | ![]() |
S1 | S2 | S3 | S4 |
N1 | E11 | E12 | E13 | N12 | E121 | E122 | E123 | ||
N2 | E21 | E22 | N13 | E131 | E132 | E133 | |||
N3 | E31 | E32 | E33 | N14 | E141 | E142 | E143 | ||
N4 | E41 | E42 | E43 | N15 | E151 | E152 | |||
N5 | E51 | E52 | N16 | E161 | E162 | ||||
N6 | E61 | E62 | N17 | E171 | E172 | ||||
N7 | E71 | E72 | N19 | E191 | E192 | E193 | |||
N8 | E81 | E82 | E83 | E84 | N20 | E201 | E202 | ||
N9 | E91 | E92 | E93 | N21 | E211 | E212 | E213 | ||
N10 | E101 | E102 | E103 | N22 | E221 | E222 | |||
N11 | E111 | E112 | E113 | N24 | E241 | E242 |
Instances Module classes |
S1 | S2 | S3 | Instances Module classes |
S1 | S2 | S3 |
N1 | E11 | E12 | E13 | N12 | E121 | E122 | |
N2 | E21 | E22 | N13 | E131 | E132 | ||
N3 | E31 | E32 | E33 | N14 | E141 | E142 | |
N4 | E41 | E42 | E43 | N15 | E151 | E152 | |
N5 | E51 | E52 | N16 | E161 | E162 | ||
N6 | E61 | E62 | N17 | E171 | E172 | ||
N7 | E71 | E72 | N19 | E191 | E192 | E193 | |
N8 | E81 | E82 | E83 | N20 | E201 | E202 | |
N9 | E91 | E92 | N22 | E221 | E222 | ||
N10 | E101 | E102 | N24 | E241 | E242 | ||
N11 | E111 | E112 |
Not buy | 1 | 2 | 3 | 4 | 5 | 6 | 7 | 8 | 9 | Buy |
Card No. | Buy transformer ontology y | Basic auxiliary modules | High pressure bushing | Low pressure bushing | Online filter oil machine | Gas relay | Pressure relief valve | High and low voltage switch control cabinet | … | Spare parts | Scoring 1 | Scoring 2 | Scoring 3 | Scoring 4 | … | Scoring 34 | Scoring 35 |
1 | E11 | E21 | E32 | E41 | E51 | E62 | E72 | E82 | … | E242 | 6 | 7 | 5 | 5 | … | 5 | 4 |
2 | E12 | E21 | E31 | E42 | E51 | E62 | E72 | E84 | … | E242 | 7 | 8 | 7 | 8 | … | 4 | 6 |
3 | E11 | E21 | E32 | E41 | E51 | E61 | E72 | E82 | … | E242 | 5 | 6 | 6 | 6 | … | 6 | 5 |
4 | E11 | E21 | E32 | E41 | E51 | E62 | E72 | E82 | … | E242 | 7 | 7 | 7 | 8 | … | 5 | 6 |
5 | E11 | E21 | E32 | E43 | E52 | E61 | E71 | E82 | … | E241 | 8 | 6 | 8 | 8 | … | 7 | 6 |
6 | E12 | E21 | E31 | E43 | E51 | E61 | E72 | E82 | … | E242 | 6 | 6 | 5 | 5 | … | 6 | 6 |
7 | E12 | E21 | E32 | E42 | E52 | E62 | E71 | E83 | … | E241 | 4 | 5 | 3 | 3 | … | 5 | 2 |
8 | E11 | E21 | E32 | E41 | E52 | E62 | E72 | E83 | … | E241 | 5 | 5 | 6 | 6 | … | 4 | 1 |
9 | E11 | E21 | E33 | E43 | E52 | E62 | E72 | E84 | … | E242 | 8 | 9 | 7 | 7 | … | 6 | 8 |
10 | E12 | E21 | E31 | E42 | E51 | E61 | E71 | E82 | … | E242 | 6 | 6 | 7 | 5 | … | 5 | 6 |
11 | E11 | E21 | E32 | E43 | E51 | E61 | E71 | E83 | … | E242 | 4 | 3 | 5 | 5 | … | 5 | 5 |
12 | E11 | E21 | E31 | E43 | E52 | E62 | E71 | E82 | … | E242 | 5 | 3 | 5 | 6 | … | 6 | 4 |
13 | E13 | E21 | E32 | E41 | E51 | E62 | E72 | E82 | … | E242 | 6 | 6 | 7 | 5 | … | 5 | 6 |
… | … | … | … | … | … | … | … | … | … | … | … | … | … | … | … | … | … |
63 | E13 | E21 | E31 | E43 | E52 | E61 | E72 | E84 | … | E242 | 4 | 5 | 5 | 5 | … | 4 | 4 |
64 | E11 | E21 | E32 | E41 | E52 | E62 | E71 | E84 | … | E242 | 6 | 7 | 5 | 5 | … | 6 | 6 |
Modules | The utility | Standard error | The importance degree | Modules | The utility | Standard error | The importance degree | ||
Buy transformer | S9 | 0.127 | 0.13 | 1.976 | Winding temperature fiber monitoring | iOFT2020 | −0.086 | 0.13 | 4.703 |
S10 | −0.069 | 0.152 | iOFT2021 | 0.233 | 0.152 | ||||
S11 | −0.058 | 0.152 | Not monitoring | −0.147 | 0.152 | ||||
Basic auxiliary modules of the transformer | JC1 | 0.047 | 0.097 | 1.643 | Cooling unit monitoring | iCSM2020 | 0.196 | 0.13 | 6.094 |
JC2 | −0.047 | 0.097 | iCSM2021 | 0.111 | 0.152 | ||||
High pressure bushing | COT-550/800 | 0.028 | 0.13 | 4.738 | Not monitoring | −0.307 | 0.152 | ||
COT-551/800 | 0.046 | 0.152 | Routine testing | Routine testing | 0.173 | 0.097 | 4.317 | ||
COT-552/800 | −0.074 | 0.152 | Not testing | −0.173 | 0.097 | ||||
Low pressure bushing | BD-20/3150 | −0.243 | 0.13 | 6.611 | Typical testing | Typical testing | 0.162 | 0.097 | 4.028 |
BD-21/3151 | 0.433 | 0.152 | Not testing | −0.162 | 0.097 | ||||
BD-22/3152 | −0.19 | 0.152 | Special testing | Special testing | 0.358 | 0.097 | 6.469 | ||
Online filter oil machine | R1 | 0.066 | 0.097 | 4.63 | Not testing | −0.358 | 0.097 | ||
R2 | −0.066 | 0.097 | Financial services | Manufacturer loan | 0.085 | 0.168 | 4.774 | ||
Gas relay | BF-80/10 | −0.01 | 0.097 | 2.335 | The third party loan | 0.16 | 0.168 | ||
BF-88/10 | 0.01 | 0.097 | Bank installment loan | −0.15 | 0.168 | ||||
Pressure relief valve | YSF8-55/130KJ | 0.017 | 0.097 | 2.684 | Not loan | −0.095 | 0.168 | ||
YSF8-56/130KJ | −0.017 | 0.097 | Transportation services | The third party transporting | −0.186 | 0.13 | 4.666 | ||
High and low voltage switch control cabinet | K1 | −0.388 | 0.168 | 5.314 | Seller transporting | 0.021 | 0.152 | ||
K2 | 0.271 | 0.168 | Customer transporting | 0.165 | 0.152 | ||||
K3 | 0.169 | 0.168 | Installation services | The third party installation | −0.06 | 0.097 | 3.485 | ||
Not provided | −0.052 | 0.168 | Seller installation | 0.06 | 0.097 | ||||
Chromatography monitoring | iMGA2020 | −0.157 | 0.13 | 5.241 | Warranty maintenance services | One-year warranty | 0.185 | 0.13 | 5.039 |
iMGA2021 | 0.114 | 0.152 | Two-year warranty | −0.236 | 0.152 | ||||
Not monitoring | 0.043 | 0.152 | Three-year warranty | 0.051 | 0.152 | ||||
Partial discharge monitoring | iPDM2020T | −0.216 | 0.13 | 4.778 | Non-warranty maintenance services | Routine maintenance | 0.233 | 0.097 | 4.272 |
iPDM2021T | 0.028 | 0.152 | Full services | −0.233 | 0.097 | ||||
Not monitoring | 0.188 | 0.152 | Recycling services | Old for new | 0.017 | 0.13 | 1.555 | ||
Casing insulation monitoring | iIMM2020 | −0.042 | 0.13 | 4.468 | Full recovery | 0.4 | 0.152 | ||
iIMM2021 | 0.016 | 0.152 | Non-recovery | −0.418 | 0.152 | ||||
Not monitoring | 0.026 | 0.152 | Spare parts | SP1 | 0.125 | 0.097 | 1.425 | ||
Core monitoring | iOCM2020 | 0.051 | 0.13 | 4.755 | SP2 | −0.125 | 0.097 | ||
iOCM2021 | 0.155 | 0.152 | |||||||
Not monitoring | −0.206 | 0.152 | |||||||
(constant) | 5.75 | 0.148 | (constant) | 5.75 | 0.148 |
Modules | The utility | Standard error | The importance degree | Modules | The utility | Standard error | The importance degree | ||
Lease transformer | S9 | −0.206 | 0.261 | 2.062 | Core monitoring | iOCM2020 | 0.108 | 0.261 | 6.721 |
S10 | −0.51 | 0.307 | iOCM2021 | 0.31 | 0.307 | ||||
S11 | 0.715 | 0.307 | Not monitoring | −0.419 | 0.307 | ||||
Basic auxiliary modules of the transformer | JC1 | −0.077 | 0.196 | 2.173 | Winding temperature fiber monitoring | iOFT2020 | 0.114 | 0.261 | 3.857 |
JC2 | 0.077 | 0.196 | iOFT2021 | −0.205 | 0.307 | ||||
High pressure bushing | COT-550/800 | −0.175 | 0.261 | 5.197 | Not monitoring | 0.091 | 0.307 | ||
COT-551/800 | 0.327 | 0.307 | Cooling unit monitoring | iCSM2020 | −0.05 | 0.261 | 3.28 | ||
COT-552/800 | −0.152 | 0.307 | iCSM2021 | −0.058 | 0.307 | ||||
Low pressure bushing | BD-20/3150 | −0.481 | 0.261 | 7.479 | Not monitoring | 0.108 | 0.307 | ||
BD-21/3151 | 0.317 | 0.307 | Routine testing | Routine testing | −0.081 | 0.196 | 4.904 | ||
BD-22/3152 | 0.163 | 0.307 | Not testing | 0.081 | 0.196 | ||||
Online filter oil machine | R1 | −0.085 | 0.196 | 2.263 | Typical testing | Typical testing | −0.281 | 0.196 | 4.85 |
R2 | 0.085 | 0.196 | Not testing | 0.281 | 0.196 | ||||
Gas relay | BF-80/10 | −0.013 | 0.196 | 2.167 | Special testing | Special testing | 0.027 | 0.196 | 4.971 |
BF-88/10 | 0.013 | 0.196 | Not testing | −0.027 | 0.196 | ||||
Pressure relief valve | YSF8-55/130KJ | −0.142 | 0.196 | 2.771 | Transportation services | The third party transporting | 0.133 | 0.261 | 3.362 |
YSF8-56/130KJ | 0.142 | 0.196 | Seller transporting | −0.079 | 0.307 | ||||
High and low voltage switch control cabinet | K1 | 0.25 | 0.34 | 7.549 | Customer transporting | −0.054 | 0.307 | ||
K2 | −0.4 | 0.34 | Installation services | The third party installation | 0.044 | 0.196 | 4.592 | ||
K3 | 0.142 | 0.34 | Seller installation | −0.044 | 0.196 | ||||
Not providing | 0.008 | 0.34 | Maintenance services | One-year warranty | −0.292 | 0.261 | 6.278 | ||
Chromatography monitoring | iMGA2020 | −0.178 | 0.261 | 4.384 | Two-year warranty | 0.31 | 0.307 | ||
iMGA2021 | −0.09 | 0.307 | Three-year warranty | −0.019 | 0.307 | ||||
Not monitoring | 0.268 | 0.307 | Non-warranty maintenance services | Routine maintenance | 0.127 | 0.196 | 2.935 | ||
Partial discharge monitoring | iPDM2020T | 0.169 | 0.261 | 8.459 | Full services | −0.127 | 0.196 | ||
iPDM2021T | −0.541 | 0.307 | Spare parts | SP1 | −0.004 | 0.196 | 2.313 | ||
Not monitoring | 0.372 | 0.307 | SP2 | 0.004 | 0.196 | ||||
Casing insulation monitoring | iIMM2020 | −0.044 | 0.261 | 7.433 | |||||
iIMM2021 | −0.374 | 0.307 | |||||||
Not monitoring | 0.418 | 0.307 | |||||||
(constant) | 5.587 | 0.292 | (constant) | 5.587 | 0.292 |
Modules | The utility | Standard error | The importance degree | Modules | The utility | Standard error | The importance degree | ||
Purchase electric power | S9 | −0.16 | 0.16 | 11.606 | Casing insulation monitoring | iIMM2020 | −0.048 | 0.12 | 5.825 |
S10 | 0.068 | 0.188 | iIMM2021 | 0.048 | 0.12 | ||||
S11 | 0.093 | 0.188 | Core monitoring | iOCM2020 | 0.183 | 0.12 | 4.038 | ||
Basic auxiliary modules of the transformer | JC1 | 0.114 | 0.12 | 3.013 | iOCM2021 | −0.183 | 0.12 | ||
JC2 | −0.114 | 0.12 | Winding temperature fiber monitoring | iOFT2020 | 0.117 | 0.12 | 3.555 | ||
High pressure bushing | COT-550/800 | −0.198 | 0.16 | 5.665 | iOFT2021 | −0.117 | 0.12 | ||
COT-551/800 | 0.252 | 0.188 | Cooling unit monitoring | iCSM2020 | −0.089 | 0.12 | 4.352 | ||
COT-552/800 | −0.054 | 0.188 | iCSM2021 | 0.089 | 0.12 | ||||
Low pressure bushing | BD-20/3150 | −0.035 | 0.16 | 5.875 | Routine testing | Routine testing | −0.011 | 0.12 | 4.021 |
BD-21/3151 | 0.099 | 0.188 | Not testing | 0.011 | 0.12 | ||||
BD-22/3152 | −0.064 | 0.188 | Typical testing | Typical testing | 0.008 | 0.12 | 4.636 | ||
Online filter oil machine | R1 | 0.123 | 0.12 | 4.376 | Not testing | −0.008 | 0.12 | ||
R2 | −0.123 | 0.12 | Special testing | Special testing | 0.205 | 0.12 | 4.405 | ||
Gas relay | BF-80/10 | −0.092 | 0.12 | 5.005 | Not testing | −0.205 | 0.12 | ||
BF-88/10 | 0.092 | 0.12 | Transportation services | The third party transporting | 0.352 | 0.16 | 4.170 | ||
Pressure relief valve | YSF8-55/130KJ | 0.142 | 0.12 | 4.986 | Seller transporting | −0.31 | 0.188 | ||
YSF8-56/130KJ | −0.142 | 0.12 | Customer transporting | −0.042 | 0.188 | ||||
High and low voltage switch control cabinet | K1 | 0.458 | 0.208 | 4.098 | Installation services | The third party installation | −0.027 | 0.12 | 3.521 |
K2 | −0.405 | 0.208 | Seller installation | 0.027 | 0.12 | ||||
K3 | −0.211 | 0.208 | Non-warranty maintenance services | S1 | −0.014 | 0.12 | 3.801 | ||
Not providing | 0.158 | 0.208 | S2 | 0.014 | 0.12 | ||||
Chromatography monitoring | iMGA2020 | 0.002 | 0.12 | 4.412 | Spare parts | SP1 | 0.18 | 0.12 | 4.248 |
iMGA2021 | −0.002 | 0.12 | SP2 | −0.18 | 0.12 | ||||
Partial discharge monitoring | iPDM2020T | −0.202 | 0.12 | 4.392 | |||||
iPDM2021T | 0.202 | 0.12 | |||||||
(constant) | 5.621 | 0.144 |
Questions | Answers |
Positive questions: If you can customize the ** module of PSS, how do you feel about it? | Very satisfied, satisfied, neutral, acceptable, not satisfied |
Negative questions: If you cannot customize the ** module of PSS, how do you feel about it? |
Decision-making needs of modules and attributes | Negative questions | |||||
Very satisfied | Satisfied | Neutral | Acceptable | Not satisfied | ||
Positive questions | Very satisfied | D | E | E | E | A |
Satisfied | P | I | I | I | B | |
Neutral | P | I | I | I | B | |
Acceptable | P | I | I | I | B | |
Not satisfied | P | P | P | P | D |
Modules | Basic needs (B) | Performance needs (A) | Excitement needs (E) | Neutral results (I) | Opposite results (P) | Confusion results (D) |
F1 | 35 | |||||
F2 | 35 | |||||
F3 | 35 | |||||
F4 | 35 | |||||
F5 | 35 | |||||
F6 | 35 | |||||
F7 | 5 | 30 | ||||
F8 | 5 | 30 | ||||
F9 | 5 | 30 | ||||
F10 | 5 | 30 | ||||
F11 | 5 | 30 | ||||
F12 | 5 | 30 | ||||
F13 | 8 | 25 | 2 | |||
F14 | 8 | 25 | 2 | |||
F15 | 8 | 25 | 2 | |||
F16 | 8 | 25 | 2 | |||
F17 | 8 | 25 | 2 | |||
F18 | 8 | 25 | 2 | |||
F19 | 25 | 10 | ||||
F20 | 25 | 10 | ||||
F21 | 25 | 10 | ||||
F22 | 25 | 10 | ||||
F23 | 35 | |||||
F24 | 10 | 22 | 3 | |||
F25 | 25 | 10 | ||||
F26 | 10 | 20 | 5 | |||
F27 | 5 | 10 | 20 | |||
F28 | 30 | 5 |
Modules | Basic needs (B) | Performance needs (A) | Excitement needs (E) | Neutral results (I) | Opposite results (P) | Confusion results (D) |
F1 | 15 | |||||
F2 | 15 | |||||
F3 | 15 | |||||
F4 | 15 | |||||
F5 | 15 | |||||
F6 | 15 | |||||
F7 | 15 | |||||
F8 | 15 | |||||
F9 | 15 | |||||
F10 | 15 | |||||
F11 | 15 | |||||
F12 | 5 | 10 | ||||
F13 | 3 | 10 | 2 | |||
F14 | 3 | 10 | 2 | |||
F15 | 3 | 10 | 2 | |||
F16 | 3 | 10 | 2 | |||
F17 | 3 | 10 | 2 | |||
F18 | 3 | 10 | 2 | |||
F19 | 3 | 10 | 2 | |||
F20 | 3 | 10 | 2 | |||
F21 | 3 | 10 | 2 | |||
F23 | 13 | 2 | ||||
F24 | 5 | 10 | ||||
F25 | 5 | 10 | ||||
F26 | 1 | 5 | 9 | |||
F28 | 10 | 5 |
Modules | Basic needs (B) | Performance needs (A) | Excitement needs (E) | Neutral results (I) | Opposite results (P) | Confusion results (D) |
F1 | 2 | 6 | 3 | |||
F2 | 10 | |||||
F3 | 10 | |||||
F4 | 10 | |||||
F5 | 10 | |||||
F6 | 10 | |||||
F7 | 8 | 2 | ||||
F8 | 8 | 2 | ||||
F9 | 8 | 2 | ||||
F10 | 8 | 2 | ||||
F11 | 8 | 2 | ||||
F12 | 7 | 3 | ||||
F13 | 6 | 2 | 2 | |||
F14 | 6 | 2 | 2 | |||
F15 | 6 | 2 | 2 | |||
F16 | 6 | 2 | 2 | |||
F17 | 6 | 2 | 2 | |||
F18 | 6 | 2 | 2 | |||
F19 | 7 | 2 | 1 | |||
F20 | 7 | 2 | 1 | |||
F21 | 7 | 2 | 1 | |||
F23 | 8 | 2 | ||||
F24 | 9 | 1 | ||||
F26 | 8 | 2 | ||||
F28 | 9 | 1 |
![]() |
MI1 | MI2 | MI3 | ||
N1 | Transforming service | Purchase transformer | S9 | S10 | S11 |
Lease transformer | S9 | S10 | S11 | ||
Purchase electric power | S9 | S10 | S11 | ||
N2 | Basic auxiliary modules of the transformer | Wire box and control cable | JC1 | JC2 | |
Cooling unit | |||||
Temperature controller | |||||
Butterfly valve | |||||
Oil storage tank | |||||
N3 | High pressure bushing | COT-550/800 | COT-551/800 | COT-552/800 | |
N4 | Low pressure bushing | BD-20/3150 | BD-21/3151 | BD-22/3152 | |
N5 | Online filter oil machine | R1 | R2 | ||
N6 | Gas relay | BF-80/10 | BF-88/10 | ||
N7 | Pressure relief valve | YSF8-55/130KJ | YSF8-56/130KJ | ||
N8 | High and low voltage switch control cabinet | K1 | K2 | K3 | |
N9 | Monitoring services | Chromatography monitoring | iMGA2020 | iMGA2021 | |
N10 | Partial discharge monitoring | iPDM2020T | iPDM2021T | ||
N11 | Casing insulation monitoring | iIMM2020 | iIMM2021 | ||
N12 | Core monitoring | iOCM2020 | iOCM2021 | ||
N13 | Winding temperature fiber monitoring | iOFT2020 | iOFT2021 | ||
N14 | Cooling unit monitoring | iCSM2020 | iCSM2021 | ||
N15 | Testing services | Routine testing | Routine testing | ||
N16 | Typical testing | Typical testing | |||
N17 | Special testing | Special testing | |||
N18 | Financial services | Manufacturer loan | The third party loan | Bank installment loan | |
N19 | Transportation services | The third party transporting | Seller transporting | Customer transporting | |
N20 | Installation services | The third party installation | Seller installation | ||
N21 | Maintenance services | Warranty maintenance services | One-year warranty | Two-year warranty | Three-year warranty |
N22 | Non-warranty maintenance services | Routine maintenance | Full services | ||
N23 | Recycling services | Old for new | Full recovery | Non-recovery | |
N24 | Spare parts | SP1 | SP2 |
![]() |
MI1 | MI2 | MI3 | MI4 | |||
N1 | Transforming service | Purchase transformer | S9 | S10 | S11 | ||
Lease transformer | S9 | S10 | S11 | ||||
Purchase electric power | S9 | S10 | S11 | ||||
N2 | Basic auxiliary modules of the transformer | JC1 | JC2 | ||||
N3 | High pressure bushing | COT-550/800 | COT-551/800 | COT-552/800 | |||
N4 | Low pressure bushing | BD-20/3150 | BD-21/3151 | BD-22/3152 | |||
N5 | Online filter oil machine | R1 | R2 | ||||
N6 | Gas relay | BF-80/10 | BF-88/10 | ||||
N7 | Pressure relief valve | YSF8-55/130KJ | YSF8-56/130KJ | ||||
N8 | High and low voltage switch control cabinet | K1 | K2 | K3 | Not supply | ||
N9 | Monitoring services | Chromatography monitoring IED component | iMGA2020 | iMGA2021 | Not monitoring | ||
N10 | Partial discharge monitoring IED component | iPDM2020T | iPDM2021T | Not monitoring | |||
N11 | Casing insulation monitoring IED component | iIMM2020 | iIMM2021 | Not monitoring | |||
N12 | Core monitoring component | iOCM2020 | iOCM2021 | Not monitoring | |||
N13 | Winding temperature fiber monitoring IED component | iOFT2020 | iOFT2021 | Not monitoring | |||
N14 | Cooling unit monitoring IED | iCSM2020 | iCSM2021 | Not monitoring | |||
N15 | Testing services | Routine testing | Routine testing | Not testing | |||
N16 | Testing services Routine testing Typical testing Special testing |
Typical testing | Typical testing | Not testing | |||
N17 | Testing services Routine testing Typical testing Special testing |
Special testing | Special testing | Not testing | |||
N18 | Financial services | Manufacturer loan | The third party loan | Bank installment loan | Not loan | ||
N19 | Transportation service s | The third party transporting | Seller transporting | Customer transporting | |||
N20 | Installation services | The third party installation | Seller installation | ||||
N21 | Maintenance services | Warranty maintenance services | One-year warranty | Two-year warranty | Three-year warranty | ||
N22 | Non-warranty maintenance services | Routine maintenance | Full services | ||||
N23 | Recycling services | Old for new | Full recovery | Non-recovery | |||
N24 | Spare parts | SP1 | SP2 |
![]() |
S1 | S2 | S3 | S4 | ![]() |
S1 | S2 | S3 | S4 |
N1 | E11 | E12 | E13 | N13 | E131 | E132 | E133 | ||
N2 | E21 | E22 | N14 | E141 | E142 | E143 | |||
N3 | E31 | E32 | E33 | N15 | E151 | E152 | |||
N4 | E41 | E42 | E43 | N16 | E161 | E162 | |||
N5 | E51 | E52 | N17 | E171 | E172 | ||||
N6 | E61 | E62 | N18 | E181 | E182 | E183 | E184 | ||
N7 | E71 | E72 | N19 | E191 | E192 | E193 | |||
N8 | E81 | E82 | E83 | E84 | N20 | E201 | E202 | ||
N9 | E91 | E92 | E93 | N21 | E211 | E212 | E213 | ||
N10 | E101 | E102 | E103 | N22 | E221 | E222 | |||
N11 | E111 | E112 | E113 | N23 | E231 | E232 | E231 | ||
N12 | E121 | E122 | E123 | N24 | E241 | E242 |
Instances Module classes |
S1 | S2 | S3 | S4 | Instances Module classes |
S1 | S2 | S3 | S4 |
N1 | E11 | E12 | E13 | N13 | E131 | E132 | E133 | ||
N2 | E21 | E22 | N14 | E141 | E142 | E143 | |||
N3 | E31 | E32 | E33 | N15 | E151 | E152 | |||
N4 | E41 | E42 | E43 | N16 | E161 | E162 | |||
N5 | E51 | E52 | N17 | E171 | E172 | ||||
N6 | E61 | E62 | N18 | E181 | E182 | E183 | E184 | ||
N7 | E71 | E72 | N19 | E191 | E192 | E193 | |||
N8 | E81 | E82 | E83 | E84 | N20 | E201 | E202 | ||
N9 | E91 | E92 | E93 | N21 | E211 | E212 | E213 | ||
N10 | E101 | E102 | E103 | N22 | E221 | E222 | |||
N11 | E111 | E112 | E113 | N23 | E231 | E232 | E231 | ||
N12 | E121 | E122 | E123 | N24 | E241 | E242 |
![]() |
S1 | S2 | S3 | S4 | ![]() |
S1 | S2 | S3 | S4 |
N1 | E11 | E12 | E13 | N12 | E121 | E122 | E123 | ||
N2 | E21 | E22 | N13 | E131 | E132 | E133 | |||
N3 | E31 | E32 | E33 | N14 | E141 | E142 | E143 | ||
N4 | E41 | E42 | E43 | N15 | E151 | E152 | |||
N5 | E51 | E52 | N16 | E161 | E162 | ||||
N6 | E61 | E62 | N17 | E171 | E172 | ||||
N7 | E71 | E72 | N19 | E191 | E192 | E193 | |||
N8 | E81 | E82 | E83 | E84 | N20 | E201 | E202 | ||
N9 | E91 | E92 | E93 | N21 | E211 | E212 | E213 | ||
N10 | E101 | E102 | E103 | N22 | E221 | E222 | |||
N11 | E111 | E112 | E113 | N24 | E241 | E242 |
Instances Module classes |
S1 | S2 | S3 | Instances Module classes |
S1 | S2 | S3 |
N1 | E11 | E12 | E13 | N12 | E121 | E122 | |
N2 | E21 | E22 | N13 | E131 | E132 | ||
N3 | E31 | E32 | E33 | N14 | E141 | E142 | |
N4 | E41 | E42 | E43 | N15 | E151 | E152 | |
N5 | E51 | E52 | N16 | E161 | E162 | ||
N6 | E61 | E62 | N17 | E171 | E172 | ||
N7 | E71 | E72 | N19 | E191 | E192 | E193 | |
N8 | E81 | E82 | E83 | N20 | E201 | E202 | |
N9 | E91 | E92 | N22 | E221 | E222 | ||
N10 | E101 | E102 | N24 | E241 | E242 | ||
N11 | E111 | E112 |
Not buy | 1 | 2 | 3 | 4 | 5 | 6 | 7 | 8 | 9 | Buy |
Card No. | Buy transformer ontology y | Basic auxiliary modules | High pressure bushing | Low pressure bushing | Online filter oil machine | Gas relay | Pressure relief valve | High and low voltage switch control cabinet | … | Spare parts | Scoring 1 | Scoring 2 | Scoring 3 | Scoring 4 | … | Scoring 34 | Scoring 35 |
1 | E11 | E21 | E32 | E41 | E51 | E62 | E72 | E82 | … | E242 | 6 | 7 | 5 | 5 | … | 5 | 4 |
2 | E12 | E21 | E31 | E42 | E51 | E62 | E72 | E84 | … | E242 | 7 | 8 | 7 | 8 | … | 4 | 6 |
3 | E11 | E21 | E32 | E41 | E51 | E61 | E72 | E82 | … | E242 | 5 | 6 | 6 | 6 | … | 6 | 5 |
4 | E11 | E21 | E32 | E41 | E51 | E62 | E72 | E82 | … | E242 | 7 | 7 | 7 | 8 | … | 5 | 6 |
5 | E11 | E21 | E32 | E43 | E52 | E61 | E71 | E82 | … | E241 | 8 | 6 | 8 | 8 | … | 7 | 6 |
6 | E12 | E21 | E31 | E43 | E51 | E61 | E72 | E82 | … | E242 | 6 | 6 | 5 | 5 | … | 6 | 6 |
7 | E12 | E21 | E32 | E42 | E52 | E62 | E71 | E83 | … | E241 | 4 | 5 | 3 | 3 | … | 5 | 2 |
8 | E11 | E21 | E32 | E41 | E52 | E62 | E72 | E83 | … | E241 | 5 | 5 | 6 | 6 | … | 4 | 1 |
9 | E11 | E21 | E33 | E43 | E52 | E62 | E72 | E84 | … | E242 | 8 | 9 | 7 | 7 | … | 6 | 8 |
10 | E12 | E21 | E31 | E42 | E51 | E61 | E71 | E82 | … | E242 | 6 | 6 | 7 | 5 | … | 5 | 6 |
11 | E11 | E21 | E32 | E43 | E51 | E61 | E71 | E83 | … | E242 | 4 | 3 | 5 | 5 | … | 5 | 5 |
12 | E11 | E21 | E31 | E43 | E52 | E62 | E71 | E82 | … | E242 | 5 | 3 | 5 | 6 | … | 6 | 4 |
13 | E13 | E21 | E32 | E41 | E51 | E62 | E72 | E82 | … | E242 | 6 | 6 | 7 | 5 | … | 5 | 6 |
… | … | … | … | … | … | … | … | … | … | … | … | … | … | … | … | … | … |
63 | E13 | E21 | E31 | E43 | E52 | E61 | E72 | E84 | … | E242 | 4 | 5 | 5 | 5 | … | 4 | 4 |
64 | E11 | E21 | E32 | E41 | E52 | E62 | E71 | E84 | … | E242 | 6 | 7 | 5 | 5 | … | 6 | 6 |
Modules | The utility | Standard error | The importance degree | Modules | The utility | Standard error | The importance degree | ||
Buy transformer | S9 | 0.127 | 0.13 | 1.976 | Winding temperature fiber monitoring | iOFT2020 | −0.086 | 0.13 | 4.703 |
S10 | −0.069 | 0.152 | iOFT2021 | 0.233 | 0.152 | ||||
S11 | −0.058 | 0.152 | Not monitoring | −0.147 | 0.152 | ||||
Basic auxiliary modules of the transformer | JC1 | 0.047 | 0.097 | 1.643 | Cooling unit monitoring | iCSM2020 | 0.196 | 0.13 | 6.094 |
JC2 | −0.047 | 0.097 | iCSM2021 | 0.111 | 0.152 | ||||
High pressure bushing | COT-550/800 | 0.028 | 0.13 | 4.738 | Not monitoring | −0.307 | 0.152 | ||
COT-551/800 | 0.046 | 0.152 | Routine testing | Routine testing | 0.173 | 0.097 | 4.317 | ||
COT-552/800 | −0.074 | 0.152 | Not testing | −0.173 | 0.097 | ||||
Low pressure bushing | BD-20/3150 | −0.243 | 0.13 | 6.611 | Typical testing | Typical testing | 0.162 | 0.097 | 4.028 |
BD-21/3151 | 0.433 | 0.152 | Not testing | −0.162 | 0.097 | ||||
BD-22/3152 | −0.19 | 0.152 | Special testing | Special testing | 0.358 | 0.097 | 6.469 | ||
Online filter oil machine | R1 | 0.066 | 0.097 | 4.63 | Not testing | −0.358 | 0.097 | ||
R2 | −0.066 | 0.097 | Financial services | Manufacturer loan | 0.085 | 0.168 | 4.774 | ||
Gas relay | BF-80/10 | −0.01 | 0.097 | 2.335 | The third party loan | 0.16 | 0.168 | ||
BF-88/10 | 0.01 | 0.097 | Bank installment loan | −0.15 | 0.168 | ||||
Pressure relief valve | YSF8-55/130KJ | 0.017 | 0.097 | 2.684 | Not loan | −0.095 | 0.168 | ||
YSF8-56/130KJ | −0.017 | 0.097 | Transportation services | The third party transporting | −0.186 | 0.13 | 4.666 | ||
High and low voltage switch control cabinet | K1 | −0.388 | 0.168 | 5.314 | Seller transporting | 0.021 | 0.152 | ||
K2 | 0.271 | 0.168 | Customer transporting | 0.165 | 0.152 | ||||
K3 | 0.169 | 0.168 | Installation services | The third party installation | −0.06 | 0.097 | 3.485 | ||
Not provided | −0.052 | 0.168 | Seller installation | 0.06 | 0.097 | ||||
Chromatography monitoring | iMGA2020 | −0.157 | 0.13 | 5.241 | Warranty maintenance services | One-year warranty | 0.185 | 0.13 | 5.039 |
iMGA2021 | 0.114 | 0.152 | Two-year warranty | −0.236 | 0.152 | ||||
Not monitoring | 0.043 | 0.152 | Three-year warranty | 0.051 | 0.152 | ||||
Partial discharge monitoring | iPDM2020T | −0.216 | 0.13 | 4.778 | Non-warranty maintenance services | Routine maintenance | 0.233 | 0.097 | 4.272 |
iPDM2021T | 0.028 | 0.152 | Full services | −0.233 | 0.097 | ||||
Not monitoring | 0.188 | 0.152 | Recycling services | Old for new | 0.017 | 0.13 | 1.555 | ||
Casing insulation monitoring | iIMM2020 | −0.042 | 0.13 | 4.468 | Full recovery | 0.4 | 0.152 | ||
iIMM2021 | 0.016 | 0.152 | Non-recovery | −0.418 | 0.152 | ||||
Not monitoring | 0.026 | 0.152 | Spare parts | SP1 | 0.125 | 0.097 | 1.425 | ||
Core monitoring | iOCM2020 | 0.051 | 0.13 | 4.755 | SP2 | −0.125 | 0.097 | ||
iOCM2021 | 0.155 | 0.152 | |||||||
Not monitoring | −0.206 | 0.152 | |||||||
(constant) | 5.75 | 0.148 | (constant) | 5.75 | 0.148 |
Modules | The utility | Standard error | The importance degree | Modules | The utility | Standard error | The importance degree | ||
Lease transformer | S9 | −0.206 | 0.261 | 2.062 | Core monitoring | iOCM2020 | 0.108 | 0.261 | 6.721 |
S10 | −0.51 | 0.307 | iOCM2021 | 0.31 | 0.307 | ||||
S11 | 0.715 | 0.307 | Not monitoring | −0.419 | 0.307 | ||||
Basic auxiliary modules of the transformer | JC1 | −0.077 | 0.196 | 2.173 | Winding temperature fiber monitoring | iOFT2020 | 0.114 | 0.261 | 3.857 |
JC2 | 0.077 | 0.196 | iOFT2021 | −0.205 | 0.307 | ||||
High pressure bushing | COT-550/800 | −0.175 | 0.261 | 5.197 | Not monitoring | 0.091 | 0.307 | ||
COT-551/800 | 0.327 | 0.307 | Cooling unit monitoring | iCSM2020 | −0.05 | 0.261 | 3.28 | ||
COT-552/800 | −0.152 | 0.307 | iCSM2021 | −0.058 | 0.307 | ||||
Low pressure bushing | BD-20/3150 | −0.481 | 0.261 | 7.479 | Not monitoring | 0.108 | 0.307 | ||
BD-21/3151 | 0.317 | 0.307 | Routine testing | Routine testing | −0.081 | 0.196 | 4.904 | ||
BD-22/3152 | 0.163 | 0.307 | Not testing | 0.081 | 0.196 | ||||
Online filter oil machine | R1 | −0.085 | 0.196 | 2.263 | Typical testing | Typical testing | −0.281 | 0.196 | 4.85 |
R2 | 0.085 | 0.196 | Not testing | 0.281 | 0.196 | ||||
Gas relay | BF-80/10 | −0.013 | 0.196 | 2.167 | Special testing | Special testing | 0.027 | 0.196 | 4.971 |
BF-88/10 | 0.013 | 0.196 | Not testing | −0.027 | 0.196 | ||||
Pressure relief valve | YSF8-55/130KJ | −0.142 | 0.196 | 2.771 | Transportation services | The third party transporting | 0.133 | 0.261 | 3.362 |
YSF8-56/130KJ | 0.142 | 0.196 | Seller transporting | −0.079 | 0.307 | ||||
High and low voltage switch control cabinet | K1 | 0.25 | 0.34 | 7.549 | Customer transporting | −0.054 | 0.307 | ||
K2 | −0.4 | 0.34 | Installation services | The third party installation | 0.044 | 0.196 | 4.592 | ||
K3 | 0.142 | 0.34 | Seller installation | −0.044 | 0.196 | ||||
Not providing | 0.008 | 0.34 | Maintenance services | One-year warranty | −0.292 | 0.261 | 6.278 | ||
Chromatography monitoring | iMGA2020 | −0.178 | 0.261 | 4.384 | Two-year warranty | 0.31 | 0.307 | ||
iMGA2021 | −0.09 | 0.307 | Three-year warranty | −0.019 | 0.307 | ||||
Not monitoring | 0.268 | 0.307 | Non-warranty maintenance services | Routine maintenance | 0.127 | 0.196 | 2.935 | ||
Partial discharge monitoring | iPDM2020T | 0.169 | 0.261 | 8.459 | Full services | −0.127 | 0.196 | ||
iPDM2021T | −0.541 | 0.307 | Spare parts | SP1 | −0.004 | 0.196 | 2.313 | ||
Not monitoring | 0.372 | 0.307 | SP2 | 0.004 | 0.196 | ||||
Casing insulation monitoring | iIMM2020 | −0.044 | 0.261 | 7.433 | |||||
iIMM2021 | −0.374 | 0.307 | |||||||
Not monitoring | 0.418 | 0.307 | |||||||
(constant) | 5.587 | 0.292 | (constant) | 5.587 | 0.292 |
Modules | The utility | Standard error | The importance degree | Modules | The utility | Standard error | The importance degree | ||
Purchase electric power | S9 | −0.16 | 0.16 | 11.606 | Casing insulation monitoring | iIMM2020 | −0.048 | 0.12 | 5.825 |
S10 | 0.068 | 0.188 | iIMM2021 | 0.048 | 0.12 | ||||
S11 | 0.093 | 0.188 | Core monitoring | iOCM2020 | 0.183 | 0.12 | 4.038 | ||
Basic auxiliary modules of the transformer | JC1 | 0.114 | 0.12 | 3.013 | iOCM2021 | −0.183 | 0.12 | ||
JC2 | −0.114 | 0.12 | Winding temperature fiber monitoring | iOFT2020 | 0.117 | 0.12 | 3.555 | ||
High pressure bushing | COT-550/800 | −0.198 | 0.16 | 5.665 | iOFT2021 | −0.117 | 0.12 | ||
COT-551/800 | 0.252 | 0.188 | Cooling unit monitoring | iCSM2020 | −0.089 | 0.12 | 4.352 | ||
COT-552/800 | −0.054 | 0.188 | iCSM2021 | 0.089 | 0.12 | ||||
Low pressure bushing | BD-20/3150 | −0.035 | 0.16 | 5.875 | Routine testing | Routine testing | −0.011 | 0.12 | 4.021 |
BD-21/3151 | 0.099 | 0.188 | Not testing | 0.011 | 0.12 | ||||
BD-22/3152 | −0.064 | 0.188 | Typical testing | Typical testing | 0.008 | 0.12 | 4.636 | ||
Online filter oil machine | R1 | 0.123 | 0.12 | 4.376 | Not testing | −0.008 | 0.12 | ||
R2 | −0.123 | 0.12 | Special testing | Special testing | 0.205 | 0.12 | 4.405 | ||
Gas relay | BF-80/10 | −0.092 | 0.12 | 5.005 | Not testing | −0.205 | 0.12 | ||
BF-88/10 | 0.092 | 0.12 | Transportation services | The third party transporting | 0.352 | 0.16 | 4.170 | ||
Pressure relief valve | YSF8-55/130KJ | 0.142 | 0.12 | 4.986 | Seller transporting | −0.31 | 0.188 | ||
YSF8-56/130KJ | −0.142 | 0.12 | Customer transporting | −0.042 | 0.188 | ||||
High and low voltage switch control cabinet | K1 | 0.458 | 0.208 | 4.098 | Installation services | The third party installation | −0.027 | 0.12 | 3.521 |
K2 | −0.405 | 0.208 | Seller installation | 0.027 | 0.12 | ||||
K3 | −0.211 | 0.208 | Non-warranty maintenance services | S1 | −0.014 | 0.12 | 3.801 | ||
Not providing | 0.158 | 0.208 | S2 | 0.014 | 0.12 | ||||
Chromatography monitoring | iMGA2020 | 0.002 | 0.12 | 4.412 | Spare parts | SP1 | 0.18 | 0.12 | 4.248 |
iMGA2021 | −0.002 | 0.12 | SP2 | −0.18 | 0.12 | ||||
Partial discharge monitoring | iPDM2020T | −0.202 | 0.12 | 4.392 | |||||
iPDM2021T | 0.202 | 0.12 | |||||||
(constant) | 5.621 | 0.144 |