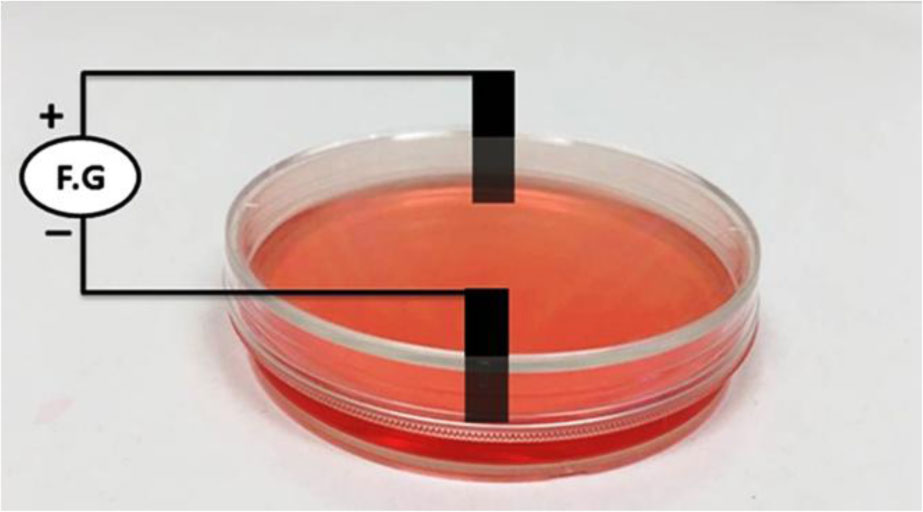
Tumor Treating Fields (TTFs) is a new tumor electric therapy that has success with many tumor types. This modality is very low-intensity alternating current (AC) fields (1–3 V/cm) and intermediate frequency (100–300 kHz). The delivery of TTFs was applied only through completely insulated electrodes by using external high voltage to avoid any reactions that may occur through using conductive electrodes, specially the generation of oxidative reactions. In order to achieve the required effect, the patient can be exposed to the technique for continuous three days, which may lead to long-term side effects. The adoption of the idea that TTFs should be applied through insulated electrodes did not give the chance to evaluate the uninsulated electrodes to conduct TTFs. This study aims to evaluate the possibility of using uninsulated electrodes, find the optimum exposure time, and determine the accompanying oxidative stress on human non-small cell lung carcinoma (NSCLC) cells. Here we found that applying TTFs through uninsulated electrodes produced 18–29% tumor growth inhibition for exposure time of 2.5 min to 40 min without significant oxidative stress. These findings will lead to the possibility of using the uninsulated electrodes TTFs in simple technology. The treatment quality will be improved by highly decreasing the exposure time.
Citation: Mamdouh M. Shawki, Seham Elabd. Tumor treating fields (TTFs) using uninsulated electrodes induce cell death in human non-small cell lung carcinoma (NSCLC) cells[J]. AIMS Biophysics, 2021, 8(2): 143-156. doi: 10.3934/biophy.2021011
[1] | Moataz M. Fahmy, Sohier M. El-Kholey, Seham Elabd, Mamdouh M. Shawki . Effect of changing the alternating electric current frequency on the viability of human liver cancer cell line (HEPG2). AIMS Biophysics, 2025, 12(1): 1-13. doi: 10.3934/biophy.2025001 |
[2] | Min Zhang, Shijun Lin, Wendi Xiao, Danhua Chen, Dongxia Yang, Youming Zhang . Applications of single-cell sequencing for human lung cancer: the progress and the future perspective. AIMS Biophysics, 2017, 4(2): 210-221. doi: 10.3934/biophy.2017.2.210 |
[3] | Mario P. Carante, Francesca Ballarini . Modelling cell death for cancer hadrontherapy. AIMS Biophysics, 2017, 4(3): 465-490. doi: 10.3934/biophy.2017.3.465 |
[4] | Massimo Fioranelli, Hijaz Ahmad, Maria Grazia Roccia, Aroonkumar Beesham, Zahir Shah . A mathematical model for inducing T-cells around tumor cells by using exchanged waves between graphene sheets interior and exterior of body. AIMS Biophysics, 2022, 9(4): 388-401. doi: 10.3934/biophy.2022030 |
[5] | Michael R Hamblin . Mechanisms and applications of the anti-inflammatory effects of photobiomodulation. AIMS Biophysics, 2017, 4(3): 337-361. doi: 10.3934/biophy.2017.3.337 |
[6] | Mikayel Ginovyan, Svetlana Hovhannisyan, Hayarpi Javrushyan, Gohar Sevoyan, Zaruhi Karabekian, Narine Zakaryan, Naira Sahakyan, Nikolay Avtandilyan . Screening revealed the strong cytotoxic activity of Alchemilla smirnovii and Hypericum alpestre ethanol extracts on different cancer cell lines. AIMS Biophysics, 2023, 10(1): 12-22. doi: 10.3934/biophy.2023002 |
[7] | Silpak Biswas, Rintu Das, Ena Ray Banerjee . Role of free radicals in human inflammatory diseases. AIMS Biophysics, 2017, 4(4): 596-614. doi: 10.3934/biophy.2017.4.596 |
[8] | Perumalsamy Balaji, Anbazhagan Murugadas, Lauri Paasonen, Sellathamby Shanmugaapriya, Mohammad A. Akbarsha . Efficiency of GrowDex® nanofibrillar cellulosic hydrogel when generating homotypic and heterotypic 3D tumor spheroids. AIMS Biophysics, 2022, 9(3): 221-234. doi: 10.3934/biophy.2022019 |
[9] | Satoru Ito, Kishio Furuya, Masahiro Sokabe, Yoshinori Hasegawa . Cellular ATP release in the lung and airway. AIMS Biophysics, 2016, 3(4): 571-584. doi: 10.3934/biophy.2016.4.571 |
[10] | Zhe Mei, Zhiwen Liu, Zhiguo Zhou . A compact and low cost microfluidic cell impedance detection system. AIMS Biophysics, 2016, 3(4): 596-608. doi: 10.3934/biophy.2016.4.596 |
Tumor Treating Fields (TTFs) is a new tumor electric therapy that has success with many tumor types. This modality is very low-intensity alternating current (AC) fields (1–3 V/cm) and intermediate frequency (100–300 kHz). The delivery of TTFs was applied only through completely insulated electrodes by using external high voltage to avoid any reactions that may occur through using conductive electrodes, specially the generation of oxidative reactions. In order to achieve the required effect, the patient can be exposed to the technique for continuous three days, which may lead to long-term side effects. The adoption of the idea that TTFs should be applied through insulated electrodes did not give the chance to evaluate the uninsulated electrodes to conduct TTFs. This study aims to evaluate the possibility of using uninsulated electrodes, find the optimum exposure time, and determine the accompanying oxidative stress on human non-small cell lung carcinoma (NSCLC) cells. Here we found that applying TTFs through uninsulated electrodes produced 18–29% tumor growth inhibition for exposure time of 2.5 min to 40 min without significant oxidative stress. These findings will lead to the possibility of using the uninsulated electrodes TTFs in simple technology. The treatment quality will be improved by highly decreasing the exposure time.
Lung cancer imposes a disease burden on the world. It has progressed from a rare disease to the most common cancer and the greatest cause of cancer-related death in the world [1]. It can be divided into non-small cell lung cancer (NSCLC) which counts 80–85% of lung cancer, and small cell lung cancer (SCLC) [2]. For a long time before, the used treatment regimens are limited to chemotherapy and radiotherapy with or without surgery; these therapies can destroy tumor cells and have a lethal effect on normal healthy cells [3]. Therefore, the search for more tolerable and efficacious anticancer therapies continues.
The effects of external electric fields on biological cells have been extensively studied. In order to elucidate a possible impact of electric fields on cancer treatments, various trials that included irreversible electroporation [4] and electro-chemotherapy have been developed in the past [5]. A new cancer electrotherapy technique has been proposed which called Tumor Treating Fields (TTFs), and has been approved by Food and Drug Administration (FDA) in 2011 for the treatment of certain tumors, including brain tumor and glioblastoma [6]. This modality depends on very low-intensity alternating current (AC) fields (1–3 V/cm) and intermediate frequency (100–300 kHz). TTFs can destroy many types of the tumor without affecting the surrounding normal cells [7]. TTFs selectively disrupt cancer cells' ability to divide by inhibiting mitotic spindle formation during metaphase, which blocks cell proliferation, leading to growth inhibition [8].
Since TTFs have been used and the only way to deliver the electric field is through specially insulated electrodes applied to the skin surface. All the different devices that were reported using insulated electrodes differ in the type of insulation, either metal wires insulated with a thin film of an insulator or with a high dielectric-constant material [9]. No conductive electrodes were reported to be used in TTFs. Both insulated electrodes and conductive electrodes have suggested comparative advantages as well as drawbacks in TTFs application.
Insulating (capacitive) electrodes have a significant advantage in delivering potential biological tissue differences without galvanic contact. They use a high electric current capable of breaking down the high electric resistance of insulating material that covers the whole electrode, including the contacting tip itself. The operating of these electrodes are with displacement currents instead of real charge currents, allowing the avoidance of the electrolytic reactions at the electrode surface with the accompanied toxic ions release [10]. On the other hand, the application of TTFs using insulating electrodes can affect the patients' life quality because they are treated outside the hospital environment and advised to wear the device for at least 18 h/day for an average of 4 weeks [11]. During the exposure period, treatment is delivered via electrodes attached to the patient's scalp, powered by a portable battery [12].
Uninsulated electrodes (conductive electrodes) have the benefit of conducting the same electrical energy in a very short time compared with insulated electrodes. There are many reaction parameters that can control the oxidants generation such as electrolyte composition, electrical energy, pH, temperature, and electrolysis type. The electrode material has been considered as the most significant factor governing the species and yield of the generated oxidants [13].
TTFs using insulated electrodes have the potential for long-term side effects. It is, therefore, essential to study methods that increase the intensity of electric fields applied to tumors, thereby enhancing its therapeutic effects while minimizing exposure of organs at risk [14]. In the current study, the efficiency of using uninsulated electrodes was evaluated, which wasn't reported before. This evaluation isn't limited to the percent of cell death but also extends to determine the total oxidative stress that may be introduced into the medium with time. Decreasing exposure time to the few min range is the main point in this study to improve the quality of the treatment. As we don't know precisely when the cell death plateau can be obtained, we examined TTFs time interval curve in the range of 2.5 min to 40 min.
TTFs conditions were delivered through a pair of plate silver/silver chloride electrodes of 1.5 cm2 surface area. The distance between the two electrodes was 5 cm. Two holes in the lid of the cell culture dish were made; through them, the two electrodes passed then fixed. Each electrode is connected to one output terminal of the function generator (F.G); Figure 1 is a schematic diagram of the exposure system.
5 V, 150 KHz sine waves were applied to the tumor cell line culture media via (CALTEK, CA1640P-02 function generator/counter, serial number: 06mg0676, USA). So the electric field was 1V/cm. The exposure time was ranged from 0 min (control) to 40 min. The current passing through the medium containing the cells was practically measured using a digital multimeter (VC9205A CE, Made in China); the measured value was 1.8 mA. The temperature was measured every 5 min using a digital thermometer.
A549 cell line, a human lung cancer cell line (adenocarcinomic human alveolar basal epithelial cells), was obtained from the Medical Research Institute (MRI), Alexandria University, Alexandria, Egypt. Cells were cultured on collagen-coated tissue culture dishes (Sarstedt AG & Co, Nümbrecht, Germany) in a complete medium of high glucose Dulbecco's Modified Eagle's Medium (DMEM) with L-Glutamine (Lonza, Belgium), supplemented with 10% fetal bovine serum (FBS) (Sigma, Germany), 1% penicillin and streptomycin (Biochrom GmbH). Cells were kept under standard cell culture conditions at 37 °C in a humidified CO2 incubator containing 5% CO2. Cells were grown in a monolayer, and the cell adherence rate during subculture was required to reach 80% confluence. In all experiments, cultures of 80% confluence were used with a maximum passage number of 20–25.
The self-renewal capacity of the A549 cells was evaluated by colony-forming efficiency assays [15]. Cells at the exponential growth phase were detached with trypsin and counted using a hemocytometer. 5×102 cells per well were seeded in six-well plates at 37 °C with 5% CO2. After overnight incubation, cells were exposed to TTFs-conductive electrodes at indicated durations and then continuously incubated for 10–14 days. After incubation, cells were washed with PBS twice, fixed with fixing solution (Acetic acid/methanol, 1: 7) for 10 min, and stained with 0.5% crystal violet (Sigma-Aldrich) for 10 min at room temperature. After staining, cells were washed with tap water. The colony is defined to consist of > 50 cells. Visible colonies were counted. Colony-forming efficiency was calculated as colony-forming unit numbers, colony formation rate = (number of colonies/number of seeded cells) × 100%.
Detection of apoptotic/necrotic cells after TTFs-conductive electrodes exposure was done by using Annexin V/propidium iodide (PI) staining. Cells were exposed to TTF for the indicated time and then incubated for 48 h. After incubation, the cells were subsequently washed with ice-cold PBS, trypsinized, centrifuged at 2,000 g for 2 min. The cell pellet was resuspended in 1x binding buffer (10 mm HEPES/NaOH (pH 7.4), 140 mm NaCl, and 2.5 mm CaCl2). Aliquots (100 µL) of the cell solution were mixed with 5 µL of Annexin V-FITC (BioVision, Milpitas, CA) and 10 µL of PI stock solution (50 µg/mL in PBS) via gentle vortexing, followed by 15 min of incubation at room temperature in the dark. 400 µL of 1x binding buffer was added to each sample. The prepared samples were analyzed using a FACScan flow cytometer (Becton Dickinson, Franklin Lakes, NJ, USA). Data were acquired and recorded using the Cellquest software. In general, samples were analyzed at a low flow rate, and 10,000 events were acquired for each sample [16].
Cell cycle analysis was done by determining the quantity of DNA content. This is preceded by staining the cells with a fluorescent DNA-binding dye like propidium iodide (PI), which is added to permeabilized single cells [17]. PI will bind to DNA, and during flow cytometry, the DNA will emit a fluorescent signal which is vary depending on the amount of DNA in the cell. The cells that are in the S phase will have more DNA than cells in G1. The cells in G2 will be approximately twice as bright as cells in G1. Because of this, G2 and M phases often cannot be distinguished from one another because they share similar DNA content.
After TTFs-conductive electrodes exposure for the indicated time, cells were harvested, washed with PBS. Cells were fixed and permeabilized in 80% ethanol for 1h. Then, the fixed cells were washed with phosphate buffer solution (PBS) followed by incubation with RNase I (10 µg/mL) at 37 °C for 30 min. Finally, the cells were resuspended in PI (50 µg/mL) at 4 °C for 30 min and then detected using a FACScan flow cytometer (Becton Dickinson, Franklin Lakes, NJ, USA). Data were acquired and recorded using the Cellquest software.
The cells' monolayers were exposed to TTField for the indicated time. After incubation, the cells were lysed, and the protein concentration in the samples was measured; then the oxidative stress markers were determined in each sample.
A549 cells were washed twice with phosphate-buffered saline (PBS), scraped into PBS, pelleted by centrifugation at 2,000 g for 2 min, and lysed by using RIPA buffer (50 mM Tris pH 7.5, 150 mM NaCl, 1% NP40, 0.5% Na-deoxycholate and protease inhibitor) and incubated for 10 min on ice. The protein extract was cleared by centrifugation at 13,000 g at 4 °C for 10 min. Protein content in the samples (protein extract) was measured according to the method of Bradford [18], and bovine serum albumin (BSA) was used as a standard.
Total antioxidant capacity (TAC) was measured via a SET (single electron transfer) mechanism. In SET mechanisms, the antioxidant provides an electron to the free radical and itself then becomes a radical cation. In this mechanism, the antioxidant's ionization potential (IP) is the most important energetic factor in evaluating the antioxidant action. In the presence of antioxidants, copper (II) (Cu2+) is reduced to copper (I) (Cu+). In turn, the copper (I) (Cu+) ion interacts with a chromogen to produce a colour compound [19]. The antioxidant capacity of samples was calculated by detecting the absorbance value at 490 nm.
Glutathione content, oxidized (GSSG), and reduced (GSH) glutathione in cell lysates were determined by Cayman's assay kit based on the enzymatic recycling method using glutathione reductase (GR) [20]–[21]. The sulfhydryl group in GSH reacts with DTNB ((5,5′-dithio-bis-2-nitrobenzoic acid) to form the disulfide GSTNB and the yellow-colored compound 5-thio-2-nitrobenzoic acid (TNB). The disulfide product (GSTNB) is then reduced by GR in the presence of NADPH, recycling GSH back into the reaction. GSH is easily oxidized to the disulfide dimer GSSG. The absorbance of TNB was measured at 405 or 412 nm. The rate of TNB production is directly proportional to the concentration of glutathione content in the sample.
All data were expressed as mean ± standard deviation (SD) from three independent repeats. ANOVA was used to evaluate the difference between multiple groups. Significant differences between experimental groups were determined using a two-tailed Student's t-test (Excel 2013 Microsoft, USA). Results were considered statistically significant when the p-value < 0.05.
The inhibitory effect of TTF using conductive electrodes treatment on lung cancer cell proliferation using A549 cells was examined. TTFs-conductive electrodes were applied to A549 cells for different durations 0, 5, and 10 min. After incubation, the cells were fixed and stained. The result of the colony-forming assay (Figure 2) indicated that the TTFs-conductive electrodes exposure inhibited A549 cells proliferation to a greater degree than that in the control cells. The results clearly indicated that the different durations of TTFs-conductive electrodes showed a cytotoxic effect on A549 cells in a time-dependent manner as the time of exposure increased, the viability of the cells decreased.
To determine whether this suppression of proliferation is due to cell death, Annexin V-FITC/propidium iodide (PI) staining protocol was performed in A549 cells. A549 cells were exposed to TTFs-conductive electrodes with different durations ranging from 2.5 min to 40 min, and 0 min exposure was used as the control, as described. Then cells were subsequently stained with Annexin V/PI and examined through flow cytometry. The result analysis showed that the rate of cell death after the TTF-conductive electrodes exposure increased in a time-dependant manner until reach the plateau at 10 min exposure and after that, there is no clear increase in the rate of cell death (Figure 3). The results indicated that exposure to diverse durations of TTFs-conductive electrodes increased cell death considerably, and the inhibition percentage of cell proliferation was similar to the colony-forming assay results.
Annexin V/PI staining was also used to evaluate the type of cell death in TTFs-conductive electrodes exposed cells related to necrosis or apoptosis pathway. As in Figures 4 and 5 the percentage of necrotic cells after exposed to TTFs-conductive electrodes for 0, 2.5, 5, 10, 30, 40 min were 0.76%, 15.66%, 19.09%, 23.21%, 17.86%, 6.91% and 7.61% respectively. A significantly higher ratio of necrotic cells was found in the TTFs exposed cells for 10 min as compared to control cells. The results showed that various TTFs durations induced a time-dependent cytotoxic effect on A549 cells until they reach the plateau at 10 min, and this is occurred by inducing cell necrosis (Figure 4) and after that (20, 30, and 40 min), the change was not obvious.
Figure 4I: Diagram represents the dual staining with annexin V and propidium iodide (PI). In normal cells, phosphatidyl serines (PS), a membrane phospholipid, are present on the cell membrane's inner layer, so Annexin V cannot attach to the cells. During early apoptosis, the PS is exposed to the cell membrane's outer layer, where they attach to the Annexin V and stain the cell surface green. In a live, intact cell, PI cannot enter the cell and label dsDNA/RNA. During late apoptosis, the cell membrane PI enters the cell due to the loss of membrane integrity and stains the contents red.
Figure 4II: Contour diagram of Annexin V/PI flow cytometry of A549 cells after treatment with TTFs at different time intervals (0–40 min). Each panel's lower left quadrant shows the viable cells, which exclude PI and are negative for Annexin V binding. The upper right quadrants contain the dead cells, positive for Annexin V binding and for PI uptake. The lower right quadrants represent the early apoptotic cells, Annexin V negative and PI positive, demonstrating loss of cytoplasmic membrane integrity. The upper left quadrant contains the necrotic cells, Annexin V positive and PI negative.
Figure 4III: Effect of TTFs-uninsulated electrodes selectively increases A549 cell necrosis. The figure represents the percentage of live, necrotic, apoptotic, and dead cells in each sample. Columns show the mean values of three experiments.
As reported before, TTFs exert their toxicity on mitotic cells that exhibit rapid microtubule dynamics. So, the cell cycle progression upon exposure to TTFs-conductive electrodes was estimated. A549 cells were exposed to TTFs-conductive electrodes for the indicated time, and then cells were fixed and stained with PI and examined by flow cytometry. The results indicated that less cells re-entered the G1 phase, while the number of cells in the G0-phase was increased after exposure to TTFs-conductive electrodes as compared to control (Figure 5). Flow cytometry analysis revealed that cells exposed to TTFs delivered through conductive electrodes were blocked in cell cycle progression and induced growth arrest. This result could explain why the cytotoxic effect of TTFs showed a continuous plateau on A549 cells. Exposure of TTFs significantly inhibited the growth of A549 cells through induction of cell death and cell cycle arrest supported by a decrease in G1 phase and increase of G0-phase or quiescence cell populations. Less cells enter G1 phase and accumulate in G0, and so cells stop their cell cycling to repair the damage which results from TTFs exposure. Collectively, after exposure to TTFs-conductive electrodes, the number of viable cells was reduced, the ratio of dead/alive cells increased, and the number of cells re-entered the G1 phase reduced.
The main drawback of using such kinds of electrodes is the expected free radicals production. Determination of the oxidative stress leads to select the appropriate exposure time depending on the risk-benefit ratio. Antioxidants play a critical role in preventing the formation of and scavenging of free radicals. Measurement of the antioxidant capacity of samples indicates the overall capability of oxidative damage. Total antioxidant capacity was measured in TTF-exposed cells and compared to control. The results (Table 1) showed that no significant changes in the total antioxidant capacity were observed after TTF exposure as compared to control cells.
Total antioxidant capacity (U/mg protein) | GSH (U/mg protein) | GSSG (U/mg protein) | GSH / GSSG ratio | |||||
Group | Control | TTFs | Control | TTFs | Control | TTFs | Control | TTFs |
Mean | 98.5 | 89 | 54.3 | 45.3 | 0.578 | 0.503 | 93.9 | 90.1 |
SD | 1.5 | 7 | 4.3 | 5.3 | 0.03 | 0.09 | 4.3 | 5.3 |
P-value | 0.0831 | 0.0844 | 0.2427 | 0.3895 |
Glutathione is present in the cells in both the reduced (GSH) and oxidized (GSSG) forms. Because of the NADPH-dependent enzyme glutathione reductase (GR) action, the cellular content of glutathione is predominantly in the form of GSH under physiological conditions. When cells are exposed to oxidative stress, GSSG accumulates. The GSH/GSSG ratio is considered as a sensitive indicator of cellular oxidative stress. The change in glutathione pool was determined in the presence and absence of TTFs-conductive electrodes, GSH and GSSG were measured, and GSH/GSSG ratio was calculated in the TTF-exposed samples and compared to control. The result clearly demonstrated that no significant changes in the glutathione pool (Table 1) in the presence and absence of TTF. Collectively, using TTFs-conductive electrodes did not induce a significant difference in the cell's oxidative stress status.
There was very small increase in the measured temperature after 5 min of TTFs exposure (0.05 ± 0.05 °C). The temperature increased after 40 min of exposure by only 0.7 ± 0.1 °C compared to control.
The results of the present study showed that the exposure to TTFs using conductive electrodes produces significant tumor inhibitory effects. The rate of cell proliferation was inhibited, the number of viable cells was reduced, and the ratio of dead/alive cells was increased. The number of cells re-entered the G1 phase was reduced leading to block the cell proliferation that results in growth inhibition. However, these growth inhibitory effects of TTFs occurred with no significant change in the cells' oxidative stress status.
While insulated electrodes TTFs have been evaluated for safety and efficacy in a number of tumor therapy studies, concerns remain regarding study design, quality of life, the long-term effect of long exposure time, and cost of treatment [22]. All the concerns are not for the used electric field, but they are mainly because insulated electrodes were used as the only way to deliver such fields. The current research article aimed to evaluate the other method of delivering such fields through normal conductive electrodes.
TTFs frequency (100 KHz to 300 KHz) can cause neither effective depolarization as in the case of lower than 1 KHz nor significant dielectric losses occurred at 10 MHz or higher, so it was considered to have no biological importance at the near past. Also the used intensity 1–3 V will not seriously heat the tissue [23]. This agrees with our results that the temperature of the media containing the cells did not change significantly after 40 min of exposure. Recently, TTFs is considered as a tumor electrotherapy via inhibition of cell division without systemic toxicity [24]. It is even considered as the fourth modality in cancer treatment [25].
The TTFs technique was modified using uninsulated electrodes to evaluate its effect in a single dose for low exposure time in the current work. The result of the current study indicated that we could get 18–29% tumor cell inhibition according to the exposure time without any synergetic method in a single dose using conductive electrodes.
The present study's hypothesis in using conductive electrodes is the proposed mechanism of interaction of the TTFs with cancer cells can be the same regardless of the type of the used electrodes either insulated or conductive. Some possible mechanisms can be responsible for the decrease in cell proliferation due to TTFs. The action of TTFs to move polar molecules during cytokinesis in the direction of the narrow neck that forms the two new cells leading to difficulty of the division process. TTFs selectively disrupt the ability of cancer cells to divide by interaction with microtubule spindle and inhibit mitotic spindle formation during metaphase, which reduces cell proliferation, resulting in growth inhibition [26]. TTFs can have an interaction with the membrane potential in dividing tumor cells, only causing possible effects on the ionic channels in tumor cells that lead to 10–17% change in tumor cell membrane potential [27]. The TTFs action can be also due to a type of immunological reaction that high-mobility group box 1 can be destroyed resulting in ATP release and calreticulin is exposed on the cell surface. At the same time, the depletion of high mobility protein produces an immune response against dying cells leading to apoptosis [28], so TTFs can promote cancer cells' engulfment by dendritic cells. Further studies are needed to explain TTF's molecular mechanism using conductive electrodes and investigate whether it is the same like TTF using insulated electrodes.
The results indicated that as the time of exposure increases, the ratio of the dead cells increases. These results are in agreement with the concept of the direct proportional between electric field dose and the apoptosis ratio [29]. In the current study, we could achieve more or less the same lethal effect of tumor cells using conductive electrodes in very short exposure time due to real charge currents used. The maximal inhibitory effect of TTFs depends on the type of tumor cell, electric field intensity, and the specific frequency [30]. 150 KHz was used in the current work because it was mentioned before as the maximum inhibitory frequency of using TTFs with insulated electrodes to treat human non-small cell lung carcinoma (NSCLC) cells [8].
The main concerns of using uninsulated electrodes are the free radicals production and the electrolysis reactions that may theoretically occur. The results showed no significant increase in antioxidant-related parameters. Regarding the ions that may be released during an electrochemical process, the rate of electrode reaction (υ) directly depends on the current (i) around the electrode as [31]:
Where A is the electrode area, n is the number of electrodes involved in the reaction and f is Faraday's constant. So, at fixed conditions, the three main electrode-related parameters are electrode material, configuration, and surface area. These factors affect the produced electric field, generated electrode reaction (amount of released ions), and the yielded oxidants. The used conditions in this work produced no significant increase in the free radicals and no significant change in the total oxidative stress. The selection of the conductive electrode-related parameters for TTFs can be a goal of future research.
The current work will be followed up with future research to improve it. Determination of the appropriate electrode material, dimensions, and configuration for uninsulated electrodes TTFs is an important step. Finding the optimum frequency for each tumor type and its corresponding normal adjacent tissue will lead to more specific lethal targeting. Further analysis of the influence of the commutation time on the anti-proliferative effect could enhance our results.
TTFs technique can be applied using uninsulated (conductive) electrodes to obtain tumor growth inhibitory effects without significant oxidative stress. This method will provide better life quality during treatment. It is a cheap method, no high technology is required, and without concern of long-term exposure.
[1] |
De Groot P, Wu CC, Carter BW, et al. (2018) The epidemiology of lung cancer. Transl Lung Cancer Res 7: 220-233. doi: 10.21037/tlcr.2018.05.06
![]() |
[2] |
Oser MG, Niederst MJ, Sequist LV, et al. (2015) Transformation from non-small-cell lung cancer to small-cell lung cancer: molecular drivers and cells of origin. Lancet Oncol 16: e165-e172. doi: 10.1016/S1470-2045(14)71180-5
![]() |
[3] | Lemjabbar-Alaoui H, Hassan OUI, Yang YW, et al. (2015) Lung cancer: biology and treatment options. BBA-Rev Cancer 1856: 189-210. |
[4] |
Rubinsky B (2007) Irreversible electroporation in medicine. Technol Cancer Res Treat 6: 255-259. doi: 10.1177/153303460700600401
![]() |
[5] |
Sersa G, Miklavcic D, Cemazar M, et al. (2008) Electrochemotherapy in treatment of tumours. Eur J Surg Oncol 34: 232-240. doi: 10.1016/j.ejso.2007.05.016
![]() |
[6] |
Kirson ED, Dbalý V, Tovaryš F, et al. (2007) Alternating electric fields arrest cell proliferation in animal tumor models and human brain tumors. Proc Natl Acad Sci 104: 10152-10157. doi: 10.1073/pnas.0702916104
![]() |
[7] |
Berkelmann L, Bader A, Meshksar S, et al. (2019) Tumour-treating fields (TTFields): Investigations on the mechanism of action by electromagnetic exposure of cells in telophase/cytokinesis. Sci Rep 9: 7362. doi: 10.1038/s41598-019-43621-9
![]() |
[8] |
Giladi M, Weinberg U, Schneiderman RS, et al. (2014) Alternating electric fields (tumor-treating fields therapy) can improve chemotherapy treatment efficacy in non-small cell lung cancer both in vitro and in vivo. Semin Oncol 41: S35-S41. doi: 10.1053/j.seminoncol.2014.09.006
![]() |
[9] | Porat Y, Giladi M, Schneiderman RS, et al. (2017) Determining the optimal inhibitory frequency for cancerous cells using tumor treating fields (TTFields). J Vis Exp 123: 55820. |
[10] |
Spinelli E, Haberman M (2010) Insulating electrodes: a review on biopotential front ends for dielectric skin–electrode interfaces. Physiol Meas 31: S183-S198. doi: 10.1088/0967-3334/31/10/S03
![]() |
[11] |
Wenger C, Miranda P, Salvador R, et al. (2018) A review on tumor-treating fields (TTFields): clinical implications inferred from computational modeling. IEEE Rev Biomed Eng 11: 195-207. doi: 10.1109/RBME.2017.2765282
![]() |
[12] |
Kanner AA, Wong ET, Villano JL, et al. (2014) Post hoc analyses of intention-to-treat population in phase III comparison of NovoTTF-100ATM system versus best physician's choice chemotherapy. Semin Oncol 41: S25-S34. doi: 10.1053/j.seminoncol.2014.09.008
![]() |
[13] |
Jeong J, Kimb C, Yoon J (2009) The effect of electrode material on the generation of oxidants and microbial inactivation in the electrochemical disinfection processes. Water Res 43: 895-901. doi: 10.1016/j.watres.2008.11.033
![]() |
[14] |
Sung J, Seo J, Jo Y, et al. (2018) Development of a method for improving the electric field distribution in patients undergoing tumor-treating fields therapy. J Korean Phys Soc 73: 1577-1583. doi: 10.3938/jkps.73.1577
![]() |
[15] |
Franken NAP, Rodermond HM, Stap J, et al. (2006) Clonogenic assay of cells in vitro. Nat Protoc 1: 2315-2319. doi: 10.1038/nprot.2006.339
![]() |
[16] |
Wlodkowic D, Skommer J, Darzynkiewicz Z (2009) Flow cytometry-based apoptosis detection. Apoptosis Totowa: Humana Press, 19-32. doi: 10.1007/978-1-60327-017-5_2
![]() |
[17] |
Pozarowski P, Darzynkiewicz Z (2004) Analysis of cell cycle by flow cytometry. Checkpoint Controls and Cancer Humana Press, 301-311. doi: 10.1385/1-59259-811-0:301
![]() |
[18] |
Bradford MM (1976) A rapid and sensitive method for the quantitation of microgram quantities of protein utilizing the principle of protein-dye binding. Anal Biochem 72: 248-254. doi: 10.1016/0003-2697(76)90527-3
![]() |
[19] |
Marques SS, Magalhães LM, Tóth IV, et al. (2014) Insights on antioxidant assays for biological samples based on the reduction of copper complexes-the importance of analytical conditions. Int J Mol Sci 15: 11387-11402. doi: 10.3390/ijms150711387
![]() |
[20] |
Baker MA, Cerniglia GJ, Zaman A (1990) Microtiter plate assay for the measurement of glutathione and glutathione disulfide in large numbers of biological samples. Anal Biochem 190: 360-365. doi: 10.1016/0003-2697(90)90208-Q
![]() |
[21] |
Eyer P, Podhradsky D (1986) Evaluation of the micromethod for determination of glutathione using enzymatic cycling and Ellman's reagent. Anal Biochem 153: 57-66. doi: 10.1016/0003-2697(86)90061-8
![]() |
[22] |
Shah PP, White T, Khalafallah AM, et al. (2020) A systematic review of tumor treating fields therapy for high grade gliomas. J Neuro-oncol 148: 433-443. doi: 10.1007/s11060-020-03563-z
![]() |
[23] |
Cifra M, Fields JZ, Farhadi A (2011) Electromagnetic cellular interactions. Prog Biophys Mol Biol 105: 223-246. doi: 10.1016/j.pbiomolbio.2010.07.003
![]() |
[24] |
Kim CY, Paek SH, Nam D, et al. (2020) Tumor treating fields plus temozolomide for newly diagnosed glioblastoma: a sub-group analysis of Korean patients in the EF-14 phase 3 trial. J Neuro-oncol 146: 399-406. doi: 10.1007/s11060-019-03361-2
![]() |
[25] |
Mun EJ, Babiker HM, Weinberg U, et al. (2018) Tumor-treating fields: A fourth modality in cancer treatment. Clin Cancer Res 24: 266-275. doi: 10.1158/1078-0432.CCR-17-1117
![]() |
[26] |
Tuszynski JA, Wenger C, Friesen DE, et al. (2016) An overview of sub-cellular mechanisms involved in the action of TTFields. Int J Environ Res Public Health 13: 1128. doi: 10.3390/ijerph13111128
![]() |
[27] |
Li X, Yang F, Rubinsky B (2020) A theoretical study on the biophysical mechanisms by which tumor treating fields affect tumor cells during mitosis. IEEE T Biomed Eng 67: 2594-2602. doi: 10.1109/TBME.2020.2965883
![]() |
[28] |
Voloshin T, Kaynan N, Davidi S, et al. (2020) Tumor-treating fields (TTFields) induce immunogenic cell death resulting in enhanced antitumor efficacy when combined with anti-PD-1 therapy. Cancer Immunol Immunother 69: 1191-1204. doi: 10.1007/s00262-020-02534-7
![]() |
[29] |
Wenger C, Salvador R, Basser PJ, et al. (2016) Improving tumor treating fields treatment efficacy in patients with glioblastoma using personalized array layouts. Int J Radiation Oncol Biol Phys 94: 1137-1143. doi: 10.1016/j.ijrobp.2015.11.042
![]() |
[30] |
Davies AM, Weinberg U, Palti Y (2013) Tumor treating fields: A new frontier in cancer therapy. Ann NY Acad Sci 1291: 86-95. doi: 10.1111/nyas.12112
![]() |
[31] | Puente C, López I (2018) Direct electrochemical synthesis of metal complexes. Direct Synthesis of Metal Complexes Elsevier, 87-141. |
1. | Vitalij Novickij, Nina Rembiałkowska, Paulina Kasperkiewicz-Wasilewska, Dagmara Baczyńska, Adam Rzechonek, Piotr Błasiak, Julita Kulbacka, Pulsed electric fields with calcium ions stimulate oxidative alternations and lipid peroxidation in human non-small cell lung cancer, 2022, 1864, 00052736, 184055, 10.1016/j.bbamem.2022.184055 | |
2. | Mamdouh M. Shawki, Alaa El Sadieque, Seham Elabd, Maisa E. Moustafa, Synergetic Effect of Tumor Treating Fields and Zinc Oxide Nanoparticles on Cell Apoptosis and Genotoxicity of Three Different Human Cancer Cell Lines, 2022, 27, 1420-3049, 4384, 10.3390/molecules27144384 |
Total antioxidant capacity (U/mg protein) | GSH (U/mg protein) | GSSG (U/mg protein) | GSH / GSSG ratio | |||||
Group | Control | TTFs | Control | TTFs | Control | TTFs | Control | TTFs |
Mean | 98.5 | 89 | 54.3 | 45.3 | 0.578 | 0.503 | 93.9 | 90.1 |
SD | 1.5 | 7 | 4.3 | 5.3 | 0.03 | 0.09 | 4.3 | 5.3 |
P-value | 0.0831 | 0.0844 | 0.2427 | 0.3895 |