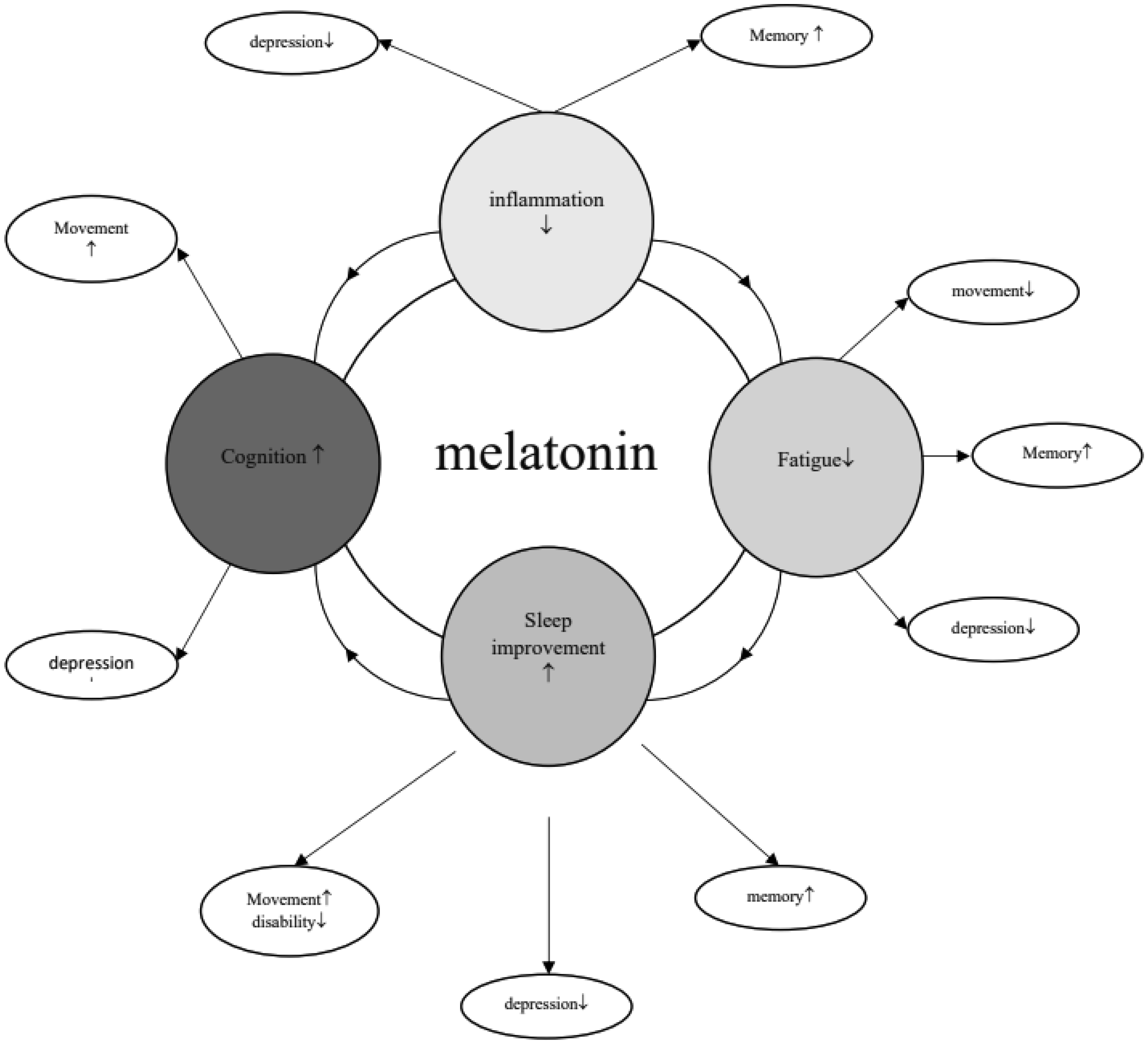
Multiple sclerosis (MS), is a debilitating neurological disease that currently has various treatments, like disease-modifying therapies, monoclonal antibodies, corticosteroids, and hormonal derivatives. Melatonin has several actions, like regulating circadian rhythms, which are usually used for insomnia. This scoping review aimed to explore the efficacy of melatonin, both as a standalone treatment and in conjunction with other drugs, in the management of MS in animal models.
We searched PubMed, Web of Science, EMBASE, and Google Scholar using (“melatonin” OR “melatonin receptor”) AND (“multiple sclerosis”) AND (“animal”). Animal studies that evaluated the effects of melatonin on the development, progression, and outcomes of MS were included. Human studies and other types of studies like case reports were excluded. We used narrative synthesis for reporting the results.
Overall, 21 studies were included, conducted on mice (n = 15) and rats (n = 6). Mostly, studies reported that melatonin led to normal circadian rhythms in animals. Melatonin in doses of both 50 and 100 mg/kg were useful in nociception latency. Melatonin in combination with other drugs like H-89, diisopropylamine dichloroacetate, gibberellins, and IFN-1β improved outcomes, while there was not improved cognition in combination with mesenchymal stem cells. In some tests, male subjects showed significantly better responses. There were controversial results regarding the effects of melatonin on cytokines, but overall, it led to a reduction in proinflammatory cytokines.
Melatonin overall demonstrated favorable outcomes regarding oxidative stress, anti-inflammation, and cytokine levels across various doses. It is recommended conducting systematic reviews and meta-analyses with a larger number of primary studies to provide more comprehensive insights.
Citation: Heliya Bandehagh, Farnaz Gozalpour, Ali Mousavi, Mahdi Hemmati Ghavshough. Effects of melatonin on the management of multiple sclerosis: A scoping review on animal studies[J]. AIMS Medical Science, 2024, 11(2): 137-156. doi: 10.3934/medsci.2024012
[1] | Belgüzar Kara, Elif Gökçe Tenekeci, Şeref Demirkaya . Factors Associated With Sleep Quality in Patients With Multiple Sclerosis. AIMS Medical Science, 2016, 3(2): 203-212. doi: 10.3934/medsci.2016.2.203 |
[2] | Claudia Chaufan, Laurie Manwell, Camila Heredia, Jennifer McDonald . COVID-19 vaccines and autoimmune disorders: A scoping review protocol. AIMS Medical Science, 2023, 10(4): 318-328. doi: 10.3934/medsci.2023025 |
[3] | Sobhan Helbi, Zahra Engardeh, Sahar Nickbin Poshtamsary, Zaynab Aminzadeh, Nahid Jivad . Down-regulation of IRF3 expression in Relapse-Remitting MS patients. AIMS Medical Science, 2019, 6(2): 140-147. doi: 10.3934/medsci.2019.2.140 |
[4] | Manishtha Rao, Madhvi Awasthi . A review on interventions to prevent osteoporosis and improve fracture healing in osteoporotic patients. AIMS Medical Science, 2020, 7(4): 243-268. doi: 10.3934/medsci.2020015 |
[5] | Jonathan Kissi, Daniel Kwame Kwansah Quansah, Jonathan Aseye Nutakor, Alex Boadi Dankyi, Yvette Adu-Gyamfi . Telehealth during COVID-19 pandemic era: a systematic review. AIMS Medical Science, 2022, 9(1): 81-97. doi: 10.3934/medsci.2022008 |
[6] | Kwon Yong, Martin Brechbiel . Application of 212Pb for Targeted α-particle Therapy (TAT): Pre-clinical and Mechanistic Understanding through to Clinical Translation. AIMS Medical Science, 2015, 2(3): 228-245. doi: 10.3934/medsci.2015.3.228 |
[7] | Payal A. Shah, John Goldberg . Novel Approaches to Pediatric Cancer: Immunotherapy. AIMS Medical Science, 2015, 2(2): 104-117. doi: 10.3934/medsci.2015.2.104 |
[8] | Bernardo Lopez Abel, María I Martínez-Soto, Maria Luz Couce . Integrative cardiology-state of the art of mind body therapies for the treatment of cardiovascular disease and risk factors. AIMS Medical Science, 2018, 5(1): 80-89. doi: 10.3934/medsci.2018.1.80 |
[9] | Vivek Radhakrishnan, Mark S. Swanson, Uttam K. Sinha . Monoclonal Antibodies as Treatment Modalities in Head and Neck Cancers. AIMS Medical Science, 2015, 2(4): 347-359. doi: 10.3934/medsci.2015.4.347 |
[10] | Elzbieta Marczak, Maria Szarras-Czapnik, Elzbieta Moszczyńska . Endocrine manifestations in Joubert syndrome—literature review. AIMS Medical Science, 2023, 10(4): 343-352. doi: 10.3934/medsci.2023027 |
Multiple sclerosis (MS), is a debilitating neurological disease that currently has various treatments, like disease-modifying therapies, monoclonal antibodies, corticosteroids, and hormonal derivatives. Melatonin has several actions, like regulating circadian rhythms, which are usually used for insomnia. This scoping review aimed to explore the efficacy of melatonin, both as a standalone treatment and in conjunction with other drugs, in the management of MS in animal models.
We searched PubMed, Web of Science, EMBASE, and Google Scholar using (“melatonin” OR “melatonin receptor”) AND (“multiple sclerosis”) AND (“animal”). Animal studies that evaluated the effects of melatonin on the development, progression, and outcomes of MS were included. Human studies and other types of studies like case reports were excluded. We used narrative synthesis for reporting the results.
Overall, 21 studies were included, conducted on mice (n = 15) and rats (n = 6). Mostly, studies reported that melatonin led to normal circadian rhythms in animals. Melatonin in doses of both 50 and 100 mg/kg were useful in nociception latency. Melatonin in combination with other drugs like H-89, diisopropylamine dichloroacetate, gibberellins, and IFN-1β improved outcomes, while there was not improved cognition in combination with mesenchymal stem cells. In some tests, male subjects showed significantly better responses. There were controversial results regarding the effects of melatonin on cytokines, but overall, it led to a reduction in proinflammatory cytokines.
Melatonin overall demonstrated favorable outcomes regarding oxidative stress, anti-inflammation, and cytokine levels across various doses. It is recommended conducting systematic reviews and meta-analyses with a larger number of primary studies to provide more comprehensive insights.
Multiple sclerosis (MS) is a chronic autoimmune disease affecting the central nervous system (CNS). It is characterized by inflammation, demyelination, gliosis, and neuronal loss [1]. The symptoms of MS can vary widely among patients, depending on the location and severity of nerve fiber damage in the CNS [1]. Common symptoms include vision impairments such as blurred vision or diplopia, muscle weakness, fatigue, depression, sexual dysfunction, and cognitive problems [1]. The progression of MS is often categorized into four stages, which are clinically isolated syndrome, relapsing-remitting MS (RRMS), secondary progressive MS (SPMS), and primary progressive MS (PPMS) [2].
Several types of treatments have been developed for MS. In this regard, disease-modifying therapies (DMTs) aim to target the immune system, each with its own mechanism of action [3]. First-line treatments include interferon (IFN) beta group, glatiramer acetate (GA) or Copaxone, dimethyl fumarate, teriflunomide, and alemtuzumab [3],[4]. Second-line treatments consist of natalizumab and fingolimod [3],[4]. However, no DMTs have been conclusively proven to affect the long-term prognosis of MS [3],[4].
MS is three times more common in women than men, particularly in those of childbearing age [5]. Hormonal differences, such as testosterone and estrogen levels, may contribute to this higher prevalence in women: prior to puberty, MS rates are almost similar between males and females, whereas with advanced ageing and hormonal fluctuations, women develop MS more frequently than men [6]. So, hormones play roles in MS and research on hormone therapy is ongoing [3],[4].
Melatonin (N-acetyl-5-methoxytryptamine), a natural hormone derived from tryptophan, is primarily synthesized by pinealocytes in the mammalian pineal gland. It is predominantly secreted during darkness [7],[8]. Melatonin and its metabolites play multifaceted roles, including reducing demyelination and promoting remyelination in inflammatory conditions, providing anti-oxidative effects by eliminating reactive oxygen and nitrogen species [7],[8]. Additionally, it has been observed to increase anti-immunomodulatory and anti-inflammatory effects, thereby reducing both chronic and acute inflammation [9]. Melatonin has also been noted for its potential in improving lipid profiles [10].
Animal studies have demonstrated that melatonin can decrease the loss of oligodendrocytes and increase levels of microglia and CD4+ T cells, suggesting its potential role to alter the treatment approaches for neurodegenerative disorders and improve patient quality of life [11]. A systematic review on human studies evaluated the effects of melatonin on MS and showed that it is almost a safe intervention that can improve sleep, fatigue, and cognition in MS [12]. Nevertheless, they recommended further studies due to the limited number of included studies [12]. Other previous review articles shed light on different aspects of melatonin roles in MS [13],[14]. Very recently, Rezmaray et al. conducted a systematic review to investigate the melatonin supplementation efficacy for disease severity and neurobehavioral modification among MS-simulating animal models. In this work, we aim to assess the melatonin mechanism in ameliorating the disease and also consider the effects of intrinsic melatonin level fluctuations and outcomes among animals (Figure 1).
Thus, this scoping review aimed to evaluate the potential of melatonin, as combined with other medications or endogenous ones, on MS in animal models.
The study was in accordance with preferred reporting items for systematic reviews and meta-analyses extension for scoping reviews [15].
We conducted a search in PubMed, Web of Science, EMBASE, and Google Scholar using a systematic strategy on November 23, 2023. We systematically searched the five aforementioned databases using the terms: (“melatonin” OR “melatonin receptor”) AND (“multiple sclerosis” OR “disseminated sclerosis” OR “acute fulminating”) AND (animals or EAE). The results were imported into EndNote software for duplicate removal.
The inclusion criteria were as follows: (1) Peer-reviewed articles reporting different effects of melatonin on MS occurrence, progression, or outcomes in animal models; (2) studies examining changes in melatonin levels in animals during various phases of the disease; and (3) research involving animals receiving melatonin supplements, either alone or in conjunction with other medications. Studies were excluded if they were other types of studies, such as case reports and case series, reviews, and conference proceedings, articles not published in English, articles without access to full-text articles published before 1990, retracted articles, and articles lacking information on the number of samples studied or study outcomes. Two authors independently reviewed the titles, abstracts, and full texts of the documents, by eligibility criteria. Any discrepancy was resolved by discussion.
Two authors independently reviewed the selected articles and then imported the data into a Microsoft Excel sheet. The extracted information was the first author's name, year of publication, MS simulation induction, sex distribution, phase of disease, disease duration, concomitant drugs and dosages, treatment duration, and outcomes for each study. We used discussion between those authors to resolve discrepancies.
We used a narrative synthesis for reporting the results of the study due to the high heterogeneity between studies.
Overall, 519 studies were found during literature search. Following the removal of 97 duplicates, 422 remained. In title/abstract screening, 401 studies were excluded. The full text of the remaining 21 studies were evaluated for eligibility and all of them met the eligibility criteria. Finally, 21 studies were included in the qualitative synthesis [16]–[36] (Figure 2).
Studies were published between 2015 and 2023. Seven studies were case-controls, two were cohorts, seven were experimental, and five were other types of observational studies. Eight studies were conducted in Iran, two in the countries of Mexico, Saudi Arabia, Spain, and Ukraine, and one in each of the countries of Canada, China, Egypt, Taiwan, and the United States. Fifteen studies were conducted on mice, and the remaining six were conducted on rats. The treatment duration was between three days and nine weeks. Concomitant medications included luzindole, cuprizone, IFN, GA, and methylprednisolone (Table 1).
Different methods for induction were used in the studies. Cuprizone was among the most applied approaches for simulating experimental allergic encephalomyelitis (EAE). Cuprizone was administrated whether at 0.2%, 0.2 mL orally, or 400 mg/kg/day. All the experiments took 5-week cuprizone administration to reach the EAE approvable extent, except Labunets et al. [27] that took three weeks. Also, in the article by Abo Taleb et al. [16], cuprizone induced the demyelination stage for five weeks, followed by spontaneous remyelination stage for four weeks (Table 2).
Pretreatment [3 days after myelin oligodendrocyte glycoprotein (MOG) injection] and post-treatment (day 10 after EAE induction) of melatonin was used in the study by Wen et al. [17]. Primary microglia cells were pretreated with melatonin at concentrations of 0.3 and 3 mM starting 30 min before and 6 h after the start of lipopolysaccharide (LPS, 100 ng/mL) exposure. Treatment of LPS-stimulated microglia with melatonin at a concentration of 0.3 mM did not significantly affect inducible nitric oxide (iNOS) expression. However, treatment with melatonin at 3 mM significantly inhibited the production of iNOS [17].
Animals pretreated with melatonin spent a similar time in the target quadrant proximity as compared to the control group [34]. In another study, the prophylactic and therapeutic treatments with melatonin significantly protected against EAE, but not as potently as daily administration. Again, levels of IL-1a, IL-6, IL-2, IFN, TNF, IL-17α, and IL-22 were reduced with melatonin and IL-10 production was significantly augmented. However, melatonin could not change the onset of MS [28].
Nine studies showed that the circadian rhythms of mice who received melatonin were normal (i.e., 12/12 h light/dark cycle) [16],[18],[20],[21],[23],[30],[33],[34],[36]. In the study by Ghareghani et al., 24 h/day bright light and 24 h/day darkness were defined as constant light and darkness, respectively. The mice exposed to constant light and darkness experienced that condition for 3 days, followed by a rest day of normal 12/12 h light/dark [22]. Five weeks of cuprizone feeding for mice showed enhanced melatonin levels compared with controls. Constant light and darkness significantly decreased and increased melatonin levels, respectively. No significant changes were reported in melatonin level with the administration of melatonin at 80 mg/kg and luzindole at 30 mg/kg. The oligodendrocyte precursor cells (OPCs) were significantly higher in vehicle mice than in control mice (p < 0.05). Immature OPCs did not increase response to constant light or luzindole in comparison to those in the vehicle group; however, the maturation of OPCs into oligodendrocytes increased significantly in response to constant light or luzindole in comparison to that in the vehicle group (p < 0.001 and p < 0.05, respectively). Despite mature oligodendrocytes, immature OPCs were significantly reduced by constant darkness but not by melatonin in comparison to the vehicle group (p < 0.01) [22] (Table 1).
Study ID | Study type | Country | Subjects | Sex and sample size | Interventions | Treatment duration | Results |
Alghamdi et al. 2020 | Observational | Saudi Arabia | Mice | All male = 30 | Cuprizone (400 mg/kg) and melatonin (80 mg/kg) daily | 5 weeks | Enhanced the memory defects, increased the nociception latency, antagonists for toxic effects of cuprizone on motor activity, enhanced memory outcomes |
Dokoohaki et al. 2017 | Observational | Iran | Lewis rats | All female = 18 | Methylprednisolone 30 mg/kg | 6 days | Decrease in melatonin level (p < 0.05), diminished IFN-γ (p < 0.01), increased serum IL-4 levels (p < 0.01) |
Ghareghani et al. 2022 | Case control | Iran | C57BL/6 mice | All female = 32 | Melatonin (10 mg/kg/day) DADA (50 mg/kg/day, orally) | 10 days | Improved neurological disability scores for melatonin, combination of melatonin + DADA improved neurological disability scores more than melatonin alone, significant increase of IL-4 and IL-10 levels, and reduction in IL-1B and TNF levels for combination therapy (p < 0.000001) |
Ghareghani et al. 2023 | Case control | Canada | C57BL/6 J wild-type mice | All males | 30 mg/kg Luzindole, 80 mg/kg melatonin | 5 weeks | Reduction in total Olig2 + oligodendroglia (p = 0.051), neural stem/progenitor cells proliferation inhibition, no influence on Olig2 + /SOX2 + cells, decreased number in melatonin did not cause modified percentage of Ly6Clow monocytes |
Gharib et al. 2022 | Case control | Egypt | Mice | All males = 50 | Cuprizone 0.2% MSC-MVs 0.2 mg/kg, A single melatonin 10 mg/kg for three weeks | 3 weeks | Increase in the duration of maintained balance, melatonin did not change the cognitive performance but MSC-MVs did. |
González et al. 2021 | Case control | Mexico | Sprague-Dawley rats | All male = 128 | IFN-® 1b (dosed at 8000 U), every third day, intramuscularly Glatiramer acetate 0.50 mg/kg/day, subcutaneously Melatonin at 10 mg/kg/day, i.p |
12 days | The least level of TNF was shown in melatonin + EAE group that was 0 pg/mL |
Ghareghani et al. 2018 | Case control | Iran | C57BL/6 mice | All female = 24 | 10 mg/kg/day i.p | 13 days | Decrease in mean clinical scores (p < 0.05), procalcitonin reduced augmented concentrations of 25-hydroxyvitamin D (p < 0.05) |
Jand et al. 2022 | Observational | Iran | KP. C57BL/6 mice | All female = 68 | Melatonin 0.1, 1.0, 5.0, 10 mg/kg | 9 days | Melatonin significantly decreased the EAE clinical scores, kynurenine pathway regulatory enzyme mRNA expression reduction inhibits the enzyme Nicotinamide N-Methyltransferase overexpression (p < 0.001) |
Labunets et al. 2023 | Case control | Ukraine | Mice | Young = 79 Aged = 79 All female |
Melatonin at 6 p.m. daily, 1 mg/kg, i.p | Days of 7, 21, and 60 | Astrocyte activation in the young and aging mice reduced the percentage of neurons with destructive variations, behavioral reactions in young and aging mice improved level of thymulin in blood, diminished only in younger mice (p < 0.05) |
Labunets et al. 2021 | Experimental | Ukraine | Mice | NA | Melatonin 1 mg/kg, at 18:00, daily, Cuprizone diet 0.5 × 106 cells, once intravenously | 11 days | Cuprizone elevated the malondialdehyde levels and reduced the activity of antioxidant enzymes in the brains of mice; there was a decrease observed in locomotor, emotional, and orientational-exploratory activity, and muscle tone |
Álvarez-Sánchez et al. 2015 | Experimental | Spain | C57BL/6 mice | All female | 80 mg/kg melatonin daily | NA | Reduced the severity of the disease (p < 0.001), diminished the incidence of EAE (p < 0.05), reduced frequencies of absolute CD4+ T macrophages (p < 0.05) |
Chen et al. 2016 | Experimental | Taiwan | C57BL/6 mic | All female | 200 mg/kg injection | Days 9, 11, and 13 after EAE induction | Improvement in clinical scores (p < 0.01), leukocyte infiltration reduction, inhibiting the T-cell proliferation, and reduced IL-10 and IL-17 expressions |
Long et al. 2018 | Experimental | China | C57BL/6 mice | All female | Melatonin 10 mg/kg, i.p | Day 0 post-immunization of EAE | Decrease in neurological deficit scores at the peak stage of EAE (p < 0.05); decrease in ROS concentrations (p < 0.05) |
Sharif et al. 2017 | Experimental | Iran | Wistar rats | All males | Melatonin (50 and 100 mg/kg/side), H-89 (10 micro-M) intra-hippocampally 30 min before each day of training | 4 days | Improved escape latency compared with H-89 alone (p = 0.008); low-dose melatonin pretreatment significantly decreased H-89-induced cytochrome c release compared to control group (p < 0.05), and high-dose melatonin completely impeded such event by leaving no significant difference compared with control group |
Ghareghani et al. 2018 | Experimental | Iran | C57BL/6 mice | All females = 40 | 10 mg/kg/day baclofen 10 mg/kg/day melatonin |
10 days | Reestablishing original normal levels of IL-4 and IFN-γ in combination therapy (p < 0.001), increase in antioxidant enzymes catalase activity in combination (p < 0.001) |
Abo Taleb et al. 2019 | Case control | Saudi Arabia | Mice | Females = 39 Males = 39 |
Cuprizone 400 mg/kg/day oral+ melatonin 80 mg/kg/day i.p at 12 p.m. | 9 weeks | Reduced MDA in males, increased CAT, SOD, GPx, and GSH, improvement in TNF and IL-1B levels |
Escribano et al. 2022 | Observational | Spain | Rats | All males = 25 | Melatonin 1 mg/kg oral 5 days a week | 51 days | Reduction in oxidative stress biomarkers levels (p < 0.001), reduction in EAE severity (p < 0.001) |
Ghareghani et al. 2016 | Observational | Iran | Rats | All females = 15 | Melatonin 10 mg/kg | 18 days | Increase in serum lactate (p < 0.01) and number of infiltrated cells (p < 0.01) |
Gabriel Ortíz et al. 2022 | Experimental | Mexico | Rats | All males = 48 | Melatonin 20 mg/kg of body weight/day, GA 10 mg/kg of body weight/day, IFN-b1b 9000 IU/kg of body weight every other day | 20 days | Reduced cumulative index score, reduced levels of IL1β, IL-6, and TNF-a, reduced MDA+4-OHA (p < 0.05) |
Vakilzadeh et al. 2015 | Cohort | Iran | Mice | N = 20 | Cuprizone, melatonin 50 mg/kg, melatonin 100 mg/kg | 7 days | Increase in moved distances for melatonin 50 mg/kg (p < 0.01) and 100 mg/kg (p < 0.001) |
Wen et al. 2016 | Cohort | United States | Mice | All females | 28 days | Melatonin 20 mg/kg i.p | Attenuated clinical severity (p < 0.05), less CD4+ positive cells (p < 0.001) |
Note: CAT: Catalase; SOD: Superoxide dismutase; GPx: Glutathione peroxidase; GSH: Glutathione; MDA: Malondialdehyde; EAE: Experimental autoimmune encephalomyelitis; GA: Glatiramer acetate; MDA: Malondialdehyde; 4-OHA: 4-hydroxyalkenals; i.p: Intraperitoneally; DADA: Diisopropyl amine dichloroacetate; MSC-MVs: Mesenchymal stem cells microvesicles; TNF: Tumor necrosis factor; CSF: Cerebrospinal fluid; ROS: Reactive oxygen species; IU: International unit.
Study ID | Approach | Simulation evidence | Duration |
Abo Taleb et al. 2019 | 400 mg/kg/day cuprizone (oral gavage with 1% CMC) | Progressive decline in locomotor activity | 5 weeks |
Vakilzadeh et al. 2016 | Diet containing 0.2 % cuprizone mixed into ground standard rodent chow | NA | 5 weeks |
Wen et al. 2016 | Injected subcutaneously with 200 µg of MOG 35–55 peptide emulsified in complete Freund's adjuvant containing 500 µg Mycobacterium tuberculosis | When an animal showed a clinical score ≥ 0.5 | 7 weeks |
Escribano et al. 2022 | 100 µL of a solution containing 150 µg of MOG | NA | 14 days |
Ghareghani et al. 2017 | Subcutaneously over the flank with 200 µL of a 1:1 mixture of 1 g GPSC in 1 mL PBS and complete Freund's adjuvant (CFA, Sigma-Aldrich, USA) and 1 mg/mL Mycobacterium tuberculosis bacteria enriched | NA | NA |
Ortíz et al. 2022 | Sterile physiological saline solution and emulsified with 50 µL of complete Freund's adjuvant containing 1 mg/mL heat-killed Mycobacterium tuberculosis strain H37Ra | NA | NA |
Ghareghani et al. 2022 | Immunization solution containing the immunogenic epitope MOG-35-55 was emulsified with complete Freund's adjuvant (CFA, Sigma-Aldrich) and Mycobacterium tuberculosis, and was injected subcutaneously over the flank | NA | NA |
Ghareghani et al. 2023 | Cuprizone (0.2% wt/wt) was incorporated into standard irradiated ground rodent chow | NA | NA |
Gharib et al. 2022 | Ad libitum and fed 0.2% (w/w) cuprizone (Sigma) in ground breeder chow | Behavioral stress | 5 weeks |
Ramos González et al. 2021 | 150 µL of a homogenate containing 25 µL of pig spinal cord homogenate, 25 µL of pig brain homogenate, and 100 µL of Freund's complete adjuvant | EAE, achieving at least grade 2 of the disease | NA |
Ghareghani et al. 2018 | Emulsified in complete Freund's adjuvant (CFA, Sigma-Aldrich), enriched Mycobacterium tuberculosis bacteria | NA | NA |
Jand et al. 2022 | Injection of MOG35-55, 300 ng of pertussis toxin (List Biological Labs, Campbell, CA, USA) intraperitoneally, into all animals, on days 0 and 2 | NA | NA |
Labunets et al. 2023 | Cuprizone at 0.2% (w/w) | NA | 3 weeks |
Álvarez-Sánchez et al. 2015 | Immunized subcutaneously in each hind leg with 100 µg of MOG35–55 (Cambridge Research Biochemicals), emulsified in CFA (Sigma) containing 50 µg of heat-killed Mycobacterium tuberculosis | NA | NA |
Chen et al. 2016 | MOG was purified to greater than 95% purity using high-performance liquid chromatography. Each C57BL/6 mouse was immunized with 100 µg of MOG in 100 µL of an emulsion of complete Freund's adjuvant and 400 µg of Mycobacterium tuberculosis H37Ra | NA | NA |
Long et al. 2018 | Injected subcutaneously at one side of the flank with 250 µg MOG35–55 peptide in complete Freund's adjuvant and 64 mg/mL of heat-killed Mycobacterium tuberculosis. At 0 h and 48 h after MOG injection, 500 ng pertussis toxin in 100 µL PBS was injected intraperitoneally | Neurological deficit | NA |
Ghareghani et al. 2018 | Immunized with MOG | Clinical signs of the disease | NA |
Dokoohaki et al. 2017 | Injected subcutaneously over the flank with 200 µL of a 1:1 mixture of 1 g of GPSC in 1 mL PBS and complete Freund's adjuvant and 1 mg/mL enriched Mycobacterium tuberculosis bacteria | Clinical signs | NA |
Alghamdi et al. 2020 | 0.2 mL cuprizone orally | NA | 5 weeks |
Note: MOG: Myelin oligodendrocyte glycoprotein; GPSC: Guinea pig spinal cord; PBS: Polybutylene succinate; EAE: Experimental autoimmune encephalomyelitis.
The concentration of 3 mM significantly reduced iNOS production [27]. Also, melatonin blocked the expression of reactive oxygen species generation in reactive microglia [27]. Melatonin at both 50 and 100 mg/kg significantly increased the nociception latency (p < 0.01). Moreover, melatonin at 100 mg/kg significantly diminished the mean number of TUNEL-positive cells in the medial region of the corpus callosum compared to the cuprizone group (p < 0.01) [36]. Melatonin at 50 mg pretreatment significantly decreased H-89-induced cytochrome C release compared to control group (p < 0.05), and melatonin at 100 mg showed no significant changes [34] (Table 1).
Melatonin treatment, alone (p < 0.01) or in combination with H-89 (p < 0.001), remarkably augmented target quadrant occupancy in comparison with H-89-treated animals [34]. Combination of diisopropylamine dichloroacetate (DADA) and melatonin further amended melatonin's effects, and it led to a positive effect on diminishing the disability. Another positive interaction occurred in the course of decreasing the loss of oligodendrocytes assessed by levels of myelin basic protein (MBP) and myelin-associated oligodendrocytic basic protein (MOBP) (p < 0.0001) [21]. Melatonin had significant influence on lactate reduction (p < 0.01) and DADA positive interaction with melatonin for that effect (p = 0.01) [21].
Melatonin did not lead to cognitive enhancement, contrary to the influence of mesenchymal stem cells macrovesicles (MSC-MVs) [23]. To some extent, it inhibited the positive effect of MSC-MVs on cognition. In Gharib et al. [23], melatonin significantly improved locomotor activity at the same extent as MSC-MVs+melatonin. Melatonin and MSC-MVs both improved psychomotor status compared with EAEs but there was synergism between them (p < 0.0001) [23]. Cognitive abilities improved significantly with MSC-MVs but decreased significantly with melatonin, and the combination made no difference compared with controls and EAE [23]. Tissue malondialdehyde (MDA) decreased significantly compared with EAE in both melatonin and with MSC-MVs. Tissue glutathione (GSH) in both melatonin and MSC-MVs increased significantly compared with EAE but it was still lower than controls [23].
Therapeutic intervention with methylprednisolone 30 mg/kg resulted in a highly significant decrease (p < 0.001) in mean clinical scores [32]. Methylprednisolone caused a significant decrease (p < 0.01) in serum IFN-γ and increase in IL-4 [32].
Therapy with GA and IFN-1β both non-significantly improved cumulative disease index score compared with EAE [20]. Melatonin alone or in combination with GA or IFN-1β significantly ameliorated the disease clinical score (p < 0.05) [20]. Treatment with melatonin alone caused significant reduced plasma levels of TNFα, IL-1β, and IL-6 compared with EAE (p < 0.05) [20]. Melatonin, however, did not alter the amount of IL-1β after EAE compared with controls [20].
In midbrain, levels of nitrite–nitrate significantly decreased compared with EAE in all medications except GA and melatonin combination, which was even higher than controls [20]. End products of lipid peroxidation significantly decreased compared with EAE in all combinations in midbrain and motor cortex except for IFN-β alone, which was significantly higher compared with controls and unchanged compared with EAE in motor cortex [20].
Synergistic effects were shown in the combination of melatonin and H-89 as the mean of time spent in target quadrant (p < 0.001) compared with H-89 alone. Melatonin also inhibited the H-89-induced mitochondrial dysfunction (p < 0.0001). Melatonin at 50 and 100 g/kg alone or in combination with H-89 significantly decreased the mitochondrial swelling in comparison with H-89 [34]. Regarding escape latency, the main effects of melatonin (p = 0.0001) and the combination of H-89 and melatonin (p = 0.008) were significant, while the effect of only H-89 was not remarkable (p < 0.13). On the other hand, the main effects of H-89 (p < 0.02), melatonin (p = 0.0001), and the combination of H-89 and melatonin (p = 0.004) were significant for traveled distance [34]. Furthermore, combination groups showed a significant decrease in both time and distance of finding a hidden platform compared with the corresponding H-89 group (p < 0.0001) [34].
Baclofen did not make any significant changes on IL-4 and IFN-γ levels; however, melatonin significantly increased IL-4 and reduced IFN-γ [31]. Also, mean cumulative clinical score decreased significantly with melatonin compared with EAE [31]. Baclofen and melatonin individually reduced MDA (p < 0.05) and the combination was synergetic (p < 0.01) [31].
MS treatment with methylprednisolone caused a significant decrease in the levels of serum melatonin, whereas the decrease in polybutylene succinate (PBS)-treated EAE group was not significant [32]. Methylprednisolone injection on day 1 caused a significant (p < 0.05) reduction of melatonin levels on day 2, which was further decreased (p < 0.01) on day 3, after a second injection on day 2 [32] (Table 1).
Melatonin led to a significant improvement in total distance movement (p = 0.0003) and grip strength (p < 0.0001) in male mice compared with females [16].
Melatonin diminished numbers of ratio of CD3+CD4+/CD3+CD8+ cells, independently of the influence of cuprizone, in both aged and young mice. The number of activated macrophages decreased after melatonin supplementation for both ages, reaching that of controls. After melatonin administration, MDA content decreased to that of controls in young mice and even lower compared with the control group among aging ones [27] (Table 1).
Application of melatonin for the last 7 days of cuprizone feeding did not change IL-6 level compared to the cuprizone group [36]. IL-4 levels in PBS- and melatonin-treated EAE rats were significantly lower (p < 0.001) than those of controls. The ratio of IFN-γ/IL-4, which acts as an indicator of Th-1/Th-2, was found to be significantly higher in PBS- (p < 0.001) and melatonin-treated (p < 0.001) subjects [19]. DADA exhibited no changes in serum levels of IL-1B, TNF, IL-4, and IL-10, while melatonin reduced the proinflammatory and enhanced the anti-inflammatory serum levels in mice [21]. There were no significant changes in the percentages of CD69+ cells within the CD4, CD8, or CD19 lymphocyte subset, pointing that melatonin primarily plays its role independently of T-cells count differentiation. Th1 cells in the CNS were slightly lower, and there were no significant differences in the percentages of these cells in either the spleens or CNS of the melatonin-treated and solvent-treated mice [29] (Table 1).
Our findings showed that melatonin administration contributed to the restoration of normal circadian rhythms in animals. Notably, both 50 and 100 mg/kg doses of melatonin demonstrated efficacy in enhancing nociception latency. Combining melatonin with other substances, such as H-89, diisopropylamine dichloroacetate, gibberellins, and IFN-1β, yielded varying effects, with no observed improvement in cognition when combined with mesenchymal stem cells. Interestingly, male subjects exhibited a significantly more favorable response in certain tests. Some results differed for aged and young animals, which requires more attention for future studies. Findings regarding the impact of melatonin on cytokines were contradictory; yet, on the whole, melatonin administration tended to reduce proinflammatory cytokines.
There are several suggested mechanisms for MS to lead to disruptions in circadian rhythms in humans. In this regard, ganglion cell atrophy and compromised integrity of the retino-hypothalamic tract result in the disruption of central clock synchronization by light/dark signals [37]. Also, there are disruptions in the rhythms of melatonin secretion, potentially contributing to sleep disturbances and fatigue in individuals with MS, that may be attributed to altered central clock rhythmicity [37]. Peripheral rhythm changes in MS have been observed for certain metabolic hormones like leptin and corticosterone. Together with variations in gut microbiome composition, these changes might contribute to alterations in metabolic functions in MS. Furthermore, disturbances in immune rhythms could potentially contribute to immune dysregulation in MS [37]. Notably, MS is associated with genetic polymorphisms within various clock genes, underscoring the role of genetic factors in MS predisposition [37]. Human studies showed that melatonin levels are lower in patients with MS compared with controls [13]; this can also be one of the explanations for circadian rhythms in MS. In our scoping review on animal studies, melatonin led to normal circadian rhythms in most subjects, whether it was administered as a single therapy or combined with other medications. In the same way as with our findings, a systematic review on observational and interventional clinical studies concluded that melatonin could affect inflammation and oxidative stress, but its application in clinical practice and its impacts on sleep, cognition, and fatigue in patients with MS need further investigations [12].
We revealed that melatonin can be useful in the different doses used in animal studies, like 50 and 100 mg/kg, through its effects on iNOS expression and OPCs. Similarly, previous studies on the roles of melatonin in MS showed that elevation of proinflammatory cytokines triggers an excessive generation of reactive oxygen and nitrogen species. They initiate the activation of nuclear kappa B factor in various cells within the CNS, including T cells, microglia, macrophages, astrocytes, oligodendrocytes, and neurons. Nuclear kappa B factor positively regulates the expression of factors implicated in the pathogenesis of MS, such as TNF-α, iNOS, IL-1α, vascular adhesion molecule 1, and several growth factors [14],[38].
Our findings showed that melatonin administration attenuated H-89-induced memory deficit and lowered escape latency and distance traveled to a control level. To the best of our knowledge, this mechanism has not been described in previous in vitro or clinical studies, and it was solely mentioned in the study by Sharif and colleagues [34]. Together, these data imply that melatonin could reverse the H-89-induced memory deficits, inferring a protective role of melatonin. Regarding other medications, one of our included studies showed corticosteroids significantly reduced melatonin in both rats and humans with MS [32]. In this regard, cortisol, a glucocorticoid hormone, has also been associated with the progression and severity of MS; reduced cortisol levels are associated with feelings of fatigue and urinary dysfunction, whereas elevated levels have been associated with increased anxiety and depression [39].
The study by Gharib et al., which was included in our scoping review, showed that melatonin did not lead to improvements in cognition, in spite of the positive effects of MSCs [23]. Existing evidence shows the combined effects of melatonin and MSCs on different diseases like MS [40]. In detail, melatonin has the capacity to decrease the release of inflammatory cytokines, boost the proliferative capabilities of MSCs, and prevent the apoptosis of MSCs [41]. Moreover, given the increasing interest in melatonin, it also plays roles in regulating the expression of genes related to both reactive oxygen species generation and antioxidant activity [41].
The study by Farhadi et al. on patients with MS and healthy controls showed a decreased level of melatonin and increased levels of cytokines like TNF-α, which can highlight the roles of melatonin in MS [42]. In accordance with the mentioned findings, we also found that levels of different cytokines like IL-1a, IL-6, IL-2, IFN, TNF, IL-17α, and IL-22 decreased in those receiving melatonin.
To the best of our knowledge, this is a pioneer scoping review conducted on animal studies to evaluate the effects of melatonin, alone or combined with other drugs, on outcomes of animals with MS. So, the findings of this study can be used for designing future animal studies or randomized controlled trials on humans to assess their effects. It can also shed light on the mechanisms of action of melatonin on MS and possible use as an adjuvant treatment in the future. However, the study has several limitations that should be considered while interpretating its findings. First, there is a possibility that our search strategy may have overlooked certain studies, although we performed searches in several databases. Second, as it was a scoping review, we did not perform a quality assessment of included studies. This point can be considered in following systematic reviews. Third, we were unable to perform a meta-analysis across diverse study designs and outcomes, so we used a narrative approach to report the findings. Fourth, there is a likelihood of publication and reporting bias, although we could not quantify such biases. Fifth, most details about the methodological aspects of animal studies, which were included, were inadequately reported. Sixth, the studies were conducted on mice and rats, while the effects on other types of animals were not evaluated. Finally, because of the fact that the study was conducted on animal studies, the findings cannot be generalized to humans. So, the application of these findings in clinical settings needs to be evaluated in future clinical trials and systematic reviews.
Melatonin, in different doses and in combination with other drugs or alone, was shown to have generally improved results in terms of oxidative stress, anti-inflammation, and cytokine levels. Considering the limitations, future primary studies should be conducted on other types of animals. Moreover, systematic reviews and meta-analysis with a higher number of studies are recommended to be performed.
Heliya Bandehagh: Conceptualization and study design; Heliya Bandehagh and Farnaz Gozalpour: Search; Farnaz Gozalpour, Ali Mousavi, Mahdi Hemmati Ghavshough: Data acquisition; Heliya Bandehagh and Ali Mousavi: Data analysis/interpretation; Mahdi Hemmati Ghavshough: Supervision/mentorship; All authors: Drafting of the manuscript. All authors had a critical role in revision of the manuscript for important intellectual content. The final manuscript was read and approved by all authors.
The authors declare that they have not used Artificial Intelligence (AI) tools in the creation of this article.
[1] |
Jakimovski D, Bittner S, Zivadinov R, et al. (2023) Multiple sclerosis. Lancet 403: 183-202. https://doi.org/10.1016/S0140-6736(23)01473-3 ![]() |
[2] |
Thompson AJ, Baranzini SE, Geurts J, et al. (2018) Multiple sclerosis. Lancet 391: 1622-1636. https://doi.org/10.1016/S0140-6736(18)30481-1 ![]() |
[3] |
McGinley MP, Goldschmidt CH, Rae-Grant AD (2021) Diagnosis and treatment of multiple sclerosis: a review. JAMA 325: 765-779. https://doi.org/10.1001/jama.2020.26858 ![]() |
[4] |
Hauser SL, Cree BAC (2020) Treatment of multiple sclerosis: a review. Am J Med 133: 1380-1390.e2. https://doi.org/10.1016/j.amjmed.2020.05.049 ![]() |
[5] |
Qian Z, Li Y, Guan Z, et al. (2023) Global, regional, and national burden of multiple sclerosis from 1990 to 2019: findings of global burden of disease study 2019. Front Public Health 11: 1073278. https://doi.org/10.3389/fpubh.2023.1073278 ![]() |
[6] |
Català-Senent JF, Andreu Z, Hidalgo MR, et al. (2023) A deep transcriptome meta-analysis reveals sex differences in multiple sclerosis. Neurobiol Dis 181: 106113. https://doi.org/10.1016/j.nbd.2023.106113 ![]() |
[7] |
Cardinali DP (2019) Melatonin: clinical perspectives in neurodegeneration. Front Endocrinol 10: 480. https://doi.org/10.3389/fendo.2019.00480 ![]() |
[8] |
Gunata M, Parlakpinar H, Acet HA (2020) Melatonin: a review of its potential functions and effects on neurological diseases. Rev Neurol 176: 148-165. https://doi.org/10.1016/j.neurol.2019.07.025 ![]() |
[9] |
Hardeland R (2018) Melatonin and inflammation—story of a double-edged blade. J Pineal Res 65: e12525. https://doi.org/10.1111/jpi.12525 ![]() |
[10] |
Loloei S, Sepidarkish M, Heydarian A, et al. (2019) The effect of melatonin supplementation on lipid profile and anthropometric indices: a systematic review and meta-analysis of clinical trials. Diabetes Metab Syndr 13: 1901-1910. https://doi.org/10.1016/j.dsx.2019.04.043 ![]() |
[11] |
Razmaray H, Nasiri E, Vakilipour P, et al. (2024) The effects of melatonin supplementation on neurobehavioral outcomes and clinical severity in rodent models of multiple sclerosis; a systematic review and meta-analysis. Inflammopharmacology 32: 927-944. https://doi.org/10.1007/s10787-023-01414-7 ![]() |
[12] |
Morsali S, Sabahi Z, Kakaei J, et al. (2023) Clinical efficacy and safety of melatonin supplementation in multiple sclerosis: a systematic review. Inflammopharmacology 31: 2213-2220. https://doi.org/10.1007/s10787-023-01271-4 ![]() |
[13] |
Skarlis C, Anagnostouli M (2020) The role of melatonin in multiple sclerosis. Neurol Sci 41: 769-781. https://doi.org/10.1007/s10072-019-04137-2 ![]() |
[14] |
Muñoz-Jurado A, Escribano BM, Caballero-Villarraso J, et al. (2022) Melatonin and multiple sclerosis: antioxidant, anti-inflammatory and immunomodulator mechanism of action. Inflammopharmacology 30: 1569-1596. https://doi.org/10.1007/s10787-022-01011-0 ![]() |
[15] |
Tricco AC, Lillie E, Zarin W, et al. (2018) PRISMA Extension for Scoping Reviews (PRISMA-ScR): checklist and explanation. Ann Intern Med 169: 467-473. https://doi.org/10.7326/M18-0850 ![]() |
[16] |
Abo Taleb HA, Alghamdi BS (2020) Neuroprotective effects of melatonin during demyelination and remyelination stages in a mouse model of multiple sclerosis. J Mol Neurosci 70: 386-402. https://doi.org/10.1007/s12031-019-01425-6 ![]() |
[17] |
Wen J, Ariyannur PS, Ribeiro R, et al. (2016) Efficacy of N-acetylserotonin and melatonin in the EAE model of multiple sclerosis. J Neuroimmune Pharmacol 11: 763-773. https://doi.org/10.1007/s11481-016-9702-9 ![]() |
[18] |
Escribano BM, Muñoz-Jurado A, Caballero-Villarraso J, et al. (2022) Protective effects of melatonin on changes occurring in the experimental autoimmune encephalomyelitis model of multiple sclerosis. Mult Scler Relat Disord 58: 103520. https://doi.org/10.1016/j.msard.2022.103520 ![]() |
[19] |
Ghareghani M, Dokoohaki S, Ghanbari A, et al. (2017) Melatonin exacerbates acute experimental autoimmune encephalomyelitis by enhancing the serum levels of lactate: a potential biomarker of multiple sclerosis progression. Clin Exp Pharmacol Physiol 44: 52-61. https://doi.org/10.1111/1440-1681.12678 ![]() |
[20] |
Ortíz GG, Briones-Torres AL, Benitez-King G, et al. (2022) Beneficial effect of melatonin alone or in combination with glatiramer acetate and interferon β-1b on experimental autoimmune encephalomyelitis. Molecules 27: 4217. https://doi.org/10.3390/molecules27134217 ![]() |
[21] |
Ghareghani M, Farhadi Z, Rivest S, et al. (2022) PDK4 inhibition ameliorates melatonin therapy by modulating cerebral metabolism and remyelination in an EAE Demyelinating mouse model of multiple sclerosis. Front Immunol 13: 862316. https://doi.org/10.3389/fimmu.2022.862316 ![]() |
[22] |
Ghareghani M, Pons V, Laflamme N, et al. (2023) Inhibiting nighttime melatonin and boosting cortisol increase patrolling monocytes, phagocytosis, and myelination in a murine model of multiple sclerosis. Exp Mol Med 55: 215-227. https://doi.org/10.1038/s12276-023-00925-1 ![]() |
[23] | Gharib D, Rashed L, Yousuf A, et al. (2022) Therapeutic effect of microvesicles derived from BM-MSCS transplantation and/or melatonin in cuprizone model of multiple sclerosis: a pharmacodynamic biochemical assay. Egypt J Chem 65: 153-169. |
[24] |
González EJR, Jirano LJ, Martínez DZG, et al. (2021) A comparative study of melatonin and immunomodulatory therapy with interferon beta and glatiramer acetate in a mouse model of multiple sclerosis. Neurología 36: 262-270. https://doi.org/10.1016/j.nrleng.2018.01.004 ![]() |
[25] |
Ghareghani M, Scavo L, Arnoult D, et al. (2018) Melatonin therapy reduces the risk of osteoporosis and normalizes bone formation in multiple sclerosis. Fundam Clin Pharmacol 32: 181-187. https://doi.org/10.1111/fcp.12337 ![]() |
[26] |
Jand Y, Ghahremani MH, Ghanbari A, et al. (2022) Melatonin ameliorates disease severity in a mouse model of multiple sclerosis by modulating the kynurenine pathway. Sci Rep 12: 15963. https://doi.org/10.1038/s41598-022-20164-0 ![]() |
[27] |
Labunets I, Rodnichenko A, Savosko S, et al. (2023) Reaction of different cell types of the brain on neurotoxin cuprizone and hormone melatonin treatment in young and aging mice. Front Cell Neurosci 17: 1131130. https://doi.org/10.3389/fncel.2023.1131130 ![]() |
[28] |
Álvarez-Sánchez N, Cruz-Chamorro I, López-González A, et al. (2015) Melatonin controls experimental autoimmune encephalomyelitis by altering the T effector/regulatory balance. Brain Behav Immun 50: 101-114. https://doi.org/10.1016/j.bbi.2015.06.021 ![]() |
[29] |
Chen SJ, Huang SH, Chen JW, et al. (2016) Melatonin enhances interleukin-10 expression and suppresses chemotaxis to inhibit inflammation in situ and reduce the severity of experimental autoimmune encephalomyelitis. Int Immunopharmacol 31: 169-177. https://doi.org/10.1016/j.intimp.2015.12.020 ![]() |
[30] |
Long T, Yang Y, Peng L, et al. (2018) Neuroprotective effects of melatonin on experimental allergic encephalomyelitis mice via anti-oxidative stress activity. J Mol Neurosci 64: 233-241. https://doi.org/10.1007/s12031-017-1022-x ![]() |
[31] |
Ghareghani M, Zibara K, Sadeghi H, et al. (2018) Spasticity treatment ameliorates the efficacy of melatonin therapy in Experimental Autoimmune Encephalomyelitis (EAE) mouse model of multiple sclerosis. Cell Mol Neurobiol 38: 1145-1151. https://doi.org/10.1007/s10571-018-0580-y ![]() |
[32] |
Dokoohaki S, Ghareghani M, Ghanbari A, et al. (2017) Corticosteroid therapy exacerbates the reduction of melatonin in multiple sclerosis. Steroids 128: 32-36. https://doi.org/10.1016/j.steroids.2017.10.006 ![]() |
[33] |
Alghamdi BS, AboTaleb HA (2020) Melatonin improves memory defects in a mouse model of multiple sclerosis by up-regulating cAMP-response element-binding protein and synapse-associated proteins in the prefrontal cortex. J Integr Neurosci 19: 229-237. https://doi.org/10.31083/j.jin.2020.02.32 ![]() |
[34] |
Sharif R, Aghsami M, Gharghabi M, et al. (2017) Melatonin reverses H-89 induced spatial memory deficit: involvement of oxidative stress and mitochondrial function. Behav Brain Res 316: 115-124. https://doi.org/10.1016/j.bbr.2016.08.040 ![]() |
[35] |
Labunets IF, Utko NA, Toporova OK (2021) Effects of multipotent mesenchymal stromal cells of the human umbilical cord and their combination with melatonin in adult and aging mice with a toxic cuprizone model of demyelination. Adv Gerontol 11: 173-180. https://doi.org/10.1134/S2079057021020077 ![]() |
[36] |
Vakilzadeh G, Khodagholi F, Ghadiri T, et al. (2016) The effect of melatonin on behavioral, molecular, and histopathological changes in cuprizone model of demyelination. Mol Neurobiol 53: 4675-4684. https://doi.org/10.1007/s12035-015-9404-y ![]() |
[37] |
Pivovarova-Ramich O, Zimmermann HG, Paul F (2023) Multiple sclerosis and circadian rhythms: can diet act as a treatment?. Acta Physiol 237: e13939. https://doi.org/10.1111/apha.13939 ![]() |
[38] |
Ohl K, Tenbrock K, Kipp M (2016) Oxidative stress in multiple sclerosis: central and peripheral mode of action. Exp Neurol 277: 58-67. https://doi.org/10.1016/j.expneurol.2015.11.010 ![]() |
[39] |
Pereira GM, Soares NM, Souza AR, et al. (2018) Basal cortisol levels and the relationship with clinical symptoms in multiple sclerosis: a systematic review. Arq Neuropsiquiatr 76: 622-634. https://doi.org/10.1590/0004-282X20180091 ![]() |
[40] |
Cohen JA (2013) Mesenchymal stem cell transplantation in multiple sclerosis. J Neurol Sci 333: 43-49. https://doi.org/10.1016/j.jns.2012.12.009 ![]() |
[41] |
Hu C, Li L (2019) Melatonin plays critical role in mesenchymal stem cell-based regenerative medicine in vitro and in vivo. Stem Cell Res Ther 10: 13. https://doi.org/10.1186/s13287-018-1114-8 ![]() |
[42] |
Farhadi N, Oryan S, Nabiuni M (2014) Serum levels of melatonin and cytokines in multiple sclerosis. Biomed J 37: 90-92. https://doi.org/10.4103/2319-4170.125885 ![]() |
1. | Behnam Golabi, Hadis Razmaray, Sepideh Seyedi-Sahebari, Heliya Bandehagh, Zahra Hakimzadeh, Ailin Khosroshahi, Seyedehyasmin Moghaddamziabari, Negar Aghaei, Sarvin Sanaie, Mahnaz Talebi, Amirreza Naseri, Sleep and cognitive outcomes in multiple sclerosis; a systematic review, 2024, 24, 1471-244X, 10.1186/s12888-024-06103-5 | |
2. | Sonda Jallouli, Sameh Ghroubi, Mariem Damak, Salma Sakka, Mohamed Habib Elleuch, Chokri Mhiri, Abdelmoneem Yahia, Tarak Driss, Giovanni de Marco, Omar Hammouda, 12-week melatonin supplementation improved dynamic postural stability and walking performance in persons living with multiple sclerosis: A randomized controlled trial, 2025, 476, 01664328, 115191, 10.1016/j.bbr.2024.115191 |
Study ID | Study type | Country | Subjects | Sex and sample size | Interventions | Treatment duration | Results |
Alghamdi et al. 2020 | Observational | Saudi Arabia | Mice | All male = 30 | Cuprizone (400 mg/kg) and melatonin (80 mg/kg) daily | 5 weeks | Enhanced the memory defects, increased the nociception latency, antagonists for toxic effects of cuprizone on motor activity, enhanced memory outcomes |
Dokoohaki et al. 2017 | Observational | Iran | Lewis rats | All female = 18 | Methylprednisolone 30 mg/kg | 6 days | Decrease in melatonin level (p < 0.05), diminished IFN-γ (p < 0.01), increased serum IL-4 levels (p < 0.01) |
Ghareghani et al. 2022 | Case control | Iran | C57BL/6 mice | All female = 32 | Melatonin (10 mg/kg/day) DADA (50 mg/kg/day, orally) | 10 days | Improved neurological disability scores for melatonin, combination of melatonin + DADA improved neurological disability scores more than melatonin alone, significant increase of IL-4 and IL-10 levels, and reduction in IL-1B and TNF levels for combination therapy (p < 0.000001) |
Ghareghani et al. 2023 | Case control | Canada | C57BL/6 J wild-type mice | All males | 30 mg/kg Luzindole, 80 mg/kg melatonin | 5 weeks | Reduction in total Olig2 + oligodendroglia (p = 0.051), neural stem/progenitor cells proliferation inhibition, no influence on Olig2 + /SOX2 + cells, decreased number in melatonin did not cause modified percentage of Ly6Clow monocytes |
Gharib et al. 2022 | Case control | Egypt | Mice | All males = 50 | Cuprizone 0.2% MSC-MVs 0.2 mg/kg, A single melatonin 10 mg/kg for three weeks | 3 weeks | Increase in the duration of maintained balance, melatonin did not change the cognitive performance but MSC-MVs did. |
González et al. 2021 | Case control | Mexico | Sprague-Dawley rats | All male = 128 | IFN-® 1b (dosed at 8000 U), every third day, intramuscularly Glatiramer acetate 0.50 mg/kg/day, subcutaneously Melatonin at 10 mg/kg/day, i.p |
12 days | The least level of TNF was shown in melatonin + EAE group that was 0 pg/mL |
Ghareghani et al. 2018 | Case control | Iran | C57BL/6 mice | All female = 24 | 10 mg/kg/day i.p | 13 days | Decrease in mean clinical scores (p < 0.05), procalcitonin reduced augmented concentrations of 25-hydroxyvitamin D (p < 0.05) |
Jand et al. 2022 | Observational | Iran | KP. C57BL/6 mice | All female = 68 | Melatonin 0.1, 1.0, 5.0, 10 mg/kg | 9 days | Melatonin significantly decreased the EAE clinical scores, kynurenine pathway regulatory enzyme mRNA expression reduction inhibits the enzyme Nicotinamide N-Methyltransferase overexpression (p < 0.001) |
Labunets et al. 2023 | Case control | Ukraine | Mice | Young = 79 Aged = 79 All female |
Melatonin at 6 p.m. daily, 1 mg/kg, i.p | Days of 7, 21, and 60 | Astrocyte activation in the young and aging mice reduced the percentage of neurons with destructive variations, behavioral reactions in young and aging mice improved level of thymulin in blood, diminished only in younger mice (p < 0.05) |
Labunets et al. 2021 | Experimental | Ukraine | Mice | NA | Melatonin 1 mg/kg, at 18:00, daily, Cuprizone diet 0.5 × 106 cells, once intravenously | 11 days | Cuprizone elevated the malondialdehyde levels and reduced the activity of antioxidant enzymes in the brains of mice; there was a decrease observed in locomotor, emotional, and orientational-exploratory activity, and muscle tone |
Álvarez-Sánchez et al. 2015 | Experimental | Spain | C57BL/6 mice | All female | 80 mg/kg melatonin daily | NA | Reduced the severity of the disease (p < 0.001), diminished the incidence of EAE (p < 0.05), reduced frequencies of absolute CD4+ T macrophages (p < 0.05) |
Chen et al. 2016 | Experimental | Taiwan | C57BL/6 mic | All female | 200 mg/kg injection | Days 9, 11, and 13 after EAE induction | Improvement in clinical scores (p < 0.01), leukocyte infiltration reduction, inhibiting the T-cell proliferation, and reduced IL-10 and IL-17 expressions |
Long et al. 2018 | Experimental | China | C57BL/6 mice | All female | Melatonin 10 mg/kg, i.p | Day 0 post-immunization of EAE | Decrease in neurological deficit scores at the peak stage of EAE (p < 0.05); decrease in ROS concentrations (p < 0.05) |
Sharif et al. 2017 | Experimental | Iran | Wistar rats | All males | Melatonin (50 and 100 mg/kg/side), H-89 (10 micro-M) intra-hippocampally 30 min before each day of training | 4 days | Improved escape latency compared with H-89 alone (p = 0.008); low-dose melatonin pretreatment significantly decreased H-89-induced cytochrome c release compared to control group (p < 0.05), and high-dose melatonin completely impeded such event by leaving no significant difference compared with control group |
Ghareghani et al. 2018 | Experimental | Iran | C57BL/6 mice | All females = 40 | 10 mg/kg/day baclofen 10 mg/kg/day melatonin |
10 days | Reestablishing original normal levels of IL-4 and IFN-γ in combination therapy (p < 0.001), increase in antioxidant enzymes catalase activity in combination (p < 0.001) |
Abo Taleb et al. 2019 | Case control | Saudi Arabia | Mice | Females = 39 Males = 39 |
Cuprizone 400 mg/kg/day oral+ melatonin 80 mg/kg/day i.p at 12 p.m. | 9 weeks | Reduced MDA in males, increased CAT, SOD, GPx, and GSH, improvement in TNF and IL-1B levels |
Escribano et al. 2022 | Observational | Spain | Rats | All males = 25 | Melatonin 1 mg/kg oral 5 days a week | 51 days | Reduction in oxidative stress biomarkers levels (p < 0.001), reduction in EAE severity (p < 0.001) |
Ghareghani et al. 2016 | Observational | Iran | Rats | All females = 15 | Melatonin 10 mg/kg | 18 days | Increase in serum lactate (p < 0.01) and number of infiltrated cells (p < 0.01) |
Gabriel Ortíz et al. 2022 | Experimental | Mexico | Rats | All males = 48 | Melatonin 20 mg/kg of body weight/day, GA 10 mg/kg of body weight/day, IFN-b1b 9000 IU/kg of body weight every other day | 20 days | Reduced cumulative index score, reduced levels of IL1β, IL-6, and TNF-a, reduced MDA+4-OHA (p < 0.05) |
Vakilzadeh et al. 2015 | Cohort | Iran | Mice | N = 20 | Cuprizone, melatonin 50 mg/kg, melatonin 100 mg/kg | 7 days | Increase in moved distances for melatonin 50 mg/kg (p < 0.01) and 100 mg/kg (p < 0.001) |
Wen et al. 2016 | Cohort | United States | Mice | All females | 28 days | Melatonin 20 mg/kg i.p | Attenuated clinical severity (p < 0.05), less CD4+ positive cells (p < 0.001) |
Note: CAT: Catalase; SOD: Superoxide dismutase; GPx: Glutathione peroxidase; GSH: Glutathione; MDA: Malondialdehyde; EAE: Experimental autoimmune encephalomyelitis; GA: Glatiramer acetate; MDA: Malondialdehyde; 4-OHA: 4-hydroxyalkenals; i.p: Intraperitoneally; DADA: Diisopropyl amine dichloroacetate; MSC-MVs: Mesenchymal stem cells microvesicles; TNF: Tumor necrosis factor; CSF: Cerebrospinal fluid; ROS: Reactive oxygen species; IU: International unit.
Study ID | Approach | Simulation evidence | Duration |
Abo Taleb et al. 2019 | 400 mg/kg/day cuprizone (oral gavage with 1% CMC) | Progressive decline in locomotor activity | 5 weeks |
Vakilzadeh et al. 2016 | Diet containing 0.2 % cuprizone mixed into ground standard rodent chow | NA | 5 weeks |
Wen et al. 2016 | Injected subcutaneously with 200 µg of MOG 35–55 peptide emulsified in complete Freund's adjuvant containing 500 µg Mycobacterium tuberculosis | When an animal showed a clinical score ≥ 0.5 | 7 weeks |
Escribano et al. 2022 | 100 µL of a solution containing 150 µg of MOG | NA | 14 days |
Ghareghani et al. 2017 | Subcutaneously over the flank with 200 µL of a 1:1 mixture of 1 g GPSC in 1 mL PBS and complete Freund's adjuvant (CFA, Sigma-Aldrich, USA) and 1 mg/mL Mycobacterium tuberculosis bacteria enriched | NA | NA |
Ortíz et al. 2022 | Sterile physiological saline solution and emulsified with 50 µL of complete Freund's adjuvant containing 1 mg/mL heat-killed Mycobacterium tuberculosis strain H37Ra | NA | NA |
Ghareghani et al. 2022 | Immunization solution containing the immunogenic epitope MOG-35-55 was emulsified with complete Freund's adjuvant (CFA, Sigma-Aldrich) and Mycobacterium tuberculosis, and was injected subcutaneously over the flank | NA | NA |
Ghareghani et al. 2023 | Cuprizone (0.2% wt/wt) was incorporated into standard irradiated ground rodent chow | NA | NA |
Gharib et al. 2022 | Ad libitum and fed 0.2% (w/w) cuprizone (Sigma) in ground breeder chow | Behavioral stress | 5 weeks |
Ramos González et al. 2021 | 150 µL of a homogenate containing 25 µL of pig spinal cord homogenate, 25 µL of pig brain homogenate, and 100 µL of Freund's complete adjuvant | EAE, achieving at least grade 2 of the disease | NA |
Ghareghani et al. 2018 | Emulsified in complete Freund's adjuvant (CFA, Sigma-Aldrich), enriched Mycobacterium tuberculosis bacteria | NA | NA |
Jand et al. 2022 | Injection of MOG35-55, 300 ng of pertussis toxin (List Biological Labs, Campbell, CA, USA) intraperitoneally, into all animals, on days 0 and 2 | NA | NA |
Labunets et al. 2023 | Cuprizone at 0.2% (w/w) | NA | 3 weeks |
Álvarez-Sánchez et al. 2015 | Immunized subcutaneously in each hind leg with 100 µg of MOG35–55 (Cambridge Research Biochemicals), emulsified in CFA (Sigma) containing 50 µg of heat-killed Mycobacterium tuberculosis | NA | NA |
Chen et al. 2016 | MOG was purified to greater than 95% purity using high-performance liquid chromatography. Each C57BL/6 mouse was immunized with 100 µg of MOG in 100 µL of an emulsion of complete Freund's adjuvant and 400 µg of Mycobacterium tuberculosis H37Ra | NA | NA |
Long et al. 2018 | Injected subcutaneously at one side of the flank with 250 µg MOG35–55 peptide in complete Freund's adjuvant and 64 mg/mL of heat-killed Mycobacterium tuberculosis. At 0 h and 48 h after MOG injection, 500 ng pertussis toxin in 100 µL PBS was injected intraperitoneally | Neurological deficit | NA |
Ghareghani et al. 2018 | Immunized with MOG | Clinical signs of the disease | NA |
Dokoohaki et al. 2017 | Injected subcutaneously over the flank with 200 µL of a 1:1 mixture of 1 g of GPSC in 1 mL PBS and complete Freund's adjuvant and 1 mg/mL enriched Mycobacterium tuberculosis bacteria | Clinical signs | NA |
Alghamdi et al. 2020 | 0.2 mL cuprizone orally | NA | 5 weeks |
Note: MOG: Myelin oligodendrocyte glycoprotein; GPSC: Guinea pig spinal cord; PBS: Polybutylene succinate; EAE: Experimental autoimmune encephalomyelitis.
Study ID | Study type | Country | Subjects | Sex and sample size | Interventions | Treatment duration | Results |
Alghamdi et al. 2020 | Observational | Saudi Arabia | Mice | All male = 30 | Cuprizone (400 mg/kg) and melatonin (80 mg/kg) daily | 5 weeks | Enhanced the memory defects, increased the nociception latency, antagonists for toxic effects of cuprizone on motor activity, enhanced memory outcomes |
Dokoohaki et al. 2017 | Observational | Iran | Lewis rats | All female = 18 | Methylprednisolone 30 mg/kg | 6 days | Decrease in melatonin level (p < 0.05), diminished IFN-γ (p < 0.01), increased serum IL-4 levels (p < 0.01) |
Ghareghani et al. 2022 | Case control | Iran | C57BL/6 mice | All female = 32 | Melatonin (10 mg/kg/day) DADA (50 mg/kg/day, orally) | 10 days | Improved neurological disability scores for melatonin, combination of melatonin + DADA improved neurological disability scores more than melatonin alone, significant increase of IL-4 and IL-10 levels, and reduction in IL-1B and TNF levels for combination therapy (p < 0.000001) |
Ghareghani et al. 2023 | Case control | Canada | C57BL/6 J wild-type mice | All males | 30 mg/kg Luzindole, 80 mg/kg melatonin | 5 weeks | Reduction in total Olig2 + oligodendroglia (p = 0.051), neural stem/progenitor cells proliferation inhibition, no influence on Olig2 + /SOX2 + cells, decreased number in melatonin did not cause modified percentage of Ly6Clow monocytes |
Gharib et al. 2022 | Case control | Egypt | Mice | All males = 50 | Cuprizone 0.2% MSC-MVs 0.2 mg/kg, A single melatonin 10 mg/kg for three weeks | 3 weeks | Increase in the duration of maintained balance, melatonin did not change the cognitive performance but MSC-MVs did. |
González et al. 2021 | Case control | Mexico | Sprague-Dawley rats | All male = 128 | IFN-® 1b (dosed at 8000 U), every third day, intramuscularly Glatiramer acetate 0.50 mg/kg/day, subcutaneously Melatonin at 10 mg/kg/day, i.p |
12 days | The least level of TNF was shown in melatonin + EAE group that was 0 pg/mL |
Ghareghani et al. 2018 | Case control | Iran | C57BL/6 mice | All female = 24 | 10 mg/kg/day i.p | 13 days | Decrease in mean clinical scores (p < 0.05), procalcitonin reduced augmented concentrations of 25-hydroxyvitamin D (p < 0.05) |
Jand et al. 2022 | Observational | Iran | KP. C57BL/6 mice | All female = 68 | Melatonin 0.1, 1.0, 5.0, 10 mg/kg | 9 days | Melatonin significantly decreased the EAE clinical scores, kynurenine pathway regulatory enzyme mRNA expression reduction inhibits the enzyme Nicotinamide N-Methyltransferase overexpression (p < 0.001) |
Labunets et al. 2023 | Case control | Ukraine | Mice | Young = 79 Aged = 79 All female |
Melatonin at 6 p.m. daily, 1 mg/kg, i.p | Days of 7, 21, and 60 | Astrocyte activation in the young and aging mice reduced the percentage of neurons with destructive variations, behavioral reactions in young and aging mice improved level of thymulin in blood, diminished only in younger mice (p < 0.05) |
Labunets et al. 2021 | Experimental | Ukraine | Mice | NA | Melatonin 1 mg/kg, at 18:00, daily, Cuprizone diet 0.5 × 106 cells, once intravenously | 11 days | Cuprizone elevated the malondialdehyde levels and reduced the activity of antioxidant enzymes in the brains of mice; there was a decrease observed in locomotor, emotional, and orientational-exploratory activity, and muscle tone |
Álvarez-Sánchez et al. 2015 | Experimental | Spain | C57BL/6 mice | All female | 80 mg/kg melatonin daily | NA | Reduced the severity of the disease (p < 0.001), diminished the incidence of EAE (p < 0.05), reduced frequencies of absolute CD4+ T macrophages (p < 0.05) |
Chen et al. 2016 | Experimental | Taiwan | C57BL/6 mic | All female | 200 mg/kg injection | Days 9, 11, and 13 after EAE induction | Improvement in clinical scores (p < 0.01), leukocyte infiltration reduction, inhibiting the T-cell proliferation, and reduced IL-10 and IL-17 expressions |
Long et al. 2018 | Experimental | China | C57BL/6 mice | All female | Melatonin 10 mg/kg, i.p | Day 0 post-immunization of EAE | Decrease in neurological deficit scores at the peak stage of EAE (p < 0.05); decrease in ROS concentrations (p < 0.05) |
Sharif et al. 2017 | Experimental | Iran | Wistar rats | All males | Melatonin (50 and 100 mg/kg/side), H-89 (10 micro-M) intra-hippocampally 30 min before each day of training | 4 days | Improved escape latency compared with H-89 alone (p = 0.008); low-dose melatonin pretreatment significantly decreased H-89-induced cytochrome c release compared to control group (p < 0.05), and high-dose melatonin completely impeded such event by leaving no significant difference compared with control group |
Ghareghani et al. 2018 | Experimental | Iran | C57BL/6 mice | All females = 40 | 10 mg/kg/day baclofen 10 mg/kg/day melatonin |
10 days | Reestablishing original normal levels of IL-4 and IFN-γ in combination therapy (p < 0.001), increase in antioxidant enzymes catalase activity in combination (p < 0.001) |
Abo Taleb et al. 2019 | Case control | Saudi Arabia | Mice | Females = 39 Males = 39 |
Cuprizone 400 mg/kg/day oral+ melatonin 80 mg/kg/day i.p at 12 p.m. | 9 weeks | Reduced MDA in males, increased CAT, SOD, GPx, and GSH, improvement in TNF and IL-1B levels |
Escribano et al. 2022 | Observational | Spain | Rats | All males = 25 | Melatonin 1 mg/kg oral 5 days a week | 51 days | Reduction in oxidative stress biomarkers levels (p < 0.001), reduction in EAE severity (p < 0.001) |
Ghareghani et al. 2016 | Observational | Iran | Rats | All females = 15 | Melatonin 10 mg/kg | 18 days | Increase in serum lactate (p < 0.01) and number of infiltrated cells (p < 0.01) |
Gabriel Ortíz et al. 2022 | Experimental | Mexico | Rats | All males = 48 | Melatonin 20 mg/kg of body weight/day, GA 10 mg/kg of body weight/day, IFN-b1b 9000 IU/kg of body weight every other day | 20 days | Reduced cumulative index score, reduced levels of IL1β, IL-6, and TNF-a, reduced MDA+4-OHA (p < 0.05) |
Vakilzadeh et al. 2015 | Cohort | Iran | Mice | N = 20 | Cuprizone, melatonin 50 mg/kg, melatonin 100 mg/kg | 7 days | Increase in moved distances for melatonin 50 mg/kg (p < 0.01) and 100 mg/kg (p < 0.001) |
Wen et al. 2016 | Cohort | United States | Mice | All females | 28 days | Melatonin 20 mg/kg i.p | Attenuated clinical severity (p < 0.05), less CD4+ positive cells (p < 0.001) |
Study ID | Approach | Simulation evidence | Duration |
Abo Taleb et al. 2019 | 400 mg/kg/day cuprizone (oral gavage with 1% CMC) | Progressive decline in locomotor activity | 5 weeks |
Vakilzadeh et al. 2016 | Diet containing 0.2 % cuprizone mixed into ground standard rodent chow | NA | 5 weeks |
Wen et al. 2016 | Injected subcutaneously with 200 µg of MOG 35–55 peptide emulsified in complete Freund's adjuvant containing 500 µg Mycobacterium tuberculosis | When an animal showed a clinical score ≥ 0.5 | 7 weeks |
Escribano et al. 2022 | 100 µL of a solution containing 150 µg of MOG | NA | 14 days |
Ghareghani et al. 2017 | Subcutaneously over the flank with 200 µL of a 1:1 mixture of 1 g GPSC in 1 mL PBS and complete Freund's adjuvant (CFA, Sigma-Aldrich, USA) and 1 mg/mL Mycobacterium tuberculosis bacteria enriched | NA | NA |
Ortíz et al. 2022 | Sterile physiological saline solution and emulsified with 50 µL of complete Freund's adjuvant containing 1 mg/mL heat-killed Mycobacterium tuberculosis strain H37Ra | NA | NA |
Ghareghani et al. 2022 | Immunization solution containing the immunogenic epitope MOG-35-55 was emulsified with complete Freund's adjuvant (CFA, Sigma-Aldrich) and Mycobacterium tuberculosis, and was injected subcutaneously over the flank | NA | NA |
Ghareghani et al. 2023 | Cuprizone (0.2% wt/wt) was incorporated into standard irradiated ground rodent chow | NA | NA |
Gharib et al. 2022 | Ad libitum and fed 0.2% (w/w) cuprizone (Sigma) in ground breeder chow | Behavioral stress | 5 weeks |
Ramos González et al. 2021 | 150 µL of a homogenate containing 25 µL of pig spinal cord homogenate, 25 µL of pig brain homogenate, and 100 µL of Freund's complete adjuvant | EAE, achieving at least grade 2 of the disease | NA |
Ghareghani et al. 2018 | Emulsified in complete Freund's adjuvant (CFA, Sigma-Aldrich), enriched Mycobacterium tuberculosis bacteria | NA | NA |
Jand et al. 2022 | Injection of MOG35-55, 300 ng of pertussis toxin (List Biological Labs, Campbell, CA, USA) intraperitoneally, into all animals, on days 0 and 2 | NA | NA |
Labunets et al. 2023 | Cuprizone at 0.2% (w/w) | NA | 3 weeks |
Álvarez-Sánchez et al. 2015 | Immunized subcutaneously in each hind leg with 100 µg of MOG35–55 (Cambridge Research Biochemicals), emulsified in CFA (Sigma) containing 50 µg of heat-killed Mycobacterium tuberculosis | NA | NA |
Chen et al. 2016 | MOG was purified to greater than 95% purity using high-performance liquid chromatography. Each C57BL/6 mouse was immunized with 100 µg of MOG in 100 µL of an emulsion of complete Freund's adjuvant and 400 µg of Mycobacterium tuberculosis H37Ra | NA | NA |
Long et al. 2018 | Injected subcutaneously at one side of the flank with 250 µg MOG35–55 peptide in complete Freund's adjuvant and 64 mg/mL of heat-killed Mycobacterium tuberculosis. At 0 h and 48 h after MOG injection, 500 ng pertussis toxin in 100 µL PBS was injected intraperitoneally | Neurological deficit | NA |
Ghareghani et al. 2018 | Immunized with MOG | Clinical signs of the disease | NA |
Dokoohaki et al. 2017 | Injected subcutaneously over the flank with 200 µL of a 1:1 mixture of 1 g of GPSC in 1 mL PBS and complete Freund's adjuvant and 1 mg/mL enriched Mycobacterium tuberculosis bacteria | Clinical signs | NA |
Alghamdi et al. 2020 | 0.2 mL cuprizone orally | NA | 5 weeks |