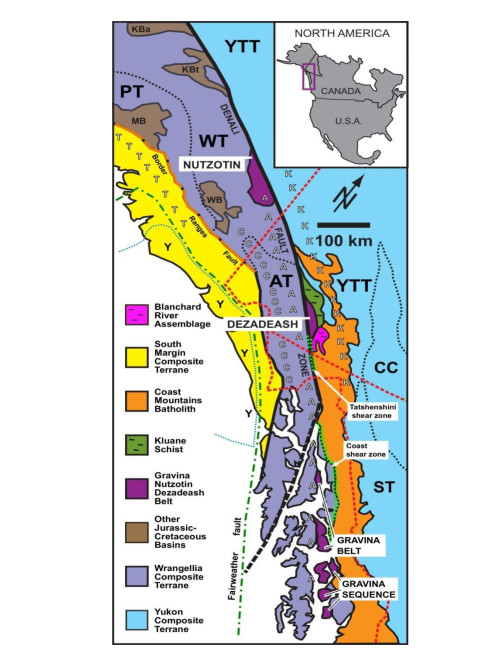
Differential evolution (DE) is one of the most successful evolutionary algorithms. However, the performance of DE is significantly influenced by its mutation strategies. Generally, different mutation strategies may obtain different search directions. The improper search direction misleads the search and results in the poor performance of DE. Therefore, it is vital to consider the search direction when designing new mutation strategies. Based on this consideration, in this paper, the quasi-reflection-based mutation is proposed to enhance the performance of DE. The quasi-reflection-based mutation is able to provide the promising search direction to guide the search. To extensively evaluate the performance of our approach, benchmark functions are chosen as the test suite. Combined with SHADE, Re-SHADE is presented. Compared with different advanced DE methods, Re-SHADE can obtain better results in terms of the accuracy and the convergence rate. Additionally, further experiments on the CEC2013 test suite also confirm the effectiveness of the proposed method.
Citation: Wei Li, Wenyin Gong. Differential evolution with quasi-reflection-based mutation[J]. Mathematical Biosciences and Engineering, 2021, 18(3): 2425-2441. doi: 10.3934/mbe.2021123
[1] | Vadim Khomich, Svyatoslav Shcheka, Natalia Boriskina . Geodynamic factors in the formation of large gold-bearing provinces with Carlin-type deposits on continental margins in the North Pacific. AIMS Geosciences, 2023, 9(4): 672-696. doi: 10.3934/geosci.2023036 |
[2] | Elmar Buchner, Volker J Sach, Martin Schmieder . Ries impact deposits in the North Alpine Foreland Basin of Germany as a terrestrial analog site for impact-produced seismites and sand spikes on planet Mars. AIMS Geosciences, 2025, 11(1): 68-90. doi: 10.3934/geosci.2025005 |
[3] | Ramón Delanoy, Misael Díaz-Asencio, Rafael Méndez-Tejeda . Sedimentation in the Bay of Samaná, Dominican Republic (1900–2016). AIMS Geosciences, 2020, 6(3): 298-315. doi: 10.3934/geosci.2020018 |
[4] | Nataša Katić, Joakim S. Korshøj, Helle F. Christensen . Bryozoan limestone experience—the case of Stevns Klint. AIMS Geosciences, 2019, 5(2): 163-183. doi: 10.3934/geosci.2019.2.163 |
[5] | Margherita Bufalini, Farabollini Piero, Fuffa Emy, Materazzi Marco, Pambianchi Gilberto, Tromboni Michele . The significance of recent and short pluviometric time series for the assessment of flood hazard in the context of climate change: examples from some sample basins of the Adriatic Central Italy. AIMS Geosciences, 2019, 5(3): 568-590. doi: 10.3934/geosci.2019.3.568 |
[6] | Richard W. Stoffle, Kathleen A. Van Vlack, Heather H. Lim, Alannah Bell, Landon Yarrington . Breaking the Clovis glass ceiling: Native American oral history of the Pleistocene. AIMS Geosciences, 2024, 10(3): 436-459. doi: 10.3934/geosci.2024023 |
[7] | Wu Yang, Ning Yu, Mingxing Yang, Jun Yan, Min Zhang, ShiQiang Yang . Sustainable development of geological resources: the Characteristics of Red Karst Landscape and Tourism Development in Tongren, Guizhou. AIMS Geosciences, 2024, 10(1): 141-171. doi: 10.3934/geosci.2024009 |
[8] | Evgeny P Terekhov, Anatoly V Mozherovsky . Some features of geological structure of the Shikotan Island (Lesser Kuril Arc)—A view from "Space". AIMS Geosciences, 2024, 10(4): 907-917. doi: 10.3934/geosci.2024042 |
[9] | Binoy Kumar Barman, K. Srinivasa Rao, Kangkana Sonowal, Zohmingliani, N.S.R. Prasad, Uttam Kumar Sahoo . Soil erosion assessment using revised universal soil loss equation model and geo-spatial technology: A case study of upper Tuirial river basin, Mizoram, India. AIMS Geosciences, 2020, 6(4): 525-544. doi: 10.3934/geosci.2020030 |
[10] | Armin Salsani, Abdolhossein Amini, Shahram shariati, Seyed Ali Aghanabati, Mohsen Aleali . Geochemistry, facies characteristics and palaeoenvironmental conditions of the storm-dominated phosphate-bearing deposits of eastern Tethyan Ocean; A case study from Zagros region, SW Iran. AIMS Geosciences, 2020, 6(3): 316-354. doi: 10.3934/geosci.2020019 |
Differential evolution (DE) is one of the most successful evolutionary algorithms. However, the performance of DE is significantly influenced by its mutation strategies. Generally, different mutation strategies may obtain different search directions. The improper search direction misleads the search and results in the poor performance of DE. Therefore, it is vital to consider the search direction when designing new mutation strategies. Based on this consideration, in this paper, the quasi-reflection-based mutation is proposed to enhance the performance of DE. The quasi-reflection-based mutation is able to provide the promising search direction to guide the search. To extensively evaluate the performance of our approach, benchmark functions are chosen as the test suite. Combined with SHADE, Re-SHADE is presented. Compared with different advanced DE methods, Re-SHADE can obtain better results in terms of the accuracy and the convergence rate. Additionally, further experiments on the CEC2013 test suite also confirm the effectiveness of the proposed method.
The Mesozoic convergence and subsequent collision of the Wrangellia composite terrane (WCT) against the western margin of North America, represented by the Yukon composite terrane (YCT), was accompanied by the collapse of several intervening flysch basins. The flysch basins are preserved as relatively thick, variably deformed Jurassic-Cretaceous sedimentary and volcanic rocks scattered along the Northern Cordillera of Alaska and Yukon (Figure 1). One of the best studied flysch basins is the Gravina-Nutzotin belt [4], comprising, from south to north, the Gravina sequence and Gravina belt in southeastern Alaska (herein referred to as the “southern” part of the Gravina-Nutzotin belt), and the Dezadeash Formation in Yukon and the Nutzotin Mountains sequence in east-central Alaska (herein referred to as the "northern" part of the Gravina-Nutzotin belt) (Figure 1).
Previous work on the southern part of the Gravina-Nutzotin belt (i.e., the Gravina sequence and Gravina belt in southeastern Alaska) demonstrated that these rocks were tectonically emplaced beneath the YCT along a regionally extensive (1200 km long) west-vergent thrust system in the mid-Cretaceous [5,6,7,8]. A regional magnetotelluric survey in east-central Alaska identified a very-low resistivity layer beneath YCT rocks extending from about 10 km to 20 km in depth, which was interpreted as tectonically underthrust Mesozoic flysch [9]. This interpretation was apparently supported by a refraction/wide-angle reflection seismic survey that suggested the presence of reflective and near-horizontal layering beneath YCT rocks from approximately 8 to 27 km depth [10]. Subsequently, the mid-Cretaceous thrust system was implicated in the metamorphism and deformation of the entire Gravina-Nutzotin belt [11].
However, additional studies cast doubt on the existence of underthrust Mesozoic flysch [12,13,14]. The very-low resistivity layer identified previously was likely highly conductive YCT rocks and not underthrust flysch [12]; the very-low resistivity layer was suggested to be the result of lower-crustal fluids in YCT rocks [13]; and isotopic evidence was presented discounting the magnetotelluric, Mesozoic underthrust flysch interpretation [14]. Nevertheless, researchers continue to invoke the underthrust flysch scenario for interpreting the structural deformation and crustal-scale structure of the Northern Cordillera [15,16,17,18,19], despite any detailed studies of the tectonometamorphic history of the northern part of the Gravina-Nutzotin belt.
The purpose of this paper is to characterize the metamorphic character of the Dezadeash Formation, including the metamorphic grade, maximum temperature and pressure reached by the strata, timing of metamorphism, and type of metamorphism. The study presents the results of a multidisciplinary analysis of the Dezadeash Formation, comprising: structural fabrics observed in outcrop; petrographic examination of thin sections of sandstones, mudstone, hemipelagite, and volcaniclastic beds; X-ray diffraction of sandstone, mudstone, hemipelagite, and volcaniclastic beds, including whole-rock, clay speciation, and illite Kübler index and chlorite Árkai index "crystallinity" determinations; microfossil analysis of mudstone and hemipelagite beds for palynomorphs and limestone clasts for conodonts; and programmed pyrolysis maturation analysis of mudstones and hemipelagites. Published thermochronometric data from the Dezadeash Formation are also incorporated. The aim of the paper is to constrain the tectonometamorphic evolution of the Dezadeash Formation, which has implications regarding the convergence and subsequent accretion of the WCT to the Mesozoic margin of North American, particularly with regards to the mid-Cretaceous west-vergent thrust system in the Northern Cordillera.
The Northern Cordillera of North America is an amalgamation of allochthonous composite terranes, superimposed magmatic arcs, and exhumed sedimentary flysch basins, all variably offset by lithospheric-scale strike-slip faults. Geologic elements relevant to this study include (Figure 1): the Yukon and Wrangellia composite terranes; the Chitina, Chisana, and Kluane arcs; the Gravina-Nutzotin belt flysch basins (i.e., the Dezadeash Formation, Nutzotin Mountains sequence, Gravina sequence, and Gravina belt); the Blanchard River assemblage and Kluane Schist high-grade metamorphic belts; and the Denali fault and Coast-Tatshenshini shear zone.
The Yukon composite terrane (YCT) consists of the polymetamorphosed and polydeformed, pericratonic and arc-related Yukon-Tanana, Slide Mountain, Cache Creek, Quesnellia, and Stikinia terranes (Figure 1) [1,2,3]. The YTC was accreted to North America during the mid-Jurassic [2,20], thus forming the Mesozoic margin of North America.
The Wrangellia composite terrane (WCT) is an amalgamation of three tectonostratigraphic terranes referred to as the Alexander, Wrangellia, and Peninsular terranes (Figure 1) [21]. These represent Neoproterozoic to Mesozoic island arc-related volcanic and sedimentary rocks [23,24]. The WCT is interpreted as part of an obliquely converging oceanic plateau [25] that was emplaced against the Mesozoic margin of North America along a regionally extensive, mid-Cretaceous, west-vergent thrust system [7,11,22,26]. Although there is general agreement that convergence and accretion were diachronous—including closure of the intervening flysch basins—there are conflicting interpretations whether accretion propagated zipper-like south-to-north or north-to-south [16,27,28,29,30,31,32,33].
The Late Jurassic-Early Cretaceous Chitina arc (~160–140 Ma) ("C" in Figure 1) is restricted to the Wrangell and Alexander terranes along the outboard margin of the WCT [22,34,35,36]. Extensive volcanic and volcaniclastic rocks derived from the Chitina arc are scattered throughout the Gravina-Nutzotin belt: generally volcanic and volcaniclastic rocks occur in the southern part of the belt (i.e., Gravina belt and Gravina sequence), and only volcaniclastic rocks occur in the northern part of the belt (i.e., Dezadeash Formation and Nutzotin Mountains sequence) [22]. Plutonic rocks interpreted as the roots of the Chitina arc extend throughout the Northern Cordillera. The plutonic rocks occur as elongate batholithic complexes consisting mainly of quartz diorite, tonalite and granodiorite that range from Late Jurassic to Early Cretaceous in age (~160–130 Ma) [34,35,37].
The Late Cretaceous Chisana arc (~120–105 Ma) ("A" in Figure 1) is restricted to the Wrangell and Alexander terranes along the inboard margin of the WCT [16,38]. Extensive volcanic and volcaniclastic rocks derived from the Chisana arc are scattered throughout the Gravina-Nutzotin belt (i.e., Gravina belt and Gravina sequence, and Nutzotin Mountains sequence). In addition, plutonic rocks interpreted as the roots of the Chisana arc also extend throughout the Northern Cordillera. The plutonic rocks occur as elongate batholithic complexes and plutons consisting of granodiorite, quartz diorite, diorite, and rarer quartz monzonite that are Early Cretaceous in age (~117–106 Ma) [34,35,37]. One of these plutonic complexes, referred to as the Shorty Creek pluton, intrudes the Dezadeash Formation in southwest Yukon (Figure 2), and has a K-Ar age of ~106 Ma [37]. Roots of the Chisana arc are also preserved as Alaskan-type ultramafic complexes: the Pyroxenite Creek ultramafic complex in southwestern Yukon (Figure 2) intrudes the Dezadeash Formation and has K-Ar ages of ~128–109 Ma and a Rb-Sr age of ~116 Ma (Rb-Sr) [39].
The Late Cretaceous-Paleogene Kluane arc (~85–45 Ma) ("K" in Figure 1) occurs much farther inboard than either the Chitina and Chisana arcs [40,41]. Plutonic rocks interpreted as the roots of the Kluane arc form part of the extensive Coast Plutonic Complex or Coast Mountains Batholith (~175–45 Ma) that extends for much of the length of the Northern Cordillera (Figure 1) [42,43]. The plutonic rocks occur as batholithic complexes consisting mainly of diorite, quartz diorite, granodiorite and locally monzonite, and syenite [34]. The Coast Plutonic Complex intrudes both the WCT and the YCT is inferred to have formed immediately after accretion of the WCT with the YCT (i.e., the ancient margin of western North America), thereby obscuring the "Shakwak" suture between these two composite terranes [41,44].
The Dezadeash Formation is an approximately 3000 m thick succession of thin- to thick-bedded turbidites and massive sandstone with minor amounts of conglomeratic mudstone containing limestone clasts up to ~10 m in exposed longest dimensions, volcaniclastic rocks, and hemipelagic lime mudstone [45,46,47]. Based on detailed lithofacies analysis, the Dezadeash Formation represents mainly the middle and lower subdivisions of a point-source, mud/sand-rich submarine fan that was derived from the WCT and Chitina arc [47,48].
The Dezadeash Formation is Late Jurassic (Oxfordian) to Early Cretaceous (Valanginian) in age based on collections of the bivalve Buchia [45], and uncomfortably overlies the Alexander and Wrangellia terranes [49]. The Dezadeash Formation is overlain unconformably by ~1000 m of unmetamorphosed nonmarine Paleogene clastic and volcaniclastic rocks of the Amphitheater Formation [45,50].
Two phases of folding have been identified in the Dezadeash Formation: the oldest folds (F1) trend northerly, are asymmetric or overturned to the east, and locally change laterally into thrust faults; the youngest folds (F2) trend west-northwesterly and are open [45]. The oldest folds are crosscut by the Shorty Creek pluton that reveals a K-Ar age of ~106 Ma [37] and they are attributed to movement on a―"tectonic slope"‖ because the trend of the folds is similar to the trend of penecontemporaneous slump folds in the Dezadeash Formation [45]. The youngest folds (F2) are ascribed to westward directed thrusting of the Kluane Schist over the Dezadeash Formation and movement on the Denali fault zone. Both the Kluane Schist and Denali fault are described later [45].
The Dezadeash Formation has also been utilized in low-temperature thermochronometric investigations. Specifically, two samples from the St. Elias Mountains syntaxis in southwest Yukon (Figure 2) [51] are summarized in Table 1. The thermochronometric investigations reveal multiple episodes of exhumation and landscape evolution attributed to rapid cooling at ~95–75 Ma due to accretion of the WCT to the YCT, slow cooling during ~75–30 Ma caused by relief degradation, and renewed rapid cooling beginning ~30 Ma and continuing to the present, attributed to flat-slab subduction of the Yakutat terrane and strike-slip displacement on the Denali fault zone [54].
Sample | Lithology | Elevation (m) | AHe (Ma) | ZHe (Ma) | AFT (Ma) | ZFT (Ma) |
KLB04 | metapelite | 605 | 17.8 ± 1.6 | 59.7 ± 8.1 | n.a. | n.a. |
KLB91 | mudstone | 725 | 11.4 ± 0.8 | 68.8 ± 2.3 | n.a. | 109.9 ± 8.9 |
Tc (℃) | 70 | 180 | 120 | 240 | ||
PRZ (℃) | 40–70 | 130–180 | n.a. | n.a. | ||
PAZ (℃) | n.a. | n.a. | 60–120 | 180–240 | ||
Note: Samples KLB04 and KLB91 from [51]. •AHe = apatite (U-Th)/He, ZHe = zircon (U-Th)/He, AFT = apatite fission-track, and ZFT = zircon fission-track thermochronometer age; •Tc = closure temperature (radioactive decay product retained below this temperature, but not above it; also referred to as the temperature of a mineral at the time given by its radiometric age); •PRZ = partial retention zone (temperature window over which radioactive decay product is retained); •PAZ = partial annealing zone (temperature window over which radioactive decay product is preserved). Closure temperatures from [52,53]. Note that the temperatures are only approximate because they depend on the cooling rate, chemistry of the mineral, and grain-size of the mineral [52]. |
The Nutzotin Mountains sequence is the proximal half of the Nutzotin-Dezadeash basin that was offset by the Denali fault (described later). The Nutzotin Mountains sequence is up to 3000 m thick and consists mainly of thin-bedded turbidites with minor amounts of massive sandstone, conglomeratic mudstone containing limestone clasts up to ~10 m exposed longest dimensions, as well as hemipelagite beds [4,55,56,57]. The strata are interpreted as westerly sourced, distal to proximal submarine fan deposits that grade upward into shelf deposits [56,57]. The Nutzotin Mountains sequence is Late Jurassic (Tithonian) to Early Cretaceous (Valanginian) in age and unconformably overlies the Wrangellia terrane [58]. The strata are unmetamorphosed and deformed by north-dipping thrust faults and overturned folds that are crosscut by 117–105 Ma plutons [57].
The Gravina sequence consists ~2200 m of mainly metavolcanic rocks and minor amounts of metasedimentary rocks [59]. U-Pb zircon ages (154–158 Ma) of granitic clasts and rare fossils suggest a Late Jurassic in age [59]). The sequence is interpreted as submarine lava flows and submarine fans deposited on the flanks a volcanic arc [59]. The Gravina sequence experienced greenschist to amphibolite facies metamorphism related to the mid-Cretaceous, regionally extensive west-vergent thrust system [7,8].
The Gravina belt includes a lower, ~1000 m thick unit of mafic volcanic and volcaniclastic rocks inferred to be Late Jurassic in age [60,61], and an upper, ~1800 m thick sequence of sandstone and mudstone turbidites with minor amounts of conglomerate referred to as the Seymour Canal Formation [60,62]. Fossils indicate the Seymour Canal Formation is Late Jurassic to Early Cretaceous, and strata are interpreted as upper and middle submarine fan deposits sourced from the WCT [62]. The Gravina belt was subject to zeolite to amphibolite facies metamorphism and experienced pressures of 8.7 ± 1 kbar (~25–30 km depth) and temperatures between 465 ± 50 ℃ to 545 ± 75 ℃ [60,63] related to the mid-Cretaceous, regionally extensive west-vergent thrust system [7].
The Blanchard River assemblage is juxtaposed along the southern margin of the Dezadeash Formation (Figure 1). It consists mainly of penetratively deformed, interlayered quartz-biotite schist and quartz-biotite psammitic schist that grades eastward into proto-gneiss and paragneiss before becoming engulfed by the Ruby Range batholith (~64–54 Ma) of the Coast Plutonic Complex [19,26]. The Blanchard River assemblage is ~5000–6000 m thick and has a detrital zircon based maximum depositional age of 130–125 Ma [19]. The Dezadeash Formation, Blanchard River assemblage, and YCT are interpreted as a structural stack related to the mid-Cretaceous, regionally extensive west-vergent thrust system, although thrusting was somewhat diachronous (i.e., ~90 Ma in southeast Alaska compared to ~83–76 Ma in Yukon) [19,26]. The Blanchard River assemblage reached amphibolite facies metamorphism with P-T conditions estimated to have been ~6.5 kbars (~24 km depth) and ~640 ℃ [19,26].
The Kluane Schist is juxtaposed along the eastern margin of the Dezadeash Formation (Figure 1). It is a sequence of graphitic mica-chlorite-quartz schist and gneiss with a structural thickness of ~12, 000 m that contains interfoliated bodies of serpentinized dunite up to 1.5 × 15 km in exposed dimensions [64]. Zircon geochronology indicates the protolith of the Kluane Schist is Late Cretaceous in age (<94 Ma) [17]. The Dezadeash Formation, Kluane Schist and YCT are also interpreted as a structural stack related to the mid-Cretaceous, regionally extensive west-vergent thrust system [17]. The Kluane Schist was subject to greenschist to amphibolite facies metamorphism with P-T estimated to have been 7–8 kbar (~24–30 km depth) and 500 ℃ [17,64,65].
The Denali fault system forms the western margin of the Dezadeash Formation (Figure 1). The Dezadeash Formation and Nutzotin Mountains sequence represent the same strata that was dismembered and displaced by the Denali fault system [45]. The Denali fault system is one of the main strike-slip faults in the Northern Cordillera, along which ~370 km of dextral slip occurred since the Early Cretaceous [66,67]. with the western boundary of the Coast Plutonic Complex is the Coast shear zone (Figure 1). The Coast shear zone extends more than 1000 km from southern British Columbia into southeastern Alaska [68,69]. Displacement across the Coast shear zone is dominantly east-over-west, and this occurred mainly in mid-Cretaceous time as part of the regionally extensive west-vergent thrust system [7,8,61,68,69,70]. Synkinematic tonalite sills intrude the western margin of the shear zone [61,68,69,70,71,72].
The Coast Shear zone was extended into southwest Yukon as part of the Tatshenshini shear zone (Figure 1) [73]. The Tatshenshini shear zone is characterized by phyllitic and protomylonitic to mylonitic turbidites of the Dezadeash Formation in the footwall and protomylonitic granodiorite of the Ruby Range Batholith (part of the Coast Plutonic Complex) in the hangingwall [73]. Kinematic indicators from the Dezadeash Formation and Ruby Range Batholith record a top-to-the southwest sense of shear that occurred in the Cretaceous.
This study is based on 16, 335 m of measured strata from ~75 sections throughout the Dezadeash Formation (Figure 2, and Table 2). In addition to collecting standard bed-by-bed sedimentological measurements (i.e., bed thickness, lithology, grain size, and sedimentary structures), data was also collected pertaining to secondary deformation features (i.e., cleavage, folds, joints, and veins). Approximately 200 samples were collected from these sections, and a subset of samples representative of a wide range of lithologies were selected for a variety of analyses, including standard thin sections, X-ray diffraction, microfossils (palynomorphs and conodonts), and pyrolysis. Standard thin sections were prepared by Vancouver Petrographics Ltd. (Vancouver, British Columbia, Canada), and included sandstone (35), coquina (4), mudstone (11), hemipelagite (7), and tuff (15) samples. X-ray diffraction analysis was undertaken by AGAT Laboratories Ltd. (Calgary, Alberta, Canada) on a Siemens D5000 Bragg-Brentano diffractometer, and comprised sandstone (2), hemipelagite (1), and tuff (1) samples for quantitative X-ray diffraction analysis, including clay speciation, and scanning electron microscope (SEM) with energy dispersive X-ray spectroscopy (SEM-EDX) analyses. X-ray diffraction analysis was also performed by Activation Laboratories Ltd. (Ancaster, Ontario, Canada) on a Panalytical X'Pert Pro diffractometer, and consisted of mudstone (2) samples for quantitative XRD analysis, including clay speciation, the Rietveld method (i.e., whole pattern fitting) for determining the quantities of crystalline mineral phases, and determination of the Kübler Index (KI) and the Árkai Index (AI). Both KI and AI measure the "sharpness" of the diffractogram peaks; the sharpness of the peaks is an indication of the crystallinity of the clay minerals, which provides an indication of the extent of diagenesis and metamorphism [74,75].
Section | NTS Map | Easting | Northing | Easting | Northing | ||
1 | 115 A/11 | Start | 371000 | 6719500 | End | 369200 | 6718000 |
2 | 115 A/11 | Start | 371600 | 6718700 | End | 371200 | 6718600 |
3 | 115 A/11 | Start | 370400 | 6720800 | End | 369200 | 6720500 |
4 | 115 A/11 | Start | 369700 | 6716600 | End | 369200 | 6716200 |
5, 264 | 115 A/11 | Start | 369700 | 6716500 | End | 369400 | 6716700 |
6 | 115 A/11 | Start | 370500 | 6716300 | End | 370300 | 6716600 |
7 | 115 A/11 | Start | 369500 | 6715600 | End | 369300 | 6716000 |
8 | 115 A/11 | Start | 370300 | 6717000 | End | 369400 | 6717500 |
9 | 115 A/11 | Start | 370600 | 6717800 | End | 369800 | 6718300 |
10 | 115 A/11 | Start | 370900 | 6718300 | End | 370400 | 6718200 |
12 | 115 A/11 | Start | 369300 | 6715700 | End | 368500 | 6716700 |
13 | 115 A/11 | Start | 385400 | 6702500 | End | 383600 | 6702300 |
14A | 115 A/11 | Start | 385500 | 6699300 | End | 384800 | 6699200 |
14B | 115 A/11 | Start | 384700 | 6698800 | End | 384300 | 6698700 |
16 | 115 A/11 | Start | 381200 | 6698200 | End | 380700 | 6698200 |
17 | 115 A/11 | Start | 383300 | 6696900 | End | 384200 | 6697700 |
18 | 115 A/11 | Start | 385100 | 6703900 | End | 384100 | 6703800 |
19 | 115 A/11 | Start | 377000 | 6715000 | End | 376200 | 6714300 |
20 | 115 A/11 | Start | 382500 | 6687700 | End | 382300 | 6687300 |
21B | 115 A/11 | Start | 384300 | 6695700 | End | 384300 | 6695700 |
22 | 115 A/11 | Start | 384700 | 7604600 | End | 384500 | 6705000 |
23 | 115 A/11 | Start | 384300 | 6705400 | End | 383800 | 6705900 |
24 | 115 A/11 | Start | 384700 | 6684700 | End | 384500 | 6684800 |
25 | 115 A/11 | Start | 382200 | 6709300 | End | 381400 | 6708700 |
26 | 115 A/11 | Start | 377200 | 6716300 | End | 382400 | 6715700 |
27, 224, 228 | 115 A/2 | Start | 392000 | 6665100 | End | 392000 | 6665100 |
28 | 115 A/2 | Start | 392100 | 6675100 | End | 392100 | 6676100 |
57, 231 | A/2 | Start | 398000 | 6685100 | End | 398800 | 6688100 |
201–202 | 115 A/6 | Start | 371800 | 6701400 | End | 371800 | 6701400 |
203–206 | 115 A/6 | Start | 373800 | 6711400 | End | 372800 | 6711400 |
207–208 | 115 A/6 | Start | 371200 | 6701400 | End | 371200 | 6701400 |
211–217 | 115 A/6 | Start | 372200 | 6711400 | End | 372200 | 6711400 |
219–222 | 115 A/6 | Start | 373000 | 6708700 | End | 373000 | 6708700 |
240–246 | 115 A/12 | Start | 358200 | 6717500 | End | 358200 | 6717500 |
247 | 115 A/12 | Start | 359400 | 6717700 | End | 359400 | 6717700 |
250–252, 261 | 115 A/12 | Start | 358500 | 6725000 | End | 358500 | 6725000 |
254, 260 | 115 A/12 | Start | 361600 | 6724500 | End | 360700 | 6725300 |
257 | 115 A/12 | Start | 358600 | 6724700 | End | 358600 | 6724700 |
280, 74B, 400 | 116 A/6 | Start | 387094 | 6608552 | End | 387094 | 6608552 |
302–303 | 115 A/11 | Start | 381700 | 6634700 | End | 382500 | 6654800 |
307, 321, 324 | 115 A/11 | Start | 371700 | 6534700 | End | 352500 | 6554800 |
316 | 115 A/11 | Start | 374700 | 6674700 | End | 377500 | 6784800 |
350 | 115 A/12 | Start | 353956 | 6740958 | End | 352956 | 6740958 |
351, 74A | 115 A/12 | Start | 352956 | 6740958 | End | 352956 | 6740958 |
352, 353 | 115 A/13 | Start | 344400 | 6745700 | End | 344300 | 6745800 |
Palynomorphs (spore and pollen) were prepared following standard extraction techniques [76], and included mudstone (4) and hemipelagite (4) samples. The degree of maturation of the palynomorphs was determined by visual comparison with the Thermal Alteration Index [77]. Conodonts were processed by the Geological Survey of Canada (Vancouver, British, Columbia, Canada), and only one limestone clast was analyzed. The degree of maturation of the recovered conodonts was determined by visual comparison with the Conodont Alteration Index [78]. Programmed temperature pyrolysis was performed by the Geological Survey of Canada (Calgary, Alberta, Canada) on a Rock-Eval 6 Turbo instrument, and comprised mudstone (12), hemipelagite (7), sandstone (1), phyllite (1), and mylonite (1) samples.
Well-exposed outcrops of the Dezadeash Formation occur throughout the St. Elias Mountains in southwestern Yukon. The majority of the outcrops are dominated by thin- to thick-bedded sandstone-mudstone couplets that form packets up to ~335 m thick, and medium- to thick-bedded sandstone that form amalgamated units up to ~136 m thick. Well preserved primary sedimentary structures are common in these lithologies, including erosional structures (sole marks and small channels), depositional structures (graded bedding, planar-stratification, and cross-stratification), deformation structures (load and flame structures, convolute stratification, dish and pillar structures, and slump structures), and biogenic sedimentary structures comprising sparse bioturbation with few discrete trace fossils preserved in the interior of beds and on the soles of beds. Detailed descriptions of these sedimentary structures and their distribution are presented in [45] and [47,79]. The pristine nature of the sedimentary structures provided unambiguous evidence for the way-up of strata, and slump folds overlain by undeformed beds confirm that the slumps are due to soft-sediment movement and not a later tectonic deformation.
Superimposed on the primary sedimentary structures are a variety of secondary structures. Sandstone beds in thin- to medium-bedded sandstones-mudstone couplets locally display a sub-vertical parting (Figure 3A). The parting is spaced 5–30 cm apart with a mean spacing of ~10.2 cm. Parting planes are parallel to each other and can be traced from one sandstone bed into several overlying or underlying sandstone beds, but they are not present in thick-bedded sandstone beds. Although the parting resembles a poorly developed spaced cleavage, the mean ratio of the separation of the partings to the thickness of the beds (s/l) is ~0.95. According to [80], s/l > 0.05 belongs to a joint set.
Mudstones are characterized by a poorly developed parting or diagenetic foliation [cf. 81], which is sub-parallel to bedding (Figure 3B). The diagenetic foliation is moderately to strongly developed, parallel to curviplanar, and generally spaced 3–8 mm, although up to 20 mm spacing is also present. The diagenetic foliation is associated with a weakly to strongly developed spaced cleavage that is at a high-angle to bedding. The cleavage domains have a spacing of 5–85 mm and are smooth, occupy < 1% of the rock volume and are parallel to curviplanar to each other. The intersection of the diagenetic foliation and spaced cleavage results in mudstones weathering into angular, irregularly-shaped pebble-sized clasts.
Rarely, well developed pencil structure is also present in the mudstone (Figure 3C) [81]. The pencil structure, formed by the intersection of well-developed diagenetic cleavage and spaced cleavage (S0-S1), trends northwesterly. The pencil length varies from 49.5–164.2 mm and the width ranges from 2.25–11.25 mm, with a mean shape factor (length-to-width ratio, L/W) of 14.5. A plot of the length and width of the pencils suggests a shortening of 9–26% (Figure 4).
Locally, mudstones display a moderately to well-developed spaced cleavage [81]. The cleavage domains have a spacing of 1–5 mm and are smooth, occupy < 1% of the rock volume and are parallel to each other (Figure 3D). The spaced cleavage appears to be roughly axial planar with north trending, upright tight folds that exhibit a fold height of several meters, but it is unclear if the folds belong to the oldest (F1) or youngest (F2) folds identified in the strata [45].
Hemipelagite beds display an irregular jointing spaced 10–20 cm (Figure 3E), resulting in the beds weathering into angular, block-shaped cobble-sized clasts. Volcaniclastic beds display a more regular jointing spaced 20–50 cm that is intersected by a very irregular second joint set that is sub-parallel to bedding (Figure 3F). As a result, the volcaniclastic beds weather into angular, irregularly-shaped boulder-sized clasts.
Several types of veins are locally present in the thin- to medium-bedded sandstones-mudstone couplets. The more common type of veining are swarms of veins that cross-cut bedding at various angles. The veins occur as differently oriented sets, often forming prominent networks, with sub-parallel veins regularly spaced ~10–20 cm. The veins are ~0.5–6 cm wide and syntaxial, with one or more phases of white quartz and minor amounts of calcite present that impart a banded appearance to the veins. Occasionally associated with the vein swarms are randomly oriented irregular vein masses, ptygma-like veins, and veinlets of milky white quartz. A less common type of veining are isolated veins and sets of veins parallel to bedding. The veins are ~4–8 cm wide and syntaxial, with a single phase of massive white quartz extending out from the wall rock of the vein.
Detailed petrography of the framework mode of sandstones and the petrography of volcaniclastic rocks (crystal and vitric tuffs) can be found in [48] and [82], respectively. Metamorphic minerals in the Dezadeash Formation were identified optically, utilizing procedures outlined in [83] for identifying phyllosilicate minerals, and with the aid of XRD analysis (Figure 5, and Table 3).
Sample | Lithology | Grain-size | Weight (%) | Quart z (%) |
Plagioclas e (%) |
Kspa r (%) | Calcit e (%) |
Muscovit e (%) |
Laumontit e (%) |
Siderit e (%) |
Kaolinit e (%) |
Chlorit e (%) |
Illit e (%) | Other (%) | Clay Mineral s (%) |
Tota l (%) |
25–3 | Sandstone | Bulk (Coarse + Cla y) Coarse (>2µm) Clay (<2µm) |
100.00 90.88 9.12 |
22 24 2 |
25 28 2 |
0 0 0 |
12 12 4 |
4 5 0 |
0 0 0 |
0 0 0 |
15 14 25 |
20 17 53 |
2 0 14 |
37 31 92 |
100 100 100 |
|
400–3 | Sandstone | Bulk Coarse Clay |
100.0094.9 6 5.04 |
24 25 1 |
37 40 1 |
0 0 0 |
2 2 0 |
2 2 0 |
0 0 0 |
0 0 0 |
11 8 51 |
15 55 35 |
9 8 12 |
34 31 98 |
100 100 100 |
|
1–3 | Hemipelagit e | Bulk Coarse Clay |
100.00 96.00 4.00 |
12 13 0 |
5 (Albite) 5 0 |
< 0.1 < 0.1 0 |
63 66 6 |
2 2 0 |
0 0 0 |
0 0 0 |
8 7 24 |
8 7 20 |
2 0 50 |
Pyrite Bassanite | 18 15 94 |
100 100 100 |
264–1 | Tuff | Bulk Coarse Clay | 100.00 94.93 5.07 |
37 37 4 |
7 (Albite) 7 2 |
0 0 0 |
6 7 1 |
3 4 0 |
41 42 33 |
3 3 0 |
0.5 0 8 |
0.5 0 8 |
2 0 44 |
2 0 60 |
100 100 100 |
|
12–5 | Mudstone | Bulk Coarse Clay | − −100 |
− −29.8 |
− −21.9 (Albite) |
− − < 0.1 |
− − 1.0 |
− − − |
− − − |
− − − |
− −0 |
− −15.9 |
− −15.3 |
< 10% expandabl e clay KI = 0.42, AI = 0.34Amorphou s (16.1%) |
− −31.2 |
− −100 |
26–1 | Mudstone | Bulk Coarse Clay | − −100 |
− −20.9 |
− −25.8 (Albite) |
− − 2.7 |
− − 4.8 |
− − 0 |
− − 0 |
− −0 |
− −0 |
− −18.8 |
− − 7.5 |
− KI = 0.26, AI = 0.33 Amorphou s (19.5%) |
− −26.3 |
− −100 |
Note: KI = Kübler Index (units of Δ°2θ); AI = Árkai Index (units of Δ°2θ). |
The dominant metamorphic mineral assemblage in sandstone is prehnite ± laumontite + chlorite + kaolinite + illite ± calcite, indicative of high temperature zeolite facies metamorphism [84]. Celadonite, pyrite, magnetite, and hematite were also observed in some thin sections. Prehnite was found in most of the thin sections and accounted for 10–50% of the thin section area. It occurs as individual grains and grain clusters that display sheaf-like structure and sweeping extinction, as well as cloudy, granular aggregates that form irregular poikilotopic patches replacing grains and matrix. Laumontite was noted in ~50% of the thin sections. It occurs as clear, irregular masses with distinct cleavage that forms poikilotopic patches replacing grains and as interstitial material. Calcite was observed in most of the thin sections. It occurs as specks of microspar in plagioclase, and as sparry cement replacing grains and grain interstices (up to 20% of the area in several thin sections). The sparry cement displays straight, thick Type II calcite twins and rare curved, thick Type III twins [cf., 85]. Type II calcite twins thick Type III twins were also observed in the coquina ("shell hash") thin sections, along with well developed microstyolites. In addition, the sandstone thin sections contain microveins of quartz-prehnite, calcite-prehnite, and calcite-quartz-prehnite, that in turn, are cross-cut by calcite microveins.
The dominant metamorphic mineral assemblage in mudstone is quartz + albite + chlorite + illite ± calcite that is non-diagnostic for determining the metamorphic facies. Variably developed K-spar, stilpnomelane, and pyrite were also observed in some thin sections. Quartz, albite, chlorite, and illite, identified by XRD (<2 µm size fraction), likely form the irresolvable turbid matrix that is too fine grained to identify optically. Stilpnomelane was observed in ~50% of thin sections of mudstones exhibiting spaced cleavage. It occurs as small (~0.1 mm long), elongated crystals that are strongly pleochroic dark brown and pale brown, and lack the "bird's eye maple" texture present in biotite. Stilpnomelane shows varying degrees of preferred alignment, accounting for the foliation in the deformed mudstones. The hemipelagite thin sections also contain microveins of calcite-pyrite. The dominant metamorphic mineral assemblage in crystal tuff is similar to that of sandstone described previously. The dominant metamorphic mineral assemblage in vitric tuff is laumontite ± prehnite + illite + chlorite + kaolinite + quartz, indicative of high temperature zeolite facies metamorphism [84]. The abundance of illite suggests that tuff has been altered to a K-bentonite [86]. Variably developed albite, palagonite, and pyrite were also observed in some thin sections. Laumontite is ubiquitous in all of the thin sections (~70% by area). It occurs as clear, irregular masses with distinct cleavage that form poikilotopic patches replacing shards and interstitial material. Prehnite was found in ~50 % of the thin sections. It comprises cloudy, granular aggregates that form irregular poikilotopic patches replacing shards and interstitial material. Thin sections of vitric tuffs also show microveins of quartz, calcite, calcite + quartz, laumontite + quartz, and calcite + quartz + laumontite + prehnite.
The < 2µm material analyzed in mudstone samples indicate that clay-size material consists mainly of albite, quartz, chlorite, and illite (the two-layer monoclinic polytype 2M1), minor amounts of calcite and K-feldspar, and rare interstratified illite/smectite (I/S) (Figure 6, and Table 3). In particular, sample GL12–5 displays < 10% interstratified I/S characterized by long-range Reichweite ordering (i.e., R3). The KI for mudstone sample GL12–5 is 0.42 Δ°2θ, and the AI is 0.34 Δ°2θ, whereas for mudstone sample GL26–1, the KI is 0.26 Δ°2θ and the AI is 0.33 Δ°2θ. Three alteration zones have been defined based on the KI: diagenetic, with KI > 0.42 Δ°2θ; anchizone, with 0.42 Δ°2θ ≤ KI ≥ 0.25 Δ°2θ; and epizone, as KI < 0.25 Δ°2θ [87,88]. The anchizone is transitional between diagenesis and metamorphism (i.e., the epizone). The diagenetic zone is further subdivided into shallow (KI > 1.0 Δ°2θ) and deep (1.0 Δ°2θ > KI > 0.42 Δ°2θ) subzones, and the anchizone is further subdivided into low (0.42 Δ°2θ < KI < 0.30 Δ°2θ) and high subzones (0.30 Δ°2θ < KI < 0.25 Δ°2θ) [89]. Boundaries for the three main KI zones have also been designated according to AI values as diagenetic, with AI > 0.33 Δ°2θ; anchizone, with 0.33 Δ°2θ ≤ AI ≥ 0.26 Δ°2θ; and epizone, with AI < 0.26 Δ°2θ [75]. Note that KI and AI are defined such that the values decrease with increasing alteration. Mudstone samples from the Dezadeash Formation indicate deep diagenetic to high anchizone conditions according to KI and AI values.
Preservation of palynomorphs extracted from the mudstone and hemipelagite samples is very poor (Table 4). Only an extremely sparse assemblage of dark brown to black, corroded silhouettes of what appear to be trilete spores, monosulcate pollen grains, and possibly one bisaccate pollen grain are present. The majority of the organic residue is dominated by palynodebris comprising: unstructured amorphous organic matter in the form of small (~20 µm), nearly equidimensional particles that are dark brown to black and semi-opaque or opaque ("black debris"); slightly larger (~50 µm), irregular "fluffy" masses appearing dark brown and partly translucent; minor amounts of dark brown globular masses possibly representing degraded Botryococcus colonies; and rare dark brown to black phytoclasts (leaf cuticles?) with no clear internal structure. The spore and pollen "wrecks" indicate a Thermal Alteration Index (TAI) of ~4.5. TAI is a semi-quantitative numerical scale from 1 to 5, with 1 being the lower maturity light color (i.e., colorless to light yellow) and 5 being the more mature darker color (i.e., black with indications of metamorphism) [77]. Hence, the TAI of ~4.5 for the Dezadeash Formation suggests mature thermal maturation.
Sample | Lithology | Spores | Pollen | AOM | Phytoclasts | Other |
1–1 | mudstone | Trilete, dark brown, corroded | Monosulcat e grain, dark brown, corroded | Black debris, round particles, dark brown to black, semi-opaque or opaque; Irregular "fluffy" masses, dark brown and partly translucent; Globular masses, dark brown, degraded Botryococcus colonies? | Black phytoclasts | Pyrite |
1–2 | mudstone | Barren | Bisaccate grain, dark brown to black, corroded | black debris, round particles, dark brown to black, semi-opaque or opaque; irregular 'fluffy' masses, dark brown and partly translucent |
||
1–3 | mudstone | Barren | Monosulcat e grain, black, corroded |
black debris, round particles, dark brown to black, semi-opaque or opaque | ||
1–4 | mudstone | Trilete, dark brown, corroded |
barren | black debris, round particles, dark brown to black, semi-opaque or opaque; irregular "fluffy" masses, dark brown and partly translucent | black phytoclasts (leaf cuticles?) | |
1–5 | hemipelag ite | Barren | Barren | black debris, round particles, dark brown to black, semi-opaque or opaque; irregular "fluffy" masses; dark brown and partly translucent | black phytoclasts (leaf cuticles?) | pyrite |
GL1–6 | hemipelag ite | Barren | Barren | black debris, round particles, dark brown to black, semi-opaque or opaque | pyrite | |
GL1–7 | hemipelag ite | Barren | Barren | black debris, round particles, dark brown to black, semi-opaque or opaque | ||
GL1–8 | hemipelag ite | Barren | Barren | black debris, round particles, dark brown to black, semi-opaque or opaque | ||
Note: AOM = amorphous organic matter. Thermal Alteration Index (TAI) = ~4.5. |
Preservation of conodonts obtained from the limestone boulder is excellent (Table 5), and includes ramiform elements, Neogondolella steinbergensis (Mosher, 1968) and Epigondolella bidentata (Mosher 1968) indicating a Late Triassic (Late Norian) age. Also present are ichthyoliths, microbivalves, and foraminifers. The conodonts reveal a Conodont Alteration Index (CAI) of ~4–4.5: CAI is also a semi-quantitative numerical scale from 1 to 5, similar in principle to the TAI, with 1 corresponding to a lower maturity light color (i.e., clear or colorless) and 5 corresponding to a more mature darker color (i.e., black) [78]. Proposed alteration zones similar to the KI and AI zones include the diacaizone, with CAI < 4, ancaizone, with 4 ≥ CAI ≤ 5.5, and epicaizone, with CAI > 5.5, with increasing CAI values corresponding to increasing alteration [89]. Hence, the CAI of ~4–4.5 for the Dezadeash Formation suggests mature thermal maturation and ancaizone conditions.
Sample | Fossils | Age | CAI |
247–1 | ichthyoliths microbivalves foraminifer conodonts: ramiform elements Neogondolella steinbergensis (Mosher, 1968) Epigondolella bidentata (Mosher 1968) |
Late Triassic (Late Norian) | 4–4.5 |
All but one sample indicates a total organic carbon (TOC) content of < 0.3 wt %, rendering the majority of the pyrolysis oven temperatures at which the maximum amounts of hydrocarbons are generated (Tmax) as suspect (Table 6). Mudstone sample GL4–8 contains 1.26% TOC and has a corresponding Tmax value of 589 ℃. Three stages of thermal maturity with respect to oil source rocks have been designated as follows: immature, with Tmax < 435 ℃ and attributed to diagenesis; mature (corresponding to the "oil window"), with Tmax 435–470 ℃ and due to catagenesis (increasing pressure and temperature); and postmature, with Tmax > 470 ℃ and indicative of metagenesis (i.e., incipient metamorphism) [90]. The one reliable sample is postmature, suggesting metagenesis conditions. Calculated parameters for this sample indicate a hydrogen index [HI = (S2/TOC) × 100] of HI = 5 and an oxygen index [OI = (S3/TOC) × 100, where S3 is the carbon dioxide generated during pyrolysis] of OI = 33, suggesting Type III (terrestrial) and IV (degraded or inert) kerogen is present. Type III kerogen is also indicated from the quantity of hydrocarbons generated by pyrolytic degradation (S2 = 0.06 mg of hydrocarbons/g of rock) and the TOC value of 1.26% when plotted on a graph of S2 versus TOC [91]. In summary, HI, OI, S2 and TOC parameters are in agreement with the type of organic matter recovered during the processing of samples for palynomorphs.
Sample | Lithology | TOC (wt%) |
Tmax (℃) | S1 (mg HC/g rock) |
S2 (mg HC/g rock) |
S3 (mg CO2/ g rock) |
HI | OI |
1–3 | Hemipelagite | 0.03 | 437 | 0.01 | 0.06 | 0.24 | 200 | 800 |
2–2 | Hemipelagite | 0.17 | 451 | 0.00 | 0.02 | 0.35 | 12 | 206 |
3–2 | Hemipelagite | 0.20 | 442 | 0.07 | 0.03 | 0.35 | 15 | 175 |
3–9 | Hemipelagite | 0.12 | 481 | 0.00 | 0.00 | 0.50 | 0 | 413 |
4–2 | Hemipelagite | 0.06 | −40 | 0.01 | 0.00 | 0.21 | 0 | 350 |
4–7 | Hemipelagite | 0.15 | 285 | 0.16 | 0.03 | 0.36 | 20 | 240 |
4–8 | Mudstone | 1.26 | 589 | 0.04 | 0.06 | 0.41 | 5 | 33 |
8–5 | Hemipelagite | 0.08 | 362 | 0.01 | 0.00 | 0.23 | 0 | 288 |
9–4 | Mudstone | 0.15 | 342 | 0.00 | 0.00 | 0.35 | 0 | 233 |
9–6 | Hemipelagite | 0.17 | 406 | 0.01 | 0.00 | 0.54 | 0 | 318 |
12–4 | Hemipelagite | 0.10 | 428 | 0.01 | 0.00 | 0.38 | 5 | 380 |
12–5 | Mudstone | 0.19 | 314 | 0.12 | 0.01 | 0.28 | 0 | 147 |
16–1 | Mudstone | 0.14 | −40 | 0.00 | 0.00 | 0.31 | 20 | 221 |
18–1 | Sandstone | 0.05 | 487 | 0.00 | 0.01 | 0.30 | 0 | 600 |
18–4 | Hemipelagite | 0.04 | −40 | 0.00 | 0.00 | 0.42 | 0 | 1050 |
22–1 | Mudstone | 0.07 | 421 | 0.00 | 0.00 | 0.35 | 14 | 500 |
23–1 | Sandstone | 0.07 | 428 | 0.00 | 0.01 | 0.24 | 0 | 343 |
26–1 | Hemipelagite | 0.07 | 430 | 0.00 | 0.00 | 0.27 | 0 | 386 |
28–1 | Phyllite | 0.01 | −40 | 0.00 | 0.00 | 0.28 | 0 | 2800 |
203–1 | Mudstone | 0.11 | −40 | 0.00 | 0.00 | 0.30 | 0 | 273 |
210–1 | Hemipelagite | 0.25 | 433 | 0.00 | 0.00 | 0.27 | 0 | 108 |
213–1 | Mudstone | 0.08 | −40 | 0.00 | 0.00 | 0.26 | 0 | 325 |
307–1 | Mylonite | 0.09 | 362 | 0.01 | 0.00 | 0.77 | 0 | 856 |
Note: TOC = total organic carbon; Tmax = Rock-Eval oven temperature at maximum S2 generation; S1 = hydrocarbons thermally distilled from sample ("free hydrocarbons"); S2 = hydrocarbons generated by pyrolytic degradation of kerogen in sample ("potential hydrocarbons"); S3 = carbon dioxide generated during pyrolysis; HI = Hydrogen Index = (S2x100/TOC); OI = Oxygen Index = (S3x100/TOC); HC = hydrocarbons |
The extremely low TOC values discouraged the use of vitrinite reflectance analysis, perhaps the most widely utilized technique for determining thermal maturity of sedimentary rocks [92]. The percent reflectivity in oil (Ro) of vitrinite (a kerogen maceral) corresponds to the stage of thermal maturity: diagenesis, with Ro < 0.5 (also referred to as immature); catagenesis, with > 0.5 Ro < 1.3 (also referred to as mature with > 0.5Ro < 1.3, and postmature with > 1.3 Ro < 2); metagenesis, with > 2Ro < 4 (also referred to as overmature); and values of Ro > 4 are in the real of metamorphism [93,94]. Several correlations between Tmax and Ro has been proposed [95,96]. The equation ln (% Rm) = (0.078 Tmax) − 1.2 converts the Tmax of 589 ℃ obtained from the Dezadeash mudstone sample to 3.8% Ro [95] and the equation Equivalent%Ro = (0.0165 Tmax) − 6.5143 converts the mudstone Tmax value of 589 ℃ to 3.2 [96]. The Tmax value of the Dezadeash sample indicates a metagenesis stage of thermal maturation (i.e., overmature), and conversion of the Tmax value to vitrinite reflectance values are consistent with the metagenesis conditions.
Multidisciplinary analysis of various mineralogic and organic thermal indicators in rocks of diverse lithologies (e.g., sandstone, mudstone, hemipelagite, and tuff) from the Dezadeash Formation are summarized in a correlation diagram (Figure 7). In particular, secondary mineral assemblages in sandstone and tuff indicate subgreenschist, high temperature zeolite facies metamorphism; Kübler indices of illite and Árkai indices of chlorite in mudstone record diagenetic to high anchizone metapelitic conditions; the color of organic matter (i.e., Thermal Alteration Index of palynomorphs and Conodont Alteration Index) and the pyrolysis of organic matter and in mudstone and hemipelagite beds suggest that thermal maturation reached catagenesis to mesogenesis stages. Correlation of the various mineralogic and organic thermal indicators is internally consistent and suggests very low-grade metamorphism (VLGM) of the Dezadeash Formation. The development of an incipient slaty cleavage (i.e., S0-S1 pencil structure) in the Dezadeash Formation is also compatible with VLGM [99,100].
Note that on the correlation diagram, illite and chlorite crystallinities document slightly higher thermal alteration than metamorphic mineral assemblages, whereas organic matter records an even slightly higher thermal alteration than illite and chlorite crystallinities. This general trend of increasing thermal alteration from zeolite facies, to diagenesis-anchizone conditions, to catagenesis- mesogenesis stages is attributed to the various "phases" (i.e., hydrous Ca-Al silicates, sheet silicates, and organic matter) reacting at different rates, or kinetics [74,87,100]. The varying reaction kinetics imply that these out-of-equilibrium phases provide only qualitative estimates of paleopressure and paleotemperature [100,101,102].
Nonetheless, based on the combination of mineralogic and organic thermal indicators observed in the Dezadeash Formation (particularly the laumontite stability field, estimates of the P-T region for the zeolite facies, the diagenesis/anchizone boundary determined from fluid inclusion data, and the effective closure temperature of zircon fission tracks), a reasonable estimate for the maximum P-T conditions experience by the strata is 2.5 ± 0.5 kbar and 250 ± 25 ℃ (Figure 8). The estimated paleopressure corresponds to a depth of 7.6–11.4 km (assuming a rock density of ρ = 2685 kg/m3). This depth is consistent with the observation of insipient spaced cleavage and widespread veining in the Dezadeash Formation, indicating that P-T conditions did not exceed the brittle-ductile transition zone that occurs at a depth of ~10–15 km and temperatures of ~250–350 ℃ [106].
Circled numbers refer to: 1) the zeolite facies metamorphism from 200–300 ℃ with total pressures below 3 kbar [83,103]; 2) the high temperature zeolite facies (i.e., Lmt with Pre) a temperature of around 230 ℃ [83]; 3a) the absence of wairakite suggests metamorphic conditions are limited by the Lmt-Wa for Ptot = PCO2, specifically P < 3 kbars and T < 300 ℃ [104,105]; 3b) the absence of lawsonite suggests metamorphic conditions limited by the Lmt-Lw equilibrium for Ptot = PCO2, specifically P < 3 kbars and T < 300 ℃ [104,105]; 4) the absence of heulandite suggests metamorphic conditions limited by the equilibrium of Heu = Lmt + Qz + W [83]; 5) the widespread veining of Qz ± Cc ± Lmt ± Pr suggests P-T conditions in the brittle ductile transition zone that occurs at 2.6–3.9 kbar (~10–15 km) and temperatures of ~250–350 ℃ [106]; 6) the laumontite dehydration equilibrium of Lmt = Wa + 2W at ~282 ± 5 ℃ and 2 kbar [107]; 7) the laumontite equilibrium of Lmt = An + 2Qz + 4W at 317 ± 10 ℃ and 2 kbar [108]; 8) the laumontite equilibrium of Lmt = Lw + 2Qz + 2W at 2.75 ± 0.25 kbar and 250 ℃ [108]; 9a)the laumontite stability field restricted to 180–285 ℃ at < 3 kbar in the NCASH system (Na2O-CaO-Al2O2-SiO2-H2O) with excess H2O and SiO2 [109]; 9b) the zeolite facies between 210–250 ℃ at 2.1–2.9 kbar [109]; 9c) the stability field of laumontite with excess Qz + W [109]; 10a) the prehnite stability of 3Pr + Chl + 4Qz +18W = 4Heu + Tr between 200–280 ℃ and < 3 kbar [102]; 10b) the prehnite stability at T > 200 ℃ and P < 2 kbar for low XCO2 [110]; 11) clay compositions containing 5–10% smectite in I/S interstratification corresponding to 200–250 ℃ [111]; 12) the R = 1 to R = 3 transition (i.e., short-range to long-range Rietveld ordering in I/S clay) at ~170–180 ℃ [112]; 13) the 1 M to 2 M polytypism transition in illite between 200–350 ℃ at ~2 kbar [113]; 14) straight thick Type II calcite twins developing in the range of 150–300 ℃ [114]; 15) the diagenesis/anchizone boundary at 240 ± 15 ℃ according to fluid inclusion data [115]; 16) the effective closure temperature (i.e., the temperature for 90% track retention) of zircon fissiontracks at ~240 ℃ [116]; 17) according to the formula Tpeak = [ln(%Ro) + 1.68]/0.0124 for estimating maximum temperatures reached by vitrinite reflectance [95], calculated equivalent reflectance values of 3.2 and 3.8 for the Dezadeash Formation correspond to temperatures of 229 ℃ and 243 ℃, respectively; 18) the diagram of nomograms of vitrinite reflectance vs. time and maximum temperature reveals that the calculated equivalent reflectance values of 3.2 and 3.8 correspond to temperatures between ~220–240 ℃ [117, their Figure 5]); 19) the Ahrrenius plot of temperature vs. time indicates the conodont CAI value of 4–4.5 for the Dezadeash Formation corresponds to a temperature of ~190–230 ℃ [78, their Figure 9]; and 20) the conodont CAI value of 4–4.5 for the Dezadeash Formation equates to a maximum temperature of 260–285 ℃ according to temperatures determined by Raman spectroscopy of carbonaceous material in conodont species [118].
The estimated maximum paleotemperature experienced by the Dezadeash Formation, together with published thermochronometric data for the strata, are plotted on a time vs. temperature diagram (Figure 9). The diagram shows that the Dezadeash Formation underwent relatively rapid, short-term heating followed by relatively gradual, long-term cooling. Based on a detrital zircon DA for Dezadeash Formation of 142 Ma [48], combined with a ZFT cooling age of ~110 Ma, strata reached peak metamorphic conditions between ~140–110 Ma. Hence the Dezadeash Formation appears to have been subject to metamorphism while the Blanchard River assemblage was being deposited (its detrital zircon maximum depositional age is 130–125 Ma) [19], and well before deposition of the Kluane Schist (its detrital zircon maximum depositional age is 94 Ma) [17]. Furthermore, the estimated peak metamorphic conditions experienced by the Dezadeash Formation appear to have coincided with the decline of the Late Jurassic-Early Cretaceous Chitina arc (~160–140 Ma) [22,34,35,36], and rise of the Late Cretaceous Chisana arc (~120–105 Ma) [16,38].
The lack of a well-developed penetrative deformation in the Dezadeash Formation suggests that burial metamorphism was responsible for the metamorphic conditions experienced by the strata [119]. The preserved compacted thickness of the Dezadeash Formation is ~3000 m. This thickness is comparable to the 3000 m thickness of the Nutzotin Mountains sequence (the proximal half of the Dezadeash Formation) that rests unconformably on the WCT and is conformably overlain by the Chisana Formation [57]. Using a normal geothermal gradient of 25 ℃/km [87], the base of the compacted Dezadeash Formation would have been subject to a temperature of only 75 ℃. Furthermore, using an estimated present-day porosity (ϕN) for the strata of 0.05 and an inferred porosity when deposited (ϕO) of 0.4 based on the porosity of shaly-sand [120], and assuming that all changes in porosity with depth are the result of compaction [121], the calculated decompacted thickness of the Dezadeash Formation at the time of deposition (TO), is ~4750 m. Again, using a normal geothermal gradient of 25 ℃/km, the base of the decompacted Dezadeash Formation would have been subject to a temperature of only 118 ℃. In addition, the stratigraphic relationship between the Dezadeash Formation and the Blanchard River assemblage is unknown [19]. Therefore, burial metamorphism seems an unlikely mechanism to account for the metamorphic grade of the Dezadeash Formation.
An alternative to burial metamorphism is "regional contact metamorphism". Regional contact metamorphism is caused by pluton intrusion and cooling over a large area, and is distinct from regional metamorphism and contact metamorphism [122,123]. This type of metamorphism has been widely recognized, and is commonly invoked for areas characterized by low grade metamorphism that lack penetrative fabrics, the presence of abundant closely spaced plutons, and an absence of a burial metamorphic mechanism [122,124,125,126,127,128,129,130,131]. The correspondence between peak metamorphic conditions experienced by the Dezadeash Formation and the existence of the Chitina and Chisana arcs suggests that regional contact metamorphism associated with coeval magmatism was a likely mechanism for metamorphism. In particular, plutonic roots of the Chitina arc are preserved as the Saint Elias plutonic suite in Yukon [37]. The Saint Elias plutonic suite has emplacement ages ranging from 160–130 Ma [37], with intrusions concentrated along the outboard margin of the WCT (corresponding to the axis of the Chitina arc, "C" in Figure 1). Plutonic roots of the Chisana arc are preserved as the Kluane Ranges plutonic suite and the Pyroxenite Creek complex in Yukon [37]. The Kluane Ranges plutonic suite and Pyroxenite Creek complex have emplacement ages between 117–106 Ma [37], with intrusions concentrated along the inboard margin of the WCT (corresponding to the axis of the Chisana arc, "A" in Figure 1). Thus, the very low-grade, subgreenschist, high temperature zeolite facies metamorphism experienced by Dezadeash Formation is attributed to regional contact metamorphism associated with emplacement of the Chitina and Chisana magmatic arcs.
The metamorphic event experienced by the Dezadeash Formation is clearly different from that preserved inthe Gravina sequence and Gravina belt in southeastern Alaska, and the Blanchard River assemblage and Kluane Schist in Yukon. Metamorphism of the Gravina sequence and Gravina belt is attributed to well documented northeast directed underthrusting of both units beneath the YCT in mid-Cretaceous time as a result of final accretion of the WCT to the North American margin [5,7,8,16,60,132,133,134], during which the Gravina sequence reached greenschist to amphibolite facies metamorphism [8], and the Gravina belt wassubject to zeolite to amphibolite facies metamorphism (with peak P-T conditions estimated to have been 8.7 ± 1 kbar, or ~25–30 km depth, and 465 ± 50 ℃ to 545 ± 75 ℃ [60,63]. Similarly, metamorphism of the Blanchard River assemblage and Kluane Schist are also attributed to northeast directed underthrusting of both units beneath the YCT in mid-Cretaceous time as a result of final accretion of the WCT to the North American margin [17,19], during which the Blanchard River assemblage reached amphibolite facies metamorphism (with peak P-T conditions estimated to have been ~6.5 kbars, or ~24 km depth assuming a rock density of 2, 750 kg/m3, and ~640 ℃) [19], and the Kluane Schist experienced greenschist to amphibolite facies metamorphism (with peak P-T conditions estimated to have been 7–8 kbar, or ~24–30 km depth, and 500 ℃) [17,64]. Furthermore, there is no compelling evidence for underthrust Mesozoic flysch in the northern part of the Gravina- Nutzotin belt [13,14,64]. Thus, the Dezadeash Formation appears to have been subject to a temporally and spatially separate metamorphic event than that experienced by the Gravina Sequence, Gravina belt, Blanchard River assemblage, and Kluane Schist.
The diverse tectonometamorphic histories of the Dezadeash Formation in the northern part of the Gravina-Nutzotin belt, compared to that of the Gravina sequence and Gravina belt in the southern part of the Gravina-Nutzotin belt, and the Blanchard River assemblage and Kluane Schist, may be a manifestation oblique convergence and diachronous accretion of the WCT to the YCT [16,26,27,28,29,30,31,32,33,135,136]. That is, underthrusting of the Gravina-Nutzotin belt decreases northward, coincident with a northward decrease in the timing of regionally extensive, mid-Cretaceous thrusting [26], suggesting that accretion of the WCT was south-to-north.
Multidisciplinary analysis of mineralogic and organic thermal indicators preserved in the Dezadeash Formation, Yukon, Canada, suggests that metamorphic mineral assemblages in sandstone and tuff indicate subgreenschist, high temperature zeolite facies metamorphism; Kübler indices of illite and Árkai indices of chlorite in mudstone record diagenetic to high anchizone metapelitic conditions; and the color of organic matter (i.e., Thermal Alteration Index of palynomorphs and Conodont Alteration Index) and pyrolysis of organic matter in mudstone and hemipelagite beds show that thermal maturation reached catagenesis to mesogenesis stages.
Based mainly on published estimates for the laumontite stability temperature, the P-T region for the zeolite facies, the temperature of the diagenesis/anchizone boundary, and the effective closure temperature of zircon fission tracks, a reasonable estimate for the maximum P-T conditions experienced by the Dezadeash Formation are 2.5 ± 0.5 kbar and 250 ± 25 ℃. The estimated paleopressure corresponds to a burial depth of 7.6–11.4 km. The estimated maximum paleotemperature experienced by the Dezadeash Formation, together with published thermochronometric data for the strata, shows that the Dezadeash Formation underwent relatively rapid, short-term heating followed by relatively gradual, long-term cooling between 140–110 Ma, attributed to regional scale contact metamorphism related to emplacement of the Jurassic-Cretaceous Chitina and Chisana magmatic arcs.
The tectonometamorphic history of the Dezadeash Formation is inconsistent with previous reconstructions of the deformation and crustal-scale structure of the Northern Cordillera that posit 756 the Dezadeash Formation was thrust to depths > 20 km beneath the Blanchard River assemblage, Kluane Schist, and YCT in the mid-Cretaceous. The tectonometamorphic history of the Dezadeash Formation also contrasts sharply with the Gravina belt and Gravina sequence in the southeastern Alaska that were apparently underthrust (~25–30 km) beneath the YCT along a regional extensive, mid-Cretaceous west-vergent thrust system. The diverse tectonometamorphic histories of the Dezadeash Formation in the northern part of the Gravina-Nutzotin belt, compared to that of the Gravina sequence and Gravina belt in the southern part of the Gravina-Nutzotin belt, may be a manifestation oblique convergence and diachronous northward accretion of the WCT to the Mesozoic margin of North America.
I wish to acknowledge that field work was undertaken in the traditional territory of the Champagne and Aishihik First Nations. Aaron Ogden, Marty Mossop, and Werner Liebau are thanked for providing field assistance in the early phases of this project. Past discussions with Stephen Johnston regarding the "Dezadeash molasse" were always entertaining. Terry Pavlis and Branimir Sergvic are thanked for their insightful comments on an earlier version of the manuscript, and two anonymous reviewers are also thanked for their helpful comments on the revised manuscript. The author declares there are no competing interests and no specific funding for this work.
The author declare no conflict of interest.
[1] |
R. Storn, K. Price, Differential evolution-A simple and efficient heuristic for global optimization over continuous spaces, J. Global Optim., 11 (1997), 341-359. doi: 10.1023/A:1008202821328
![]() |
[2] |
S. Das, P. Suganthan, Differential evolution: A survey of the state-of-the-art, IEEE Trans. Evol. Comput., 15 (2011), 4-31. doi: 10.1109/TEVC.2010.2059031
![]() |
[3] |
S. Das, S. Mullick, P. Suganthan, Recent advances in differential evolution-An updated survey, Swarm Evol. Comput., 27 (2016), 1-30. doi: 10.1016/j.swevo.2016.01.004
![]() |
[4] |
J. Jang, K. Jang, H. Kwon, J. Lee, Feedback control of an HBV model based on ensemble kalman filter and differential evolution, Math. Biosci. Eng., 15 (2018), 667-691. doi: 10.3934/mbe.2018030
![]() |
[5] |
M. Bilal, H. Zaheer, L. Garcia-Hernandez, A. Abraham, Differential evolution: A review of more than two decades of research, Eng. Appl. Artif. Intell., 90 (2020), 103479. doi: 10.1016/j.engappai.2020.103479
![]() |
[6] | K. Price, R. Storn, J. Lampinen, Differential Evolution: A Practical Approach to Global Optimization, Springer-Verlag, Berlin, 2005. |
[7] |
Y. Li, S. Wang, B. Yang, An improved differential evolution algorithm with dual mutation strategies collaboration, Expert Syst. Appl., 153 (2020), 113451. doi: 10.1016/j.eswa.2020.113451
![]() |
[8] |
J. Nelder, R. Mead, A simplex method for function minimization, Comput. J., 7 (1965), 308-313. doi: 10.1093/comjnl/7.4.308
![]() |
[9] | R. Tanabe, A. Fukunaga, Success-history based parameter adaptation for differential evolution, in 2013 IEEE Congress on Evolutionary Computation (CEC), 2013, 71-78. |
[10] |
J. Zhang, A. Sanderson, JADE: Adaptive differential evolution with optional external archive, IEEE Trans. Evol. Comput., 13 (2009), 945-958, . doi: 10.1109/TEVC.2009.2014613
![]() |
[11] | J. Zhang, A. Sanderson, Adaptive Differential Evolution: A Robust Approach to Multimodal Problem Optimization, Springer-Verlag, Berlin, 2009. |
[12] | A. Mohamed, A. Mohamed, Adaptive guided differential evolution algorithm with novel mutation for numerical optimization, Int. J. Mach. Learn. & Cyber., 10 (2019), 253-277. |
[13] |
A. Mohamed, A. Hadi, K. Jambi, Novel mutation strategy for enhancing SHADE and LSHADE algorithms for global numerical optimization, Swarm Evol. Comput., 50 (2019), 100455. doi: 10.1016/j.swevo.2018.10.006
![]() |
[14] | R. Tanabe, A. Fukunaga, Improving the search performance of SHADE using linear population size reduction, in 2014 IEEE Congress on Evolutionary Computation (CEC), 2014, 1658-1665. |
[15] |
A. Ghosh, S. Das, A. K. Das, L. Gao, Reusing the past difference vectors in differential evolution - A simple but significant improvement, IEEE Trans. on Cybern., 50 (2020), 4821-4834. doi: 10.1109/TCYB.2019.2921602
![]() |
[16] | M. Ali, M. Pant, A. Abraham, Simplex differential evolution, Acta Polytech. Hungarica., 6 (2009), 95-115. |
[17] |
Z. Gao, T. Xiao, W. Fan, Hybrid differential evolution and Nelder-Mead algorithm with re-optimization, Soft Comput., 15 (2011), 581-594. doi: 10.1007/s00500-010-0566-2
![]() |
[18] |
J. Brest, S. Greiner, B. Bošković, M. Mernik, V. Žumer, Self-adapting control parameters in differential evolution: A comparative study on numerical benchmark problems, IEEE Trans. Evol. Comput., 10 (2006), 646-657. doi: 10.1109/TEVC.2006.872133
![]() |
[19] |
X. Yao, Y. Liu, G. Lin, Evolutionary programming made faster, IEEE Trans. Evol. Comput., 3 (1999), 82-102. doi: 10.1109/4235.771163
![]() |
[20] |
S. Rahnamayan, H. Tizhoosh, M. Salama, Opposition-based differential evolution, IEEE Trans. Evol. Comput., 12 (2008), 64-79. doi: 10.1109/TEVC.2007.894200
![]() |
[21] |
M. Ali, C. Khompatraporn, Z. Zabinsky, A numerical evaluation of several stochastic algorithms on selected continuous global optimization test problems, J. Global Optim., 31 (2005), 635-672. doi: 10.1007/s10898-004-9972-2
![]() |
[22] |
W. Gong, A. Zhou, Z. Cai, A multioperator search strategy based on cheap surrogate models for evolutionary optimization, IEEE Trans. Evol. Comput., 19 (2015), 746-758. doi: 10.1109/TEVC.2015.2449293
![]() |
[23] |
Y. Wang, H. X. Li, T. Huang, L. Li, Differential evolution based on covariance matrix learning and bimodal distribution parameter setting, Appl. Soft Comput., 18 (2014), 232-247. doi: 10.1016/j.asoc.2014.01.038
![]() |
[24] |
J. Alcalá-Fdez, L. Sánchez, S. García, M. del Jesus, S. Ventura, J. M. Garrell, et al., KEEL: A software tool to assess evolutionary algorithms for data mining problems, Soft Comput., 13 (2009), 307-318. doi: 10.1007/s00500-008-0323-y
![]() |
[25] | J. Liang, B, Qu, P. Suganthan, A. Hernández-Díaz, Problem definitions and evaluation criteria for the CEC 2013 special session and competition on real-parameter optimization, Technical Report 201212, Computational Intelligence Laboratory, Zhengzhou University, 2013. |
[26] |
N. Qiu, Q. Liu, Q. Gao, Q. Zeng, Combining genetic algorithm and generalized least squares for geophysical potential field data optimized inversion, IEEE Geosci. Remote. Sens. Lett., 7 (2010), 660-664. doi: 10.1109/LGRS.2010.2045152
![]() |
[27] |
C. Hu, J. Cai, D. Zeng, X. Yan, W. Gong, L. Wang, Deep reinforcement learning based valve scheduling for pollution isolation in water distribution network, Math. Biosci. Eng., 17 (2020), 105-121. doi: 10.3934/mbe.2020006
![]() |
[28] |
A. Alejo-Reyes, E. Olivares-Benitez, A. Mendoza, A. Rodriguez, Inventory replenishment decision model for the supplier selection problem using metaheuristic algorithms, Math. Biosci. Eng., 17 (2020), 2016-2036. doi: 10.3934/mbe.2020107
![]() |
[29] |
X. Peng, H. Jia, C. Lang, Modified dragonfly algorithm based multilevel thresholding method for color images segmentation, Math. Biosci. Eng., 16 (2019), 6467-6511. doi: 10.3934/mbe.2019324
![]() |
![]() |
![]() |
Sample | Lithology | Elevation (m) | AHe (Ma) | ZHe (Ma) | AFT (Ma) | ZFT (Ma) |
KLB04 | metapelite | 605 | 17.8 ± 1.6 | 59.7 ± 8.1 | n.a. | n.a. |
KLB91 | mudstone | 725 | 11.4 ± 0.8 | 68.8 ± 2.3 | n.a. | 109.9 ± 8.9 |
Tc (℃) | 70 | 180 | 120 | 240 | ||
PRZ (℃) | 40–70 | 130–180 | n.a. | n.a. | ||
PAZ (℃) | n.a. | n.a. | 60–120 | 180–240 | ||
Note: Samples KLB04 and KLB91 from [51]. •AHe = apatite (U-Th)/He, ZHe = zircon (U-Th)/He, AFT = apatite fission-track, and ZFT = zircon fission-track thermochronometer age; •Tc = closure temperature (radioactive decay product retained below this temperature, but not above it; also referred to as the temperature of a mineral at the time given by its radiometric age); •PRZ = partial retention zone (temperature window over which radioactive decay product is retained); •PAZ = partial annealing zone (temperature window over which radioactive decay product is preserved). Closure temperatures from [52,53]. Note that the temperatures are only approximate because they depend on the cooling rate, chemistry of the mineral, and grain-size of the mineral [52]. |
Section | NTS Map | Easting | Northing | Easting | Northing | ||
1 | 115 A/11 | Start | 371000 | 6719500 | End | 369200 | 6718000 |
2 | 115 A/11 | Start | 371600 | 6718700 | End | 371200 | 6718600 |
3 | 115 A/11 | Start | 370400 | 6720800 | End | 369200 | 6720500 |
4 | 115 A/11 | Start | 369700 | 6716600 | End | 369200 | 6716200 |
5, 264 | 115 A/11 | Start | 369700 | 6716500 | End | 369400 | 6716700 |
6 | 115 A/11 | Start | 370500 | 6716300 | End | 370300 | 6716600 |
7 | 115 A/11 | Start | 369500 | 6715600 | End | 369300 | 6716000 |
8 | 115 A/11 | Start | 370300 | 6717000 | End | 369400 | 6717500 |
9 | 115 A/11 | Start | 370600 | 6717800 | End | 369800 | 6718300 |
10 | 115 A/11 | Start | 370900 | 6718300 | End | 370400 | 6718200 |
12 | 115 A/11 | Start | 369300 | 6715700 | End | 368500 | 6716700 |
13 | 115 A/11 | Start | 385400 | 6702500 | End | 383600 | 6702300 |
14A | 115 A/11 | Start | 385500 | 6699300 | End | 384800 | 6699200 |
14B | 115 A/11 | Start | 384700 | 6698800 | End | 384300 | 6698700 |
16 | 115 A/11 | Start | 381200 | 6698200 | End | 380700 | 6698200 |
17 | 115 A/11 | Start | 383300 | 6696900 | End | 384200 | 6697700 |
18 | 115 A/11 | Start | 385100 | 6703900 | End | 384100 | 6703800 |
19 | 115 A/11 | Start | 377000 | 6715000 | End | 376200 | 6714300 |
20 | 115 A/11 | Start | 382500 | 6687700 | End | 382300 | 6687300 |
21B | 115 A/11 | Start | 384300 | 6695700 | End | 384300 | 6695700 |
22 | 115 A/11 | Start | 384700 | 7604600 | End | 384500 | 6705000 |
23 | 115 A/11 | Start | 384300 | 6705400 | End | 383800 | 6705900 |
24 | 115 A/11 | Start | 384700 | 6684700 | End | 384500 | 6684800 |
25 | 115 A/11 | Start | 382200 | 6709300 | End | 381400 | 6708700 |
26 | 115 A/11 | Start | 377200 | 6716300 | End | 382400 | 6715700 |
27, 224, 228 | 115 A/2 | Start | 392000 | 6665100 | End | 392000 | 6665100 |
28 | 115 A/2 | Start | 392100 | 6675100 | End | 392100 | 6676100 |
57, 231 | A/2 | Start | 398000 | 6685100 | End | 398800 | 6688100 |
201–202 | 115 A/6 | Start | 371800 | 6701400 | End | 371800 | 6701400 |
203–206 | 115 A/6 | Start | 373800 | 6711400 | End | 372800 | 6711400 |
207–208 | 115 A/6 | Start | 371200 | 6701400 | End | 371200 | 6701400 |
211–217 | 115 A/6 | Start | 372200 | 6711400 | End | 372200 | 6711400 |
219–222 | 115 A/6 | Start | 373000 | 6708700 | End | 373000 | 6708700 |
240–246 | 115 A/12 | Start | 358200 | 6717500 | End | 358200 | 6717500 |
247 | 115 A/12 | Start | 359400 | 6717700 | End | 359400 | 6717700 |
250–252, 261 | 115 A/12 | Start | 358500 | 6725000 | End | 358500 | 6725000 |
254, 260 | 115 A/12 | Start | 361600 | 6724500 | End | 360700 | 6725300 |
257 | 115 A/12 | Start | 358600 | 6724700 | End | 358600 | 6724700 |
280, 74B, 400 | 116 A/6 | Start | 387094 | 6608552 | End | 387094 | 6608552 |
302–303 | 115 A/11 | Start | 381700 | 6634700 | End | 382500 | 6654800 |
307, 321, 324 | 115 A/11 | Start | 371700 | 6534700 | End | 352500 | 6554800 |
316 | 115 A/11 | Start | 374700 | 6674700 | End | 377500 | 6784800 |
350 | 115 A/12 | Start | 353956 | 6740958 | End | 352956 | 6740958 |
351, 74A | 115 A/12 | Start | 352956 | 6740958 | End | 352956 | 6740958 |
352, 353 | 115 A/13 | Start | 344400 | 6745700 | End | 344300 | 6745800 |
Sample | Lithology | Grain-size | Weight (%) | Quart z (%) |
Plagioclas e (%) |
Kspa r (%) | Calcit e (%) |
Muscovit e (%) |
Laumontit e (%) |
Siderit e (%) |
Kaolinit e (%) |
Chlorit e (%) |
Illit e (%) | Other (%) | Clay Mineral s (%) |
Tota l (%) |
25–3 | Sandstone | Bulk (Coarse + Cla y) Coarse (>2µm) Clay (<2µm) |
100.00 90.88 9.12 |
22 24 2 |
25 28 2 |
0 0 0 |
12 12 4 |
4 5 0 |
0 0 0 |
0 0 0 |
15 14 25 |
20 17 53 |
2 0 14 |
37 31 92 |
100 100 100 |
|
400–3 | Sandstone | Bulk Coarse Clay |
100.0094.9 6 5.04 |
24 25 1 |
37 40 1 |
0 0 0 |
2 2 0 |
2 2 0 |
0 0 0 |
0 0 0 |
11 8 51 |
15 55 35 |
9 8 12 |
34 31 98 |
100 100 100 |
|
1–3 | Hemipelagit e | Bulk Coarse Clay |
100.00 96.00 4.00 |
12 13 0 |
5 (Albite) 5 0 |
< 0.1 < 0.1 0 |
63 66 6 |
2 2 0 |
0 0 0 |
0 0 0 |
8 7 24 |
8 7 20 |
2 0 50 |
Pyrite Bassanite | 18 15 94 |
100 100 100 |
264–1 | Tuff | Bulk Coarse Clay | 100.00 94.93 5.07 |
37 37 4 |
7 (Albite) 7 2 |
0 0 0 |
6 7 1 |
3 4 0 |
41 42 33 |
3 3 0 |
0.5 0 8 |
0.5 0 8 |
2 0 44 |
2 0 60 |
100 100 100 |
|
12–5 | Mudstone | Bulk Coarse Clay | − −100 |
− −29.8 |
− −21.9 (Albite) |
− − < 0.1 |
− − 1.0 |
− − − |
− − − |
− − − |
− −0 |
− −15.9 |
− −15.3 |
< 10% expandabl e clay KI = 0.42, AI = 0.34Amorphou s (16.1%) |
− −31.2 |
− −100 |
26–1 | Mudstone | Bulk Coarse Clay | − −100 |
− −20.9 |
− −25.8 (Albite) |
− − 2.7 |
− − 4.8 |
− − 0 |
− − 0 |
− −0 |
− −0 |
− −18.8 |
− − 7.5 |
− KI = 0.26, AI = 0.33 Amorphou s (19.5%) |
− −26.3 |
− −100 |
Note: KI = Kübler Index (units of Δ°2θ); AI = Árkai Index (units of Δ°2θ). |
Sample | Lithology | Spores | Pollen | AOM | Phytoclasts | Other |
1–1 | mudstone | Trilete, dark brown, corroded | Monosulcat e grain, dark brown, corroded | Black debris, round particles, dark brown to black, semi-opaque or opaque; Irregular "fluffy" masses, dark brown and partly translucent; Globular masses, dark brown, degraded Botryococcus colonies? | Black phytoclasts | Pyrite |
1–2 | mudstone | Barren | Bisaccate grain, dark brown to black, corroded | black debris, round particles, dark brown to black, semi-opaque or opaque; irregular 'fluffy' masses, dark brown and partly translucent |
||
1–3 | mudstone | Barren | Monosulcat e grain, black, corroded |
black debris, round particles, dark brown to black, semi-opaque or opaque | ||
1–4 | mudstone | Trilete, dark brown, corroded |
barren | black debris, round particles, dark brown to black, semi-opaque or opaque; irregular "fluffy" masses, dark brown and partly translucent | black phytoclasts (leaf cuticles?) | |
1–5 | hemipelag ite | Barren | Barren | black debris, round particles, dark brown to black, semi-opaque or opaque; irregular "fluffy" masses; dark brown and partly translucent | black phytoclasts (leaf cuticles?) | pyrite |
GL1–6 | hemipelag ite | Barren | Barren | black debris, round particles, dark brown to black, semi-opaque or opaque | pyrite | |
GL1–7 | hemipelag ite | Barren | Barren | black debris, round particles, dark brown to black, semi-opaque or opaque | ||
GL1–8 | hemipelag ite | Barren | Barren | black debris, round particles, dark brown to black, semi-opaque or opaque | ||
Note: AOM = amorphous organic matter. Thermal Alteration Index (TAI) = ~4.5. |
Sample | Fossils | Age | CAI |
247–1 | ichthyoliths microbivalves foraminifer conodonts: ramiform elements Neogondolella steinbergensis (Mosher, 1968) Epigondolella bidentata (Mosher 1968) |
Late Triassic (Late Norian) | 4–4.5 |
Sample | Lithology | TOC (wt%) |
Tmax (℃) | S1 (mg HC/g rock) |
S2 (mg HC/g rock) |
S3 (mg CO2/ g rock) |
HI | OI |
1–3 | Hemipelagite | 0.03 | 437 | 0.01 | 0.06 | 0.24 | 200 | 800 |
2–2 | Hemipelagite | 0.17 | 451 | 0.00 | 0.02 | 0.35 | 12 | 206 |
3–2 | Hemipelagite | 0.20 | 442 | 0.07 | 0.03 | 0.35 | 15 | 175 |
3–9 | Hemipelagite | 0.12 | 481 | 0.00 | 0.00 | 0.50 | 0 | 413 |
4–2 | Hemipelagite | 0.06 | −40 | 0.01 | 0.00 | 0.21 | 0 | 350 |
4–7 | Hemipelagite | 0.15 | 285 | 0.16 | 0.03 | 0.36 | 20 | 240 |
4–8 | Mudstone | 1.26 | 589 | 0.04 | 0.06 | 0.41 | 5 | 33 |
8–5 | Hemipelagite | 0.08 | 362 | 0.01 | 0.00 | 0.23 | 0 | 288 |
9–4 | Mudstone | 0.15 | 342 | 0.00 | 0.00 | 0.35 | 0 | 233 |
9–6 | Hemipelagite | 0.17 | 406 | 0.01 | 0.00 | 0.54 | 0 | 318 |
12–4 | Hemipelagite | 0.10 | 428 | 0.01 | 0.00 | 0.38 | 5 | 380 |
12–5 | Mudstone | 0.19 | 314 | 0.12 | 0.01 | 0.28 | 0 | 147 |
16–1 | Mudstone | 0.14 | −40 | 0.00 | 0.00 | 0.31 | 20 | 221 |
18–1 | Sandstone | 0.05 | 487 | 0.00 | 0.01 | 0.30 | 0 | 600 |
18–4 | Hemipelagite | 0.04 | −40 | 0.00 | 0.00 | 0.42 | 0 | 1050 |
22–1 | Mudstone | 0.07 | 421 | 0.00 | 0.00 | 0.35 | 14 | 500 |
23–1 | Sandstone | 0.07 | 428 | 0.00 | 0.01 | 0.24 | 0 | 343 |
26–1 | Hemipelagite | 0.07 | 430 | 0.00 | 0.00 | 0.27 | 0 | 386 |
28–1 | Phyllite | 0.01 | −40 | 0.00 | 0.00 | 0.28 | 0 | 2800 |
203–1 | Mudstone | 0.11 | −40 | 0.00 | 0.00 | 0.30 | 0 | 273 |
210–1 | Hemipelagite | 0.25 | 433 | 0.00 | 0.00 | 0.27 | 0 | 108 |
213–1 | Mudstone | 0.08 | −40 | 0.00 | 0.00 | 0.26 | 0 | 325 |
307–1 | Mylonite | 0.09 | 362 | 0.01 | 0.00 | 0.77 | 0 | 856 |
Note: TOC = total organic carbon; Tmax = Rock-Eval oven temperature at maximum S2 generation; S1 = hydrocarbons thermally distilled from sample ("free hydrocarbons"); S2 = hydrocarbons generated by pyrolytic degradation of kerogen in sample ("potential hydrocarbons"); S3 = carbon dioxide generated during pyrolysis; HI = Hydrogen Index = (S2x100/TOC); OI = Oxygen Index = (S3x100/TOC); HC = hydrocarbons |
Sample | Lithology | Elevation (m) | AHe (Ma) | ZHe (Ma) | AFT (Ma) | ZFT (Ma) |
KLB04 | metapelite | 605 | 17.8 ± 1.6 | 59.7 ± 8.1 | n.a. | n.a. |
KLB91 | mudstone | 725 | 11.4 ± 0.8 | 68.8 ± 2.3 | n.a. | 109.9 ± 8.9 |
Tc (℃) | 70 | 180 | 120 | 240 | ||
PRZ (℃) | 40–70 | 130–180 | n.a. | n.a. | ||
PAZ (℃) | n.a. | n.a. | 60–120 | 180–240 | ||
Note: Samples KLB04 and KLB91 from [51]. •AHe = apatite (U-Th)/He, ZHe = zircon (U-Th)/He, AFT = apatite fission-track, and ZFT = zircon fission-track thermochronometer age; •Tc = closure temperature (radioactive decay product retained below this temperature, but not above it; also referred to as the temperature of a mineral at the time given by its radiometric age); •PRZ = partial retention zone (temperature window over which radioactive decay product is retained); •PAZ = partial annealing zone (temperature window over which radioactive decay product is preserved). Closure temperatures from [52,53]. Note that the temperatures are only approximate because they depend on the cooling rate, chemistry of the mineral, and grain-size of the mineral [52]. |
Section | NTS Map | Easting | Northing | Easting | Northing | ||
1 | 115 A/11 | Start | 371000 | 6719500 | End | 369200 | 6718000 |
2 | 115 A/11 | Start | 371600 | 6718700 | End | 371200 | 6718600 |
3 | 115 A/11 | Start | 370400 | 6720800 | End | 369200 | 6720500 |
4 | 115 A/11 | Start | 369700 | 6716600 | End | 369200 | 6716200 |
5, 264 | 115 A/11 | Start | 369700 | 6716500 | End | 369400 | 6716700 |
6 | 115 A/11 | Start | 370500 | 6716300 | End | 370300 | 6716600 |
7 | 115 A/11 | Start | 369500 | 6715600 | End | 369300 | 6716000 |
8 | 115 A/11 | Start | 370300 | 6717000 | End | 369400 | 6717500 |
9 | 115 A/11 | Start | 370600 | 6717800 | End | 369800 | 6718300 |
10 | 115 A/11 | Start | 370900 | 6718300 | End | 370400 | 6718200 |
12 | 115 A/11 | Start | 369300 | 6715700 | End | 368500 | 6716700 |
13 | 115 A/11 | Start | 385400 | 6702500 | End | 383600 | 6702300 |
14A | 115 A/11 | Start | 385500 | 6699300 | End | 384800 | 6699200 |
14B | 115 A/11 | Start | 384700 | 6698800 | End | 384300 | 6698700 |
16 | 115 A/11 | Start | 381200 | 6698200 | End | 380700 | 6698200 |
17 | 115 A/11 | Start | 383300 | 6696900 | End | 384200 | 6697700 |
18 | 115 A/11 | Start | 385100 | 6703900 | End | 384100 | 6703800 |
19 | 115 A/11 | Start | 377000 | 6715000 | End | 376200 | 6714300 |
20 | 115 A/11 | Start | 382500 | 6687700 | End | 382300 | 6687300 |
21B | 115 A/11 | Start | 384300 | 6695700 | End | 384300 | 6695700 |
22 | 115 A/11 | Start | 384700 | 7604600 | End | 384500 | 6705000 |
23 | 115 A/11 | Start | 384300 | 6705400 | End | 383800 | 6705900 |
24 | 115 A/11 | Start | 384700 | 6684700 | End | 384500 | 6684800 |
25 | 115 A/11 | Start | 382200 | 6709300 | End | 381400 | 6708700 |
26 | 115 A/11 | Start | 377200 | 6716300 | End | 382400 | 6715700 |
27, 224, 228 | 115 A/2 | Start | 392000 | 6665100 | End | 392000 | 6665100 |
28 | 115 A/2 | Start | 392100 | 6675100 | End | 392100 | 6676100 |
57, 231 | A/2 | Start | 398000 | 6685100 | End | 398800 | 6688100 |
201–202 | 115 A/6 | Start | 371800 | 6701400 | End | 371800 | 6701400 |
203–206 | 115 A/6 | Start | 373800 | 6711400 | End | 372800 | 6711400 |
207–208 | 115 A/6 | Start | 371200 | 6701400 | End | 371200 | 6701400 |
211–217 | 115 A/6 | Start | 372200 | 6711400 | End | 372200 | 6711400 |
219–222 | 115 A/6 | Start | 373000 | 6708700 | End | 373000 | 6708700 |
240–246 | 115 A/12 | Start | 358200 | 6717500 | End | 358200 | 6717500 |
247 | 115 A/12 | Start | 359400 | 6717700 | End | 359400 | 6717700 |
250–252, 261 | 115 A/12 | Start | 358500 | 6725000 | End | 358500 | 6725000 |
254, 260 | 115 A/12 | Start | 361600 | 6724500 | End | 360700 | 6725300 |
257 | 115 A/12 | Start | 358600 | 6724700 | End | 358600 | 6724700 |
280, 74B, 400 | 116 A/6 | Start | 387094 | 6608552 | End | 387094 | 6608552 |
302–303 | 115 A/11 | Start | 381700 | 6634700 | End | 382500 | 6654800 |
307, 321, 324 | 115 A/11 | Start | 371700 | 6534700 | End | 352500 | 6554800 |
316 | 115 A/11 | Start | 374700 | 6674700 | End | 377500 | 6784800 |
350 | 115 A/12 | Start | 353956 | 6740958 | End | 352956 | 6740958 |
351, 74A | 115 A/12 | Start | 352956 | 6740958 | End | 352956 | 6740958 |
352, 353 | 115 A/13 | Start | 344400 | 6745700 | End | 344300 | 6745800 |
Sample | Lithology | Grain-size | Weight (%) | Quart z (%) |
Plagioclas e (%) |
Kspa r (%) | Calcit e (%) |
Muscovit e (%) |
Laumontit e (%) |
Siderit e (%) |
Kaolinit e (%) |
Chlorit e (%) |
Illit e (%) | Other (%) | Clay Mineral s (%) |
Tota l (%) |
25–3 | Sandstone | Bulk (Coarse + Cla y) Coarse (>2µm) Clay (<2µm) |
100.00 90.88 9.12 |
22 24 2 |
25 28 2 |
0 0 0 |
12 12 4 |
4 5 0 |
0 0 0 |
0 0 0 |
15 14 25 |
20 17 53 |
2 0 14 |
37 31 92 |
100 100 100 |
|
400–3 | Sandstone | Bulk Coarse Clay |
100.0094.9 6 5.04 |
24 25 1 |
37 40 1 |
0 0 0 |
2 2 0 |
2 2 0 |
0 0 0 |
0 0 0 |
11 8 51 |
15 55 35 |
9 8 12 |
34 31 98 |
100 100 100 |
|
1–3 | Hemipelagit e | Bulk Coarse Clay |
100.00 96.00 4.00 |
12 13 0 |
5 (Albite) 5 0 |
< 0.1 < 0.1 0 |
63 66 6 |
2 2 0 |
0 0 0 |
0 0 0 |
8 7 24 |
8 7 20 |
2 0 50 |
Pyrite Bassanite | 18 15 94 |
100 100 100 |
264–1 | Tuff | Bulk Coarse Clay | 100.00 94.93 5.07 |
37 37 4 |
7 (Albite) 7 2 |
0 0 0 |
6 7 1 |
3 4 0 |
41 42 33 |
3 3 0 |
0.5 0 8 |
0.5 0 8 |
2 0 44 |
2 0 60 |
100 100 100 |
|
12–5 | Mudstone | Bulk Coarse Clay | − −100 |
− −29.8 |
− −21.9 (Albite) |
− − < 0.1 |
− − 1.0 |
− − − |
− − − |
− − − |
− −0 |
− −15.9 |
− −15.3 |
< 10% expandabl e clay KI = 0.42, AI = 0.34Amorphou s (16.1%) |
− −31.2 |
− −100 |
26–1 | Mudstone | Bulk Coarse Clay | − −100 |
− −20.9 |
− −25.8 (Albite) |
− − 2.7 |
− − 4.8 |
− − 0 |
− − 0 |
− −0 |
− −0 |
− −18.8 |
− − 7.5 |
− KI = 0.26, AI = 0.33 Amorphou s (19.5%) |
− −26.3 |
− −100 |
Note: KI = Kübler Index (units of Δ°2θ); AI = Árkai Index (units of Δ°2θ). |
Sample | Lithology | Spores | Pollen | AOM | Phytoclasts | Other |
1–1 | mudstone | Trilete, dark brown, corroded | Monosulcat e grain, dark brown, corroded | Black debris, round particles, dark brown to black, semi-opaque or opaque; Irregular "fluffy" masses, dark brown and partly translucent; Globular masses, dark brown, degraded Botryococcus colonies? | Black phytoclasts | Pyrite |
1–2 | mudstone | Barren | Bisaccate grain, dark brown to black, corroded | black debris, round particles, dark brown to black, semi-opaque or opaque; irregular 'fluffy' masses, dark brown and partly translucent |
||
1–3 | mudstone | Barren | Monosulcat e grain, black, corroded |
black debris, round particles, dark brown to black, semi-opaque or opaque | ||
1–4 | mudstone | Trilete, dark brown, corroded |
barren | black debris, round particles, dark brown to black, semi-opaque or opaque; irregular "fluffy" masses, dark brown and partly translucent | black phytoclasts (leaf cuticles?) | |
1–5 | hemipelag ite | Barren | Barren | black debris, round particles, dark brown to black, semi-opaque or opaque; irregular "fluffy" masses; dark brown and partly translucent | black phytoclasts (leaf cuticles?) | pyrite |
GL1–6 | hemipelag ite | Barren | Barren | black debris, round particles, dark brown to black, semi-opaque or opaque | pyrite | |
GL1–7 | hemipelag ite | Barren | Barren | black debris, round particles, dark brown to black, semi-opaque or opaque | ||
GL1–8 | hemipelag ite | Barren | Barren | black debris, round particles, dark brown to black, semi-opaque or opaque | ||
Note: AOM = amorphous organic matter. Thermal Alteration Index (TAI) = ~4.5. |
Sample | Fossils | Age | CAI |
247–1 | ichthyoliths microbivalves foraminifer conodonts: ramiform elements Neogondolella steinbergensis (Mosher, 1968) Epigondolella bidentata (Mosher 1968) |
Late Triassic (Late Norian) | 4–4.5 |
Sample | Lithology | TOC (wt%) |
Tmax (℃) | S1 (mg HC/g rock) |
S2 (mg HC/g rock) |
S3 (mg CO2/ g rock) |
HI | OI |
1–3 | Hemipelagite | 0.03 | 437 | 0.01 | 0.06 | 0.24 | 200 | 800 |
2–2 | Hemipelagite | 0.17 | 451 | 0.00 | 0.02 | 0.35 | 12 | 206 |
3–2 | Hemipelagite | 0.20 | 442 | 0.07 | 0.03 | 0.35 | 15 | 175 |
3–9 | Hemipelagite | 0.12 | 481 | 0.00 | 0.00 | 0.50 | 0 | 413 |
4–2 | Hemipelagite | 0.06 | −40 | 0.01 | 0.00 | 0.21 | 0 | 350 |
4–7 | Hemipelagite | 0.15 | 285 | 0.16 | 0.03 | 0.36 | 20 | 240 |
4–8 | Mudstone | 1.26 | 589 | 0.04 | 0.06 | 0.41 | 5 | 33 |
8–5 | Hemipelagite | 0.08 | 362 | 0.01 | 0.00 | 0.23 | 0 | 288 |
9–4 | Mudstone | 0.15 | 342 | 0.00 | 0.00 | 0.35 | 0 | 233 |
9–6 | Hemipelagite | 0.17 | 406 | 0.01 | 0.00 | 0.54 | 0 | 318 |
12–4 | Hemipelagite | 0.10 | 428 | 0.01 | 0.00 | 0.38 | 5 | 380 |
12–5 | Mudstone | 0.19 | 314 | 0.12 | 0.01 | 0.28 | 0 | 147 |
16–1 | Mudstone | 0.14 | −40 | 0.00 | 0.00 | 0.31 | 20 | 221 |
18–1 | Sandstone | 0.05 | 487 | 0.00 | 0.01 | 0.30 | 0 | 600 |
18–4 | Hemipelagite | 0.04 | −40 | 0.00 | 0.00 | 0.42 | 0 | 1050 |
22–1 | Mudstone | 0.07 | 421 | 0.00 | 0.00 | 0.35 | 14 | 500 |
23–1 | Sandstone | 0.07 | 428 | 0.00 | 0.01 | 0.24 | 0 | 343 |
26–1 | Hemipelagite | 0.07 | 430 | 0.00 | 0.00 | 0.27 | 0 | 386 |
28–1 | Phyllite | 0.01 | −40 | 0.00 | 0.00 | 0.28 | 0 | 2800 |
203–1 | Mudstone | 0.11 | −40 | 0.00 | 0.00 | 0.30 | 0 | 273 |
210–1 | Hemipelagite | 0.25 | 433 | 0.00 | 0.00 | 0.27 | 0 | 108 |
213–1 | Mudstone | 0.08 | −40 | 0.00 | 0.00 | 0.26 | 0 | 325 |
307–1 | Mylonite | 0.09 | 362 | 0.01 | 0.00 | 0.77 | 0 | 856 |
Note: TOC = total organic carbon; Tmax = Rock-Eval oven temperature at maximum S2 generation; S1 = hydrocarbons thermally distilled from sample ("free hydrocarbons"); S2 = hydrocarbons generated by pyrolytic degradation of kerogen in sample ("potential hydrocarbons"); S3 = carbon dioxide generated during pyrolysis; HI = Hydrogen Index = (S2x100/TOC); OI = Oxygen Index = (S3x100/TOC); HC = hydrocarbons |