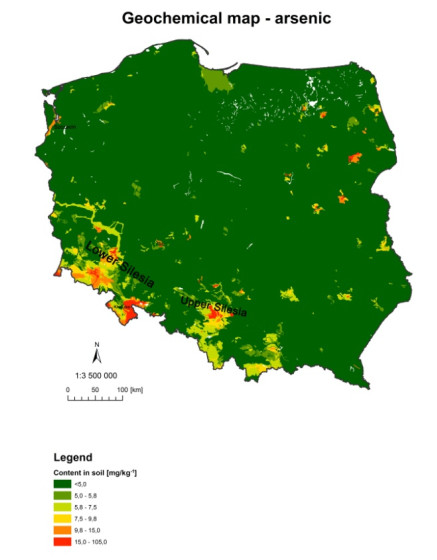
Citation: Jolanta Kwiatkowska-Malina, Andrzej Szymon Borkowski. Geostatistical modelling of soil contamination with arsenic, cadmium, lead, and nickel: the Silesian voivodeship, Poland case study[J]. AIMS Geosciences, 2020, 6(2): 135-148. doi: 10.3934/geosci.2020009
[1] | Stefano Albanese, Annalise Guarino . Assessing contamination sources and environmental hazards for potentially toxic elements and organic compounds in the soils of a heavily anthropized area: the case study of the Acerra plain (Southern Italy). AIMS Geosciences, 2022, 8(4): 552-578. doi: 10.3934/geosci.2022030 |
[2] | Alessia Nannoni, Federica Meloni, Marco Benvenuti, Jacopo Cabassi, Francesco Ciani, Pilario Costagliola, Silvia Fornasaro, Pierfranco Lattanzi, Marta Lazzaroni, Barbara Nisi, Guia Morelli, Valentina Rimondi, Orlando Vaselli . Environmental impact of past Hg mining activities in the Monte Amiata district, Italy: A summary of recent studies. AIMS Geosciences, 2022, 8(4): 525-551. doi: 10.3934/geosci.2022029 |
[3] | Jianzhao Yin, Haoyu Yin, Yuhong Chao, Hongyun Shi . Energy and tellurium deposits. AIMS Geosciences, 2024, 10(1): 28-42. doi: 10.3934/geosci.2024002 |
[4] | Diaka Sidibé, Mamadou Diallo, Ahmed Amara Konaté, Muhammad Zaheer . Geotechnical investigations of lead-silver ore processing residues at the Auzelles site, Auvergne (France). AIMS Geosciences, 2024, 10(4): 735-758. doi: 10.3934/geosci.2024036 |
[5] | Trevor B. Kelly, Grant D. Wach, Darragh E. O'Connor . The technical challenges and outcomes of ground-penetrating radar: A site-specific example from Joggins, Nova Scotia. AIMS Geosciences, 2021, 7(1): 22-55. doi: 10.3934/geosci.2021002 |
[6] | Maurizio Barbieri, Tiziano Boschetti, Giuseppe Sappa, Francesca Andrei . Hydrogeochemistry and groundwater quality assessment in a municipal solid waste landfill (central Italy). AIMS Geosciences, 2022, 8(3): 467-487. doi: 10.3934/geosci.2022026 |
[7] | Armin Salsani, Abdolhossein Amini, Shahram shariati, Seyed Ali Aghanabati, Mohsen Aleali . Geochemistry, facies characteristics and palaeoenvironmental conditions of the storm-dominated phosphate-bearing deposits of eastern Tethyan Ocean; A case study from Zagros region, SW Iran. AIMS Geosciences, 2020, 6(3): 316-354. doi: 10.3934/geosci.2020019 |
[8] | Eve-Agnès Fiorentino, Sheldon Warden, Maksim Bano, Pascal Sailhac, Thomas Perrier . One-off geophysical detection of chlorinated DNAPL during remediation of an industrial site: a case study. AIMS Geosciences, 2021, 7(1): 1-21. doi: 10.3934/geosci.2021001 |
[9] | Firoz Ahmad, Laxmi Goparaju . Soil and Water Conservation Prioritization Using Geospatial Technology – a Case Study of Part of Subarnarekha Basin, Jharkhand, India. AIMS Geosciences, 2017, 3(3): 375-395. doi: 10.3934/geosci.2017.3.375 |
[10] | Li Ding, Yubing Wu, Xuechao Liu, Fenfen Liu, Harry Mei . Application of Microbial Geochemical Exploration Technology in Identifying Hydrocarbon Potential of Stratigraphic Traps in Junggar Basin, China. AIMS Geosciences, 2017, 3(4): 576-589. doi: 10.3934/geosci.2017.4.576 |
Contamination of the environment among others with heavy metals considerably has increased over the last centuries as a result of rapid civilisation development, and particularly urbanisation and industrialisation [1]. Due to this, environmental management is an object of interest of scientists, authorities, and entities dealing with environmental protection [2]. Data concerning the spatial distribution of soil pollution with heavy metals are of great importance for the environmental protection, regional development, and spatial planning [3]. Owing to geostatistical and spatial information systems, commonly used in many areas of the economy, the majority of data from the existing resources can be presented with no loss of valuable information [4,5]. The application of geostatistics at the beginning have been reserved for the estimation of mineral and petroleum resources [6]. Recently geostatistics is used in oceanography, cartography, meteorology, agriculture, fisheries resources, civil engineering, finance, as well as the environment, especially in the contaminated sites [7,8,9,10,11]. The determination of the sources of anthropopressure requires the understanding of the spatial distribution of heavy metals in soils [10,11]. The approach based on the spatial information system is useful for the description of such structures [10,11]. Better understanding of the scale of soil pollution with heavy metals can be facilitated by geochemical cartography [11,12]. By means of maps, it presents the distribution of chemical elements in soils, bottom sediments, surface water, and groundwater [13,14]. It presents data which should be considered among the most important criteria in defining limitations of various anthropogenic activities [15]. Moreover, it is one of the most useful tools for the implementation of plans of environmental interventions [16,17]. Soil pollution with heavy metals is particularly strenuous in areas concentrating industry related to coal and liquid fuels extraction, mining and smelting industry, chemical industry, energy production, agriculture, and transport [11,18,19]. According to research conducted by the Polish Geological Institute-National Research Institute (PGI-NRI), soils in certain regions of Poland are strongly polluted with heavy metals particularly hazardous for human health and life, such as arsenic, cadmium, nickel, and lead, and the soil pollution persists considerably longer than air and water pollution—even up to several centuries.
Data concerning the content of heavy metals can be presented in the form of a cartographic study (map) resulting from their interpolation in a specific point. The first stage involves interpolation followed by agammaegation of values obtained as a result of interpolation, within designated geological segments. Agammaegation results in ascribing a designated geological segment with a mean or median of the content of an element (substance)—depending on the distribution of a given element [11,20].
The main aim of the study was the analysis of the content of selected heavy metals: arsenic, cadmium, nickel, and lead in soils in Poland, identification of degraded areas and the emitters of contamination, as well as the assessment of the degree of soil contamination with arsenic, cadmium, nickel, and lead. The specific aims of the work were to use geostatistical methods to estimate the degree of heavy metal soil pollution caused by dry and/or wet deposition from atmospheric air, to determine the relationship between the level of heavy metal pollution in atmospheric air and soils for the purposes of assessing their quality and determining the possibility of assessment damage to the soil environment in the province of Silesia in Poland.
The maps were prepared based on Web Map Services (WMS) provided by the Polish Geological Institute-National Research Institute (PGI-NRI), with the application of GIS software. Maps of soil contamination with selected heavy metals: arsenic, cadmium, lead, and nickel, were prepared as a combination of the interpolation of their content in soils with the agammaegation of values within designated geological segments. As a result of agammaegation, a designated geological segment is ascribed with a mean or median of the content of an element—depending on the spatial distribution of a given element. The resulting maps allow for considerably easier and credible interpretation of results in comparison to maps prepared in the standard way, i.e. as a result of application of interpolation only.
In order to check whether there is accumulation of contaminants in the top soil layer (0–30 cm), the deposition of selected heavy metals (As, Cd, Ni, Pb) was calculated, which depending on such factors as geographic location and volume of emissions in a given area may be 40–70% of the total lead content in soil [21,22]. Calculations of total deposition were made as the sum of dry and wet deposition. There are many gas dry deposition models that require different types of input data to estimate a dry deposition stream. One of them is the model of dry deposition (Ⅰ) ISC (The Industrial Source Complex), which is based on the dry deposition algorithm [23], included in the ADOM model (Acid Deposition and Oxidant Model). This algorithm was chosen as one of the best in an independent type assessment by the Environmental Protection Agency in 1994. In the case of wet deposition, the chemical transport model was used [24].
Measurements related to air quality in Poland have been conducted since 2000, and the collected data are collected as part of the State Environmental Monitoring (SEM) by the Chief Inspectorate for Environmental Protection (CIEP). The air quality monitoring system performs the following functions: (ⅰ) information, (ⅱ) forecasting, (ⅲ) warning, (ⅳ) emission control. The system’s task is to systematically inform administrative and public authorities as well as to support environmental management. As part of air monitoring, data concerning the levels of selected air pollutants in individual voivodeships are performed, analyzed and collected. The atmospheric air tests concern the determination of the following substances: sulfur dioxide (SO2), nitrogen oxides (NO2, NO, NOx), ozone, PM10 dust, PM2.5 dust, carbon monoxide, benzene. In addition, heavy metal content (arsenic, cadmium, nickel, lead) and benzo (a) pyrene are determined in PM10. For this reason, these heavy metals have been selected for analysis. In the most heavily contaminated heavy metals in the Silesian voivodeship (Figure 6), the average share of the phenomenon of dry and wet deposition in the total contamination of the top layer (0–30 cm) of soil with lead was calculated. 5 measurement points for the chemistry of Polish arable soils were selected for the calculations, in which, in 5-year periods in the years 1995–2010, steadily growing soil contamination with lead was recorded.
Arsenic in small amounts is a component of proper human and animal diet. However, in larger doses, it is a toxic element. Long-term contact with it (for example through inhalation) leads to the serious health problem such as, chronic disorders of the nervous, respiratory, or circulatory system [25,26].
In the environment, arsenic occurs in atmospheric air and in various minerals. Arsenic compounds are easily diluted in water, and therefore they are quickly migrating to the hydrosphere [27]. The main source of soil, water, and air contamination with arsenic and its compounds is industry related to coal and liquid fuels extraction, mining, smelting industry, and agriculture. The presence of arsenic is determined to a considerably lower degree by natural factors such as volcano eruptions and microbiological processes. The content of arsenic in soils can vary from 1 to 30 mg·kg-1 [28]. In strongly polluted regions (industrial areas, large urban agglomerations), the content of arsenic can exceed such values even tenfold.
Soils the most contaminated with arsenic are closely related to human activity (Figure 1). The largest area of soils with a high arsenic content is located near the arsenic ore mine closed in 1960. Arsenic ores are no longer extracted due to lack of demand [29]. Areas with an equally high arsenic content in the soil are around brown coal mines and copper smelters. Copper ores contain considerable amounts of arsenic which is released in smelting processes to the air and soils [30].
Another large area strongly polluted with arsenic is the Upper Silesian Industrial Area. The occurrence of the element in the region is related to the intensity of hard coal extraction, highly developed smelting and metallurgical industry, as well as pollution from the Upper Silesian conurbation. In the remaining areas of Poland, the content of arsenic in soils is maintained on a low level, with the exception of single areas of large cities.
Cadmium is an exceptionally toxic element (many times more than arsenic), accumulating in the human body. It strongly damages the kidneys and circulatory system, and blocks the circulation of microelements in the organism. In pregnant women, it can cause strong fetal disorders. Moreover, the removal of cadmium from the organism is a very time-consuming process [31].
Cadmium commonly occurs in soils. Its average content usually does not exceed 0.2 mg·kg-1. Cadmium usually occurs in the environment in the form of chemical compounds, e.g. in ores of zinc, lead, and copper. Cadmium and its compounds are easily diluted in water, which facilitates its transport in the environment. In the vicinity of industrial areas (particularly metal ore extraction) and waste dumps, its considerable accumulation may occur. The accumulation of cadmium, also in atmospheric air and water, is caused by the application of mineral fertilisers and/or sewage sludge, and high intensity of road traffic. Natural processes such as volcano eruptions and precipitation contribute to the presence of cadmium to a lower degree. Cadmium is very easily absorbed by plants, also consumption plants. This results in its introduction into the trophic chain [32,33,34,35].
The largest area in Poland characterized with high cadmium content in soils is located in Upper Silesia (Figure 2). Such high content of cadmium, similarly as in the case of occurrence of arsenic, is particularly caused by highly developed mining and smelting industry. Another source of pollution with cadmium in the region is the strongly developed road and railway network.
Apart from Upper Silesia, the territory of Poland includes several other small areas with high content of cadmium in soils. This is related to the functioning of the refinery and oil processing plant producing high amounts of substances harmful for the environment. The content of cadmium in soil in the immediate vicinity of the industrial plants amounts reaches 12 mg·kg-1, and the area of direct impact is considerably larger (up to several tens of km in each direction). Another area, also related to fuel industry and mountains, with one of the largest in the world so far functioning oil mining centre—branch of PGNiG in Sanok.
Nickel is one of the most common elements on Earth. It predominantly occurs in the form of minerals. It is supplied to soils and groundwater from atmospheric air through dry and/or wet deposition. Its presence in the atmosphere results from the processes of coal, oil, and waste combustion, as well as steel production and plating processes.
Nickel can be carcinogenic, and cause skin allergies [36]. It is introduced to the human body particularly through the respiratory system [37].
Areas with high content of nickel in soils are rare in Poland. Somewhat more nickel is contained in the soils of the southern part of Poland (Figure 3). Soils with high nickel content occur in areas with high content of arsenic. This suggests similar causes of its occurrence. In Lower Silesia, the sources of nickel in the soil are mines and copper smelters, and in Upper Silesia—coal extraction and smelting industry. In addition, high nickel content in soil occurs in the Podkarpackie Voivodeship. Includes industrial plants dealing with glassworks. Higher than the average nickel content in soil is also found in the vicinity of Krakow, where “NowaHuta” is located—the second largest steel smelter in Poland, as well as near the oil processing plant. In the remaining areas of the country, the content of nickel in soils does not exceed 7.2 mg·kg-1.
Lead is highly harmful for the human body. It disturbs the functioning of the kidneys, liver, and respiratory system [38]. It is a mutagenic element. It can be introduced to the organism through skin, the respiratory system, or through the digestive system through the infection of consumption plants. It is accumulated in the human body and is also harmful for plants—it disturbs the processes of photosynthesis and cell division [39].
Lead, in small amounts commonly occurs in soils, atmospheric air, and water. The natural content of lead in soils amounts to 20 mg·kg-1. The primary causes of the presence of higher than average content of lead in soils is particularly metal smelting and glassworks, oil industry, production of pesticides, and traffic pollution.
Similarly as in the case of nickel, somewhat more lead in soils occurs in the southern, more industrialised part of Poland. Even there, however, its content in soils does not exceed 45 mg·kg-1 (Figure 4). In the northern part of Poland, the content of lead oscillates around 20 mg·kg-1. The area of Upper Silesia is subject to the strongest pollution. The content of lead in soils in the region considerably exceeds the admissible norms (100 mg·kg-1) and reaches up to 470 mg·kg-1. This is related to the developed mining and smelting industry and transport. An increased content of lead also occurs in the Lower Silesia voivodeship in relation to the presence of a coal and copper mine and copper smelters, as well as in the vicinity of industrial plants in Mielec.
Cumulative maps usually convey one single content: They represent, on a qualitative, ordinal or quantitative scale, the level of risk at each point. Therefore, although largely conventional, they are rather unambiguous, easy to interpret and to convey to non-experts for use in decision support, compared with sets of separate maps for single causes of risk [40]. Soils polluted as a result of anthropogenic activity occupy 3% of the territory of Poland [41]. The concentration of the mentioned pollutants (their total) occurs in the zones of developed mining, smelting industry and in areas of heavy industry. It is therefore the southern part of Poland, especially almost the entire area of Upper Silesia, the vicinity of the copper mine in Lower Silesia, the Krakow agglomeration and the vicinity of industrial plants in south-eastern Poland. In the northern part of the country, the areas with the largest heavy metal pollution include those where the oil industry is developing.
Podzol, rusty, and lessive soils occupy approximately 80% of the area of Poland (Figure 5). They commonly occur in lowland areas, and in smaller amounts in uplands and mountains (particularly podzols). Chernozems are the most resistant to degradation, including pollution with heavy metals, occupy only approximately 1% of the area of Poland.
The Institute of Soil Science and Plant Cultivation—State Research Institute (ISSPC-SRI) in Puławy carries out assessments of soil quality in the scope of pollution with heavy metals in five-year periods. According to the guidelines of the ISSPC, the degree of soil pollution with heavy metals is determined on a six-degree scale (from 0 to Ⅴ), where: 0 means the natural content of the element in the soil, and Ⅴ means strongly polluted soils, Ⅰ—increased content of heavy metals—all field crops are allowed with limited vegetables for children, Ⅱ—poorly contaminated soils—vegetables are excluded, crops of cereals, root and fodder crops are allowed, Ⅲ—medium contaminated—industrial crops and seed grass are recommended, Ⅳ—heavily contaminated—it is allowed to grow industrial plants, plants for the production of seed, energy plants, Ⅴ—very heavily contaminated—it is allowed to grow flax, hemp, oilseed rape and wicker [42].
The results of monitoring research on the chemism of agricultural soils suggest that soils in Poland do not show content of heavy metals exceeding the norms. The large majority of soils show natural content of heavy metals. The results of research of PGI-NRI show that the content of selected heavy metals in soils suggests [42]:
● Ⅱ degree of contamination with lead (100–500 mg Pb·kg-1 of dry soil mass)—occurring over approximately 3% of the area of Poland,
● Ⅰ degree of contamination with cadmium (1–3 mg Cd·kg-1 of dry soil mass)—occurring over approximately 10 % of the area of Poland,
● Ⅰ degree of contamination with nickel (30–75 mg Ni·kg-1 of dry soil mass)—occurring over approximately 3% of the area of Poland.
Arsenic was not subject to classification due to the lack of a sufficient number of samples.
In the most heavily contaminated heavy metals in the Silesian voivodeship (Figures 6 and 7), a systematic increase in soil pollution by lead was recorded (Table 1).For this purpose, geostatistical methods were used, in particular the cokriging tool integrating with each other the data of the examined variable (air quality) and auxiliary variables (precipitation and DTM).
Point number | Place | Soil type | Lead content [mg·kg-1] | |||
1995 | 2000 | 2005 | 2010 | |||
329 | Czernica | brown leached | 10.7 | 13.9 | 14.3 | 17.5 |
409 | Goczałkowice (earlier Ćwiklice) | lessive | 27.2 | 28.3 | 31.2 | 34.7 |
335 | Piekary Śląskie | brown rendzinas | 448.3 | 473.3 | 486.5 | 549.5 |
411 | Aleksandrowice | black proper earths | 46.1 | 50.8 | 48.4 | 55.9 |
415 | Żywiec | brown river alluvial | 18.8 | 20.3 | 21.3 | 23.8 |
The completed calculations were used to map soil contamination with selected heavy metals. Over 20 measuring points for monitoring the chemistry of arable soils in Poland were used for interpolation (cokriging). In the case of auxiliary variables of precipitation, it was over 1000 points. As part of the research, the total lead content in soil was added in 2015 (monitoring data for Polish arable soils) and the calculated lead deposition in 2015. As a result of map algebra, maps of the forecast for soil contamination with lead were obtained in 2016 (Figure 8).
Based on the results of analyzes carried out for the Silesian voivodeship, a systematic increase in soil pollution by lead can be identified as a result of the deposition of dry and wet lead from atmospheric air. This is a disturbing signal not only for institutions monitoring and controlling the condition of soil quality, but also for local, regional and central authorities. Based on calculations according to the chemical transport model, the dry deposition algorithm and the estimation of the data examined, it was shown that the lead deposition charge in atmospheric air (up to 8 mg/m2), in the Silesian voivodeship, in 2015, several times exceeds the permissible lead content in Polish law (up to 2 mg/m2), on the basis of the lead soil contamination forecast in the Silesian Province for 2016, a further accumulation of lead in the soil (up to more than 500 ppm) is predicted, where for a number of years the permissible content in Poland has been exceeded lead in soil (up to 100 ppm for arable land).
Geochemical cartography permits the visual assessment of the degree of soil pollution with heavy metals. The cartographic presentation of study results by means of maps facilitates the identification of particular phenomena in space. Maps prepared by means of the interpolation method in combination with data agammaegation within designated geological segments consider both the spatial variability of contents of the analysed elements during interpolation and their spatial delineation with boundaries of different geological segments in the scope of agammaegation. The performed analyses permitted generating maps of content of selected heavy metals in soils and the determination of correlations between areas characterised by excessive content of heavy metals and location of emitters responsible for the pollution. It was evidenced that areas the most polluted with heavy metals in Poland and the industrial areas of Upper and Lower Silesia and areas of large cities related to industry (for example Krakow).
Geostatistics introduces new enabling tools to many fields of science for the interpretation of data in space and obtaining information necessary in the decision-making process. Sophisticated geostatistical methods such as e.g. cokriging can be used in many studies of the natural environment. The obtained test results confirm the usefulness of geostatistical analyzes in the study of the state of atmospheric air pollution and soils, where as a result of the analyzes it is possible to obtain precise and reliable information on the state of contamination of the studied area. The spatial distribution of pollutants, including heavy metals, in the soil environment is a very important information when drawing up thematic maps, eg. geochemical and sozological maps, which can be used, inter alia, in sustainable remediation for the purposes of rational environmental protection and spatial planning.
All authors declare no conflicts of interest in this paper.
[1] |
Modrzewska B, Wyszkowski M (2014) Trace metals content in soils along the state road 51 (north-eastern Poland). Environ Monit Assess 186: 2589-2597. doi: 10.1007/s10661-013-3562-z
![]() |
[2] |
Osakwe SA (2013) Chemical partitioning of iron, cadmium, nickel and chromium in contaminated soils of south-eastern Nigeria. Chem Speciation Bioavailability 25: 71-78. doi: 10.3184/095422913X13581872822530
![]() |
[3] |
Bień JD, Meer J, Rulkens WH, et al. (2005) A GIS-based approach for the long-term prediction of human health risks at contaminated sites. Environ Model Assess 9: 221-226. doi: 10.1007/s10666-005-0909-z
![]() |
[4] |
Jalali M, Karami S, Marj AF (2016) Geostatistical Evaluation of Spatial Variation Related to Groundwater Quality Database: Case Study for Arak Plain Aquifer, Iran. Environ Model Assess 21: 707-719. doi: 10.1007/s10666-016-9506-6
![]() |
[5] |
Krami LK, Amiri F, Sefiyanian A, et al. (2013) Spatial patterns of heavy metals in soil under different geological structures and land uses for assessing metal enrichments. Environ Monit Assess 185: 9871-9888. doi: 10.1007/s10661-013-3298-9
![]() |
[6] | Goovaerts P (1997) Geostatistics for natural resources evaluation. Oxford Uni. Press, New York, 483. |
[7] |
Lin WC, Lin YP, Wang YC (2016) A decision-making approach for delineating sites which are potentially contaminated by heavy metals via joint simulation. Environ Pollut 211: 98-110. doi: 10.1016/j.envpol.2015.12.030
![]() |
[8] |
Shen F, Liao R, Ali A, et al. (2017) Spatial distribution and risk assessment of heavy metals in soil near a Pb/Zn smelter in Feng County, China. Ecotoxicol Environ Saf 139: 254-262. doi: 10.1016/j.ecoenv.2017.01.044
![]() |
[9] |
Xie Y, Chen TB, Lei M, et al. (2011) Spatial distribution of soil heavy metal pollution estimated by different interpolation methods: Accuracy and uncertainty analysis. Chemosphere 82: 468-476. doi: 10.1016/j.chemosphere.2010.09.053
![]() |
[10] |
Zawadzki J, Fabjańczyk P (2012) Geostatistical evaluation of lead and zinc concentration in soils of an old mining area with complex land management. Int J Environ Sci Technol 10: 729-742 doi: 10.1007/s13762-012-0132-9
![]() |
[11] | Namysłowska-Wilczyńska B (2019) Application of Geostatistical Techniques for the Determining of an Anomalous Zone of Copper Ore Deposit in the Area of Polkowice Mine (Region of Lubin-Sieroszowice, SW Part of Poland). Geoinfor Geostat Overv 7: 1-22 |
[12] |
Namysłowska-Wilczyńska B, Wilczyński A (2002) Multivariate estimation and simulationfor environmental data modelling: processing of heavy metals concentration data in soil. Data Sci J 1: 27-44 doi: 10.2481/dsj.1.27
![]() |
[13] | Ripin SNM, Hasan S, Kamal M (2014) Environmental Geochemical Mapping on Distribution of Metal Contamination in Topsoils Perlis, Malaysia. J Med Bioeng 3: 277-281. |
[14] |
Cai L, Xu Z, Bao P, et al. (2015). Multivariate and geostatistical analyses of the spatial distribution and source of arsenic and heavy metals in the agricultural soils in Shunde, Southeast China. J Geochem Explor 148: 189-195. doi: 10.1016/j.gexplo.2014.09.010
![]() |
[15] | Salminen R, Tarvainen T, Demetriades A, et al. (1998) FOREGS Geochemical Mapping Field Manual. Geological Survey of Finland. |
[16] | De Vivo B, Lima A, Bove AM, et al. (2006) Environmental Geochemical Atlas of Italy. European Congress on Regional Geoscientific Cartography and Earth Information and Systems Water, 126-127. |
[17] |
Guillén MT, Delgado J, Albanese S, et al. (2011) Environmental geochemical mapping of Huelva municipality soils (SW Spain) as a tool to determine background and baseline values. J Geochem Explor 109: 59-69. doi: 10.1016/j.gexplo.2011.03.003
![]() |
[18] |
Frentiu T, Ponta M, Levei E, et al. (2008) Preliminary study on heavy metals contamination of soil using solid phase speciation and the influence on groundwater in Bozanta-Baia Mare Area, Romania. Chem Speciation Bioavailability 20: 99-109. doi: 10.3184/095422908X324471
![]() |
[19] |
Malina G (2004) Ecotoxicological and environmental problems associated with the former chemical plant in Tarnowskie Gory, Poland. Toxicology 205: 157-172. doi: 10.1016/j.tox.2004.06.064
![]() |
[20] |
Saito H, Goovaerts P (2000) Geostatistical Interpolation of Positively Skewed and Censored Data in a Dioxin-Contaminated Site. Environ Sci Technol 34: 4228-4235. doi: 10.1021/es991450y
![]() |
[21] |
Fowler D, Skiba U, Nemitz E, et al. (2004). Measuring Aerosol and Heavy Metal Deposition on Urban Woodland and Grass Using Inventories of 210Pb and Metal Concentrations in Soil. Water Air Soil Pollut Focus 4: 483-499. doi: 10.1023/B:WAFO.0000028373.02470.ba
![]() |
[22] | Brunner PH, Rechberger H (2016) Practical Handbook of Material Flow Analysis. CRC Press, 173-178. |
[23] | Pleim J, Venkatram A, Yamartino R (1984) ADOM/TADAM model development program. ERT P-b980-520, prepared for OME AES of Canada and the Umweltbundesamt, West Germany, 111. |
[24] | Sportisse B (2007) A review of parameterizations for modelling dry deposition and scavenging of radionuclides, Atmos Environ 41: 2683-2698. |
[25] |
Hopenhayn C (2006) Arsenic in Drinking Water: Impact on Human Health. Elements 2: 103-107. doi: 10.2113/gselements.2.2.103
![]() |
[26] |
Kapaj S, Peterson H, Liber K, et al. (2006) Human Health Effects From Chronic Arsenic Poisoning-A Review. J Environ Sci Health Part A Toxic/Hazard Subst Environ Eng 41: 2399-2428. doi: 10.1080/10934520600873571
![]() |
[27] |
Zawierucha I, Kozłowski C, Malina G (2016) Immobilized materials for removal of toxic metal ions from surface/groundwaters and aqueous waste streams. Environ Sci Processes Impacts 18: 429. doi: 10.1039/C5EM00670H
![]() |
[28] | Kucharczak-Moryl E, Moryl A, Żmuda R (2014) Environment influence arsenic content in croplands in the zgorzelecki-bogatyński region. Ecological Engineering, No 37: 107-116. Available from: http://www.ineko.net.pl/Wplyw-srodowiska-na-zawartosc-arsenu-w-glebach-uprawnych-rejonu-zgorzelecko-bogatynskiego,283,0,1.html. Accessed 5 May 2016. |
[29] | Mikulski S (2010) Gold, arsenic and dopeore. Polish Geological Institute-National Research Institute. |
[30] | Kuys K, Ralston J, Sobieraj S, et al. (1989) Methods for the enrichment of bauxite ultrafines. Physicochem Metall Probl 21: 23-26. |
[31] | Bernard A (2008) Cadmium & its adverse effects on human health. Indian J Med Res 128: 557-564. |
[32] | Kabata-Pendias A, Piotrkowska M (1997) Transfer of cadmium, zinc and lead from soils to plants. The 5th international conference proceedings: transport, fate and effects of silver in the environment, 171-176. |
[33] | Kwiatkowska-Malina J, Maciejewska A (2013) Uptake of heavy metals by darnel multifloral (Loliummultiflorum Lam.) at diverse soil reaction and organic matter content. Soil Sci Annu 64: 19-23. |
[34] | Maciejewska A, Kwiatkowska J (2001) Contamination of soil with heavy metals along highways as well as in the city of Warsaw, In Obieg pierwiastków w przyrodzie (in Polish), Instytut Ochrony Środowiska: 49-54. |
[35] | Maciejewska A, Ociepa E, Kwiatkowska J (2003) Changes in the availability of heavy metals and content of organic carbon in polluted soil after the application of an organic-mineral fertilizer obtained from brown coal. Pol J Soil Sci 36:129-136. |
[36] | Duda-Chodak A, Błaszczyk U (2008) The impact of nickel on human health. J Elem 13: 685-696. |
[37] | Cempel M, Nikel G (2006) Nickel: A Review of Its Sources and Environmental Toxicology. Pol J Environ Stud 15: 375-382. |
[38] |
Järup L, Åkesson A (2009) Current status of cadmium as an environmental health problem. Toxicol Appl Pharmacol 238: 201-208. doi: 10.1016/j.taap.2009.04.020
![]() |
[39] |
Mohammed AS, Kapri A, Goel R (2011) Heavy Metal Pollution: Source, Impact, and Remedies: Biomanagement of Metal-Contaminated Soils. Environ Pollut 20: 1-28. doi: 10.1007/978-94-007-1914-9_1
![]() |
[40] |
Pistocchi A, Groenwold J, Lahr J, et al. (2011) Mapping Cumulative Environmental Risks: Examples from the EU NoMiracle Project. Environ Model Assess 16: 119-133. doi: 10.1007/s10666-010-9230-6
![]() |
[41] | Strzelecki R (2004) Directions and problems of abiotic environment research in Poland conducted by the State Geological Survey. Pol Geol Rev 52: 716-719. |
[42] | Institute of Soil Science and Plant Cultivation-State Research Institute (2010) The assessment of soil contamination in Poland. Available from http://www.iung.pulawy.pl/index.php?option=com_content&view=article&id=147:zanieczyszczenia&catid=45:oferta&Itemid=115. Accessed 11 June 2016. |
1. | Andrew N. Amenaghawon, Chinedu L. Anyalewechi, Handoko Darmokoesoemo, Heri Septya Kusuma, Hydroxyapatite-based adsorbents: Applications in sequestering heavy metals and dyes, 2022, 302, 03014797, 113989, 10.1016/j.jenvman.2021.113989 | |
2. | Arnab Saha, Bhaskar Sen Gupta, Sandhya Patidar, Nadia Martínez-Villegas, Spatial distribution based on optimal interpolation techniques and assessment of contamination risk for toxic metals in the surface soil, 2022, 115, 08959811, 103763, 10.1016/j.jsames.2022.103763 | |
3. | Nur Shuhada Tajudin, Mazidah Mazidah Zulkifli, Mohd Fuad Miskon, Mohamad Izzuddin Anuar, Zulkifli Hashim, Fikriah Faudzi, Nurul Mayzaitul Azwa Jamaluddin, Integrated Approach of Heavy Metal Evaluation Using Geostatistical and Pollution Assessment Index in Soil of Bauxite Mining Area, 2022, 30, 2231-8526, 1545, 10.47836/pjst.30.2.38 | |
4. | Božena Skoko, Milica Kovačić, Maja Lazarus, Ankica Sekovanić, Jelena Kovačić, Agnieszka Sergiel, Tomasz Zwijacz-Kozica, Slaven Reljić, Branko Petrinec, Nuria Selva, Đuro Huber, 90Sr and stable element levels in bones of brown bears: long-term trends in bear populations from Croatia and Poland, 2022, 0944-1344, 10.1007/s11356-022-24397-4 | |
5. | Reynolds Yvan Abende Sayom, Martin Luther Mfenjou, Mouhamed Ayiwouo Ngounouno, Michele Maguy Cathya Etoundi, William André Boroh, Luc Leroy Mambou Ngueyep, Arsene Meying, A coupled geostatistical and machine learning approach to address spatial prediction of trace metals and pollution indices in sediments of the abandoned gold mining site of Bekao, Adamawa, Cameroon, 2023, 9, 24058440, e18511, 10.1016/j.heliyon.2023.e18511 | |
6. | Amar Kumar Kathwas, Pranata Hazra, Rakesh Saur, Barnali Saha, Loveraj Singh, Leela Gariya, Shruti Kumari, , 2023, Chapter 5, 978-3-031-45299-4, 119, 10.1007/978-3-031-45300-7_5 |
Point number | Place | Soil type | Lead content [mg·kg-1] | |||
1995 | 2000 | 2005 | 2010 | |||
329 | Czernica | brown leached | 10.7 | 13.9 | 14.3 | 17.5 |
409 | Goczałkowice (earlier Ćwiklice) | lessive | 27.2 | 28.3 | 31.2 | 34.7 |
335 | Piekary Śląskie | brown rendzinas | 448.3 | 473.3 | 486.5 | 549.5 |
411 | Aleksandrowice | black proper earths | 46.1 | 50.8 | 48.4 | 55.9 |
415 | Żywiec | brown river alluvial | 18.8 | 20.3 | 21.3 | 23.8 |
Point number | Place | Soil type | Lead content [mg·kg-1] | |||
1995 | 2000 | 2005 | 2010 | |||
329 | Czernica | brown leached | 10.7 | 13.9 | 14.3 | 17.5 |
409 | Goczałkowice (earlier Ćwiklice) | lessive | 27.2 | 28.3 | 31.2 | 34.7 |
335 | Piekary Śląskie | brown rendzinas | 448.3 | 473.3 | 486.5 | 549.5 |
411 | Aleksandrowice | black proper earths | 46.1 | 50.8 | 48.4 | 55.9 |
415 | Żywiec | brown river alluvial | 18.8 | 20.3 | 21.3 | 23.8 |