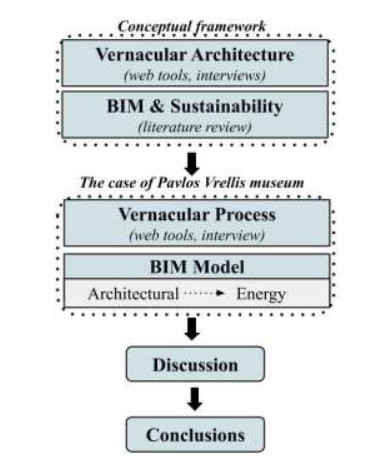
Citation: Christina Priavolou. To BIM or not to BIM? Lessons learned from a Greek vernacular museum building[J]. AIMS Environmental Science, 2020, 7(2): 192-207. doi: 10.3934/environsci.2020012
[1] | Saad S. Alrwashdeh . The Effect of Environmental Albedo on the Energy Use of a Selected House in Amman-Jordan. AIMS Environmental Science, 2023, 10(5): 628-643. doi: 10.3934/environsci.2023035 |
[2] | Miguel Mora-Pérez, Ignacio Guillen-Guillamón, Petra Amparo López-Jiménez . A CFD study for evaluating the effects of natural ventilation on indoor comfort conditions. AIMS Environmental Science, 2017, 4(2): 289-309. doi: 10.3934/environsci.2017.2.289 |
[3] | Lars-Åke Mikaelsson, Jonas Jonasson . Sustainable Built Environment in Mid Sweden: Case study based models for sustainable building and construction processes. AIMS Environmental Science, 2021, 8(1): 47-59. doi: 10.3934/environsci.2021004 |
[4] | Dimitrios Kotzias . Built environment and indoor air quality: The case of volatile organic compounds. AIMS Environmental Science, 2021, 8(2): 135-147. doi: 10.3934/environsci.2021010 |
[5] | Jana Söderlund, Peter Newman . Biophilic architecture: a review of the rationale and outcomes. AIMS Environmental Science, 2015, 2(4): 950-969. doi: 10.3934/environsci.2015.4.950 |
[6] | Adriano Magliocco, Katia Perini . The perception of green integrated into architecture: installation of a green facade in Genoa, Italy. AIMS Environmental Science, 2015, 2(4): 899-909. doi: 10.3934/environsci.2015.4.899 |
[7] | Vanessa Duarte Pinto, Catarina Martins, José Rodrigues, Manuela Pires Rosa . Improving access to greenspaces in the Mediterranean city of Faro. AIMS Environmental Science, 2020, 7(3): 226-246. doi: 10.3934/environsci.2020014 |
[8] | Siti Rachmawati, Syafrudin, Budiyono, Ellyna Chairani, Iwan Suryadi . Life cycle analysis and environmental cost-benefit assessment of utilizing hospital medical waste into heavy metal safe paving blocks. AIMS Environmental Science, 2024, 11(5): 665-681. doi: 10.3934/environsci.2024033 |
[9] | Shenwei Yu, Mengqiu Yang . Research on the applicability of passive house technology in areas hot in summer and cold in winter-take Nanjing area as the research object. AIMS Environmental Science, 2019, 6(6): 460-471. doi: 10.3934/environsci.2019.6.460 |
[10] | Ik-Whan G. Kwon, Nina Shin, Sung-Ho Kim, Hamed Usman . Trust and commitment in supply chain during digital transformation: A case in Korea. AIMS Environmental Science, 2021, 8(6): 641-655. doi: 10.3934/environsci.2021040 |
The impact of buildings on the environment has been gaining attention over the last decades [1]. The conventional way of producing buildings has been notorious for its significant amounts of waste [2]. Indicatively, demolition processes account for 40% of total solid waste, while the operation stage of buildings produces 30% of the total greenhouse gases [3,4]. Hence, the need to improve the life cycle performance of buildings is imperative.
In an attempt to enhance sustainability in the construction sector, the adaptation of practices that improve the energy and environmental performance of buildings has been suggested [5]. The sustainability of buildings is based on factors such as water conservation, energy efficiency, and appropriateness of building materials, which are influenced by architectural planning. To this end, there is an urgent need to create new design models through the implementation of an integrated strategy [6].
The attention of the global construction industry is geared toward approaches that reduce construction and operation costs while improving the quality of buildings [7]. Design decisions regarding energy consumption have a great impact on the life cycle of buildings given the long operation stage of buildings [8]. Some scholars argue that Building Information Modeling (BIM) offers the opportunity to achieve these goals via time and money savings [9,10]. Nevertheless, others [11,12] are sceptical in regard to the adoption of such practices mainly due to resistance to change in construction practices, lack of government incentives, and relevant costs.
In this study, BIM technology is implemented for the architectural design and the energy analysis of a Greek vernacular museum building model. The whole process is then assessed based on the real performance of the building after 25 years of operation. The dynamics of BIM technology to design and analyze complex architectural elements of vernacular buildings, as well as its ability to incorporate tacit knowledge of the vernacular process in building design is critically assessed. In addition, sustainability features observed in the vernacular process followed by the owner of the museum to construct the building are also discussed.
The main aim of this paper is to explore the challenges and opportunities of applying BIM technology to the design and analysis of vernacular buildings. What innovations does BIM technology introduce for the simulation of vernacular buildings? Is there room for improvement for BIM technology, and if so, in which direction? These are the main questions attempted to be answered through the application of BIM technology to the analysis of a vernacular museum building. In that sense, it aspires to elaborate on the potential of BIM to be used as a tool to foster vernacular design processes, instead of being used as an engineering analysis tool solely. By expanding the scope of BIM applications to vernacular buildings, BIM will enable the preservation of sustainable features embedded in vernacular processes.
First, the conceptual background of BIM technology as well as its relation to sustainability are presented. Then, the concept and basic principles of vernacular architecture are discussed. The methodology chapter follows where the research framework is outlined. The next chapter describes the vernacular building process and the BIM-based approach of analyzing the museum building model. In the discussion section, relevant challenges and opportunities observed through the analysis are elaborated. Finally, the conclusionsmsection summarizes the findings of this research.
The term vernacular architecture was coined to describe simple structures composed of indigenous materials [13]. Vernacular architecture utilizes traditional building methods to produce various types of buildings [14]. The plurality of such buildings derives from diverse local identities and construction practices that have evolved over time through experiential knowledge. The term “identity” is a blend of natural (such as climate and topography), human (such as society and community) and cultural factors (such as customs and religion) that defines the traditional building methods in a local context [15].
Vernacular architecture is designed by people who have gained tacit knowledge from observations of the natural environment. Such knowledge relates to technical skills, available materials, construction techniques, and local identity. In an attempt to respond to the rapidly changing reality, vernacular architecture has been evolved through trial and error. Thus, it is believed that vernacular architecture embodies a comprehensive wisdom accumulated in a local context.
Vernacular architecture is a valuable source of reference for bioclimatic design of buildings [16]. The latter aims to provide conditions of thermal and visual comfort, utilizing natural local resources (such as soil, vegetation, and wind). By leveraging the interactions between the building and the natural environment, energy demands for heating, cooling and lighting of the building can be moderated. However, research shows that this way of fostering sustainability may not always be sufficient especially in regions under extreme weather [17].
Natural and economic limitations, such as topography, climate, and availability of building materials in the surrounding area, are imposed on vernacular buildings. In that sense, vernacular architecture is grounded in inherently sustainable rules with the aim to fulfil human needs with respect for the cycle of life [15,18,19].
Vernacular processes can provide useful insights for boosting the sustainability of contemporary building design and construction [20]. Current environmental concerns justify the increasing interest in vernacular architecture studies. Hence, the need to preserve the sustainable principles and values embedded in vernacular buildings has been highlighted in the research community [21,22]. In this context, this study will investigate the potential of BIM to design and analyze vernacular buildings.
During the 1980s, the tendency to switch to electronic drawings became evident. The advent of information and communication technology appeared to have significant economic and social implications [23]. Simultaneously, developments in programming languages led to the emergence of parametric technologies that enabled the digital representation of buildings [24].
Given the complexity of construction projects, the need for the automation of the relevant processes has surfaced. Parametric design tools, such as BIM technology, perceive building elements as dynamic systems, which form a variety of morphological representations subject to transformations. In that sense, entire systems, such as buildings, are composed by multiple interacting subsystems (such as beams, columns, and pipes) with endless possibilities for their functional interrelationships.
As an integrated digital representation of the physical and functional features of a building [25], BIM technology is a tool for automation of the construction process but also a means for specialization that contributes to higher productivity levels of buildings [26]. By gathering all information about a building in a central model, building information management processes are facilitated. The validity of the construction schedule is ensured by monitoring relevant processes [27].
Time-related information is regarded as the fourth dimension in BIM technology, empowering data scheduling and sequential visualization of the buildings’ development. Cost calculations are often considered the fifth dimension in BIM technology, offering the possibility to evaluate buildings from an economic point of view [28]. Building lifecycle data, including operation manuals, maintenance and manufacturer data, can be managed, while sustainability analyses can be performed adding more dimensions to BIM technology [29].
According to Migilinskas et al. [30], the more collaborative a project is, the more benefits from BIM applications arise. The establishment of open standards in BIM applications is expected to catalyze the application of diverse types of analysis and accelerate the adoption of innovations [31]. Data exchange between BIM technologies should be enhanced to develop collaborative environments. Such attempts are made through non-proprietary file formats, such as Green Building XML (gbXML) and Industry Foundation Classes (IFC), which aim to facilitate compatibility between BIM software applications [32]. However, so far they have failed to provide a fully collaborative environment for BIM applications [33].
The concept of sustainable constructions entails the minimization of environmental footprints through the efficient use of natural resources, social cohesion and cost-effective building methods that meet the requirements of users [34]. Conventional design methods have failed to develop sustainable buildings so far mainly due to fragmented data [35]. Given the significant impact of the construction sector on the environment, the need for sustainability integration in buildings has recently attracted attention [36,37].
The assessment of sustainability in buildings during the design phase is hampered by the lack of data and expertise in the field [38]. Further, the complexity of the building sector hinders the development of an internationally comparable inventory for the application of Life Cycle Assessment (LCA) methods [39].
Despite its immature form, BIM technology holds potential for enhancing sustainability over the life cycle of a building [40,41,42]. Sustainability-related factors can be incorporated in BIM software, benefiting the environment, the economy, and society. According to Krygiel and Nies [43], BIM technology can be implemented to improve certain aspects of sustainability in buildings utilizing multidisciplinary data. These aspects include analyses related to building orientation, energy performance, and sustainable materials, which can lead to cost savings and environmental gains.
Considering the collaborative aspect of BIM technology, digital information associated with sustainability can be shared, advancing the field of knowledge commons [44]. In that sense, innovation is fostered, while visualization enables the engagement of non-experts in the design process. Moreover, BIM designs comprise interchangeable modular units that correspond to building components, encouraging reversibility and replacement of components.
Although BIM technology does not provide a comprehensive life-cycle management of environmental sustainability of buildings, it has been applied in the early design and construction [40]. Sustainability analyses can be performed through external evaluation tools and cloud-based technologies. Further, online open inventories can be used to evaluate alternative design options, which correspond to different energy efficiency levels [38]. Such platforms foster collaboration among the participants concerning the decision making processes of construction projects via shared models.
Despite the potential of BIM to promote sustainability, the resistance of relevant parties to change conventional practices and invest in software and skills development prevents the implementation of BIM to its fullest capability. Further, interoperability issues should be addressed to promote collaboration and accurate analyses through BIM technology [40,45]. Notwithstanding such limitations, political measures foster the implementation of BIM technology in constructions, increasing its impact on building design [46].
In order to study highly complex objects, such as vernacular buildings, the BIM ecosystem, and their interrelationship with society, investigations in multi-dimensional ways are needed. For this reason, the case study approach was used since it allows the investigation of phenomena from diverse aspects [47]. Further, it enables an in-depth examination of single cases [48].
The specific case study was selected because it represents a distinctive type of vernacular building that encompasses special architectural features, as described in the following section. This argument was also reinforced via testimonies of the interviewees. By investigating such a complex building from an architectural aspect, it is expected that the challenges and opportunities of BIM technology for designing vernacular buildings will become more evident [49]. Further, most studies on vernacular architecture focus on houses, while recent studies indicate the necessity to investigate public buildings as well [16].
The research framework of this study is illustrated in Figure 1. First, theories on BIM technology were studied, as well as relevant literature on the connection of BIM with sustainability. The concept and principles of vernacular architecture followed. The case of Pavlos Vrellis museum was then analyzed, elaborating on the approach taken by the creator of the museum to construct the building. Web tools, a semi-structured interview with the current owner of the museum and semi-structured interviews with local builders of the wider area took place. The aim of the interview with the owner of the museum was to obtain the required information for the case study, while the interviews with local builders shed light on local building practices and vernacular processes of the wider area. Web tools included data gathered from online platforms, discussion sections and the official website of the museum [50] as well as email communications with BIM experts.
The interview with the current owner of the museum was structured around core questions that attempted to enlighten data regarding the current state of the museum, details about other contributions to its development, the main desires and goals of the creator of the museum, as well as relevant challenges and barriers to progress. The interview took place on site, allowing for observations of the building.
Nine local builders aged between 65 to 90 years old participated in the interviews. Most of the interviewees had previously participated in the construction process of a vernacular building, which was usually their private house or house of a relative of theirs in the wider area of Ioannina city. In terms of their expertise, all of the interviewees were farmers. The questions revolved around their experience of participating in the vernacular process and the entailed challenges and opportunities.
The application of BIM technology followed to illuminate the limitations and advantages of BIM software for the design and analysis of vernacular buildings. In this study, a specific BIM software (i.e., Autodesk Revit Architecture) was implemented for the architectural design of a vernacular museum building model. Further, an energy analysis tool included in the software package (i.e., Revit Conceptual Energy Analysis-CEA) was applied. The results were compared with the actual data of the building’s performance available through testimonies of the owner of the museum. The information needed for the BIM model, including geometrical and topological data, derived from the design drawings of the museum, narratives of the owner, as well as information provided by the creator at the website of the museum.
In line with the principles of vernacular architecture, the buildings of the area under study are made of local stones (usually left unused), wood and mud. Lime, straw, bricks (frequently sun-baked), porcelain, iron, glass and sand are also used. Most of these materials are environmentally friendly since they come from the surrounding environment and they do not require high transport costs or material processing.
Given the steep slopes, rocky terrain and severe winters of the wider region of Epirus (Greece), people had to compromise with constraints set by nature and techno-economic conditions. These techno-economic limitations imposed a certain degree of standardization on the construction of the buildings in terms of available materials and their processing ability. At the same time, the accumulated empirical wisdom and local knowledge exchange practices facilitated the development of inventive building solutions against physical stresses, such as earthquakes and landslides.
Regarding the building structure of the museum, stone walls are thick enough to insulate the building. The windows of the museum are few and small placed at the lower levels of the ground floor for safety reasons. In the upper floor, windows are spacious to ensure ventilation. On the south side, openings are large for storing solar radiation. The rising hot air is concentrated at the roof level and exits through skylights.
Fireplaces and chimneys were some of the most elaborate elements of the museum. Craftsmen composed a variety of decorative volumes and forms in the building structure as an indication of wealth and luxury. Chimneys were used to create a vertical stream of air that contributes to the cooling of the space. On the south side, trees were planted to provide coolness in summer, while in winter they lose leaves, allowing the sun’s rays to enter the building. Further shading was provided by the brown covers of the windows by filtering out the sunlight. In addition, the surrounding vegetation contributed to the shading, sun protection and insulation capacity of the museum.
Pavlos Vrellis, the creator of the museum, was inspired by local traditional mansions. First, he laid out roads and squares that lead visitors to the building. He took special care to harmonize the building with the harsh natural environment. Large rocks and rubble taken from excavations were used to create retaining walls and stabilize steep slopes. The processing of the materials was kept simple, utilizing physical processes and natural resources, such as the sun and water. For example, to make the rocks of the garden look natural, they were left two winters to be washed away from the rain and ice and were wrapped with fine fluid cement.
To grasp the volume of the museum, the creator built maquettes of both the exterior and the interior space. To issue the building permit, local counterparts undertook the planning procedures and the creation of linear drawings. Decisions regarding the building’s orientation, the selection of proper materials, and the structural behavior of the building were taken through knowledge exchange practices.
Considering that the museum counts 25 years of operation, the current owners focus on monitoring and maintaining the building, preserving its original typology and morphology. The first intervention on the building took place in 2008 when significant operational problems appeared on the roof, while specialists were commissioned later to replace some of the windows to reduce energy losses.
The architectural design of the museum building was modeled in Revit Architecture software of Autodesk, while Revit CEA was implemented to convert the architectural design into an energy analytical model. Such a model provides the means for an integrated energy analysis.
The museum consists of two separate buildings: the ground floor, which hosts the library and the laboratory, and an underground space, where the exhibits are placed. Due to the complexity and cave-like shape of the underground space, only the ground floor of the museum space was analyzed. To imprint the underground space, diverse assumptions should be made considering the lack of relevant technological equipment, such as laser scanning.
First, the architectural design of the building was created. After establishing the location of the project, including its longitude and latitude, the creation of “families” took place. This term refers to the ability of an object to have many types within it so that diverse sizes and shapes are created, as the definition of parametric design stipulates. Families were realized directly into the model or were modified separately and employed based on the requirements of each building component. In this way, flexibility was added to the software by personalizing objects of a category according to the relevant needs.
For example, floor projections arose from the composition of different elements that belong to the “wall sweep family”. The protruding volumes of the floor at the corners of the building are supported via wooden components, which create decorative panels. These components were modelled in Revit as structural framing elements.
The museum building retains special local architectural elements that allow for the exploration of the flexibility of BIM technology. Such elements include chimneys and skylights at the central part of the building. Also, extended portions of the roof at the perimeter walls are formed to facilitate the flow of rainwater directly to the ground. Most of these elements resulted from the transformation of existing families that were properly modified to simulate the real building components.
Then, the energy analysis of the building followed. An energy analytical model was created by sorting the building space into discrete spaces. The analysis aimed to forecast energy consumption levels of the building during the stages of the construction and operation. At the same time, it took into account certain characteristics of the building, such as its type, opening hours, and location. The model was also linked to the geospatial world through an online mapping system.
The results of the analysis enable the evaluation of alternative scenarios for energy efficiency. They include carbon dioxide emissions, electricity costs, and energy consumption rates on an annual and a monthly basis (see Appendix). Monthly heating and cooling loads are calculated, indicating the months with the largest demand for heating and cooling (i.e., January and July respectively). Also, graphical representations of wind speed and direction data are offered, facilitating decisions for natural ventilation.
The sensitivity of each building element to the total energy performance of the building is shown in the “Potential Energy Savings/losses” tab. The building elements with the greatest potential for energy savings are listed on top. The bar size indicates the energy-saving potential of the specific element. Therefore, a change in elements with large bar sizes can have a major impact on the energy performance of the building.
Following the results of the CEA, roof insulation has the most significant influence on the energy use of the museum building, as indicated in Figure 5. In particular, a change in the roof insulation element has the potential to contribute to the energy savings of the building by 23% approximately. Wall insulation is another sensitive building element from an energy point of view.
BIM technology can also be used to conduct preliminary assessments for the optimal design and management of buildings. The creation of photorealistic models via online services took place to visually test the integration of the museum building into the surrounding area as depicted in Figure 6. Finally, solar and shading studies can be conducted utilizing representations of the sun path.
The simulation of the museum building in Revit verified certain advantages of BIM technology. Among them, the visualization of the building process, the enhancement of safety levels, and the predictability of future failures are mentioned. Considering the inaccessibility of the area where the museum was built, the use of BIM technology is particularly important for safety reasons. By creating a BIM model, tests and applications can take place within BIM software, saving construction time and effort.
Through BIM technology, the building can be decomposed into elements, which can be analyzed independently. In this way, the researcher can focus on particular elements and perform on-demand simulations. In this case study, the functionality and effectiveness of the energy analysis software were tested based on the current state of the museum building and the interventions implemented therein.
The simulation results of the energy model appeared to be representative of the real situation. By conducting this analysis, cost reductions can potentially be achieved as certain failures that emerged in the building could have been predicted through proper actions and, thus, avoided. However, the exact time when the failures will take place or the way in which specific building components need to be modified to avoid failures could not be specified through this analysis.
Based on this study, the advantages of BIM correlate with the definition of the parameter itself. Given that buildings are dynamic entities confronted with varying conditions, their ability to handle diverse situations is necessary. Although theoretical descriptions of parametric relationships allow for infinite changes in shape and form, parameterized models operate on fixed rules, reducing thousands of objects to minimal parameters. These parameters explicitly describe the internal relationships between building components so that the software can understand relevant functions. This fact limits to some extent the capability to create complex building elements without restrictions imposed by BIM technology [27]. Thus, a kind of standardization in construction emerges due to the parametric nature of BIM technology.
Concerning vernacular design development, each vernacular building is by definition unique and is designed as such. Consequently, there is little standardization in building design of vernacular components and new choices need to be made for each situation. The construction of the architectural and energy model of the museum proved to be a complex and time-consuming process in this study. It was often necessary to depart from the usual modeling in Revit by creating new families that precisely represent the building elements of the museum. Besides, high levels of familiarity with BIM software were required, as well as constant communication with experienced users.
Given the complexity of vernacular buildings, the creation of simulation models is challenging since contradictory factors need to be balanced. On the one hand, the model has to be kept simple enough, so that it can be easily designed and constructed. On the other, it has to be complex enough to be able to represent as accurately as possible the blend of factors that compose a vernacular building.
The initial direction of this research was to create a BIM model of the entire museum. However, due to the sheer complexity of this cave-like space and the lack of technological means, it was impossible to imprint this space. Specifically, there were no semi-automated tools used, such as laser scanning, to provide accurate as-built BIM data of the underground space. Besides, it is typical that the creator built this space by following the geomorphology and terrain of the area without being able to conform to design plans.
Having been developed for modern constructions mainly, BIM software presents limitations when applied to the design of complex elements of vernacular buildings. Besides, building elements of vernacular buildings must be dynamic entities to be able to reflect the evolving cultural, economic, and environmental features of a local society. To this end, the use of open-source templates could facilitate the codification and sharing of parameterized object libraries. The development of building components in a shared database would lead to the integration of the construction industry by creating common communication protocols.
Further, to produce a faithful representation of vernacular buildings, careful consideration must be given to precisely assign the elements of the model to the actual building components. Parametric design through BIM enables adaptability and diversity, facilitating the encoding of the tacit knowledge embodied in vernacular buildings. However, the analysis disregarded the role of environment-related elements, such as local vegetation and soil conditions, for enhancing the building performance. Moreover, the knowledge and building practices used by the creator of the museum could not be incorporated into BIM software (including, for example, the method followed for the processing of the rocks, as described in the previous section).
Hence, it is evident that tacit knowledge cannot be easily captured through BIM. Future research should focus on providing additional smart features to BIM technology. For instance, tacit knowledge could be reflected in the architectural model through the incorporation of local materials and environment-related components (such as foliage cover and vegetation types). Geospatial technologies could play a great role in detecting construction materials in the surrounding area. BIM users could, thus, evaluate alternative options regarding the suitability of materials on a context-specific basis. Finally, by embedding commenting abilities into BIM technology, local building practices could be preserved, opening up the design process to the world and enabling beneficial effects for society.
Sustainable design of buildings is a complex interplay of diverse factors, which include social, technological, economic, and environmental features. Although vernacular architecture seems to be forgotten in contemporary architecture, it arguably provides a worthwhile source of knowledge that fosters environmental, economic and social sustainability. This study explores the challenges and opportunities of using BIM technology for the architectural design and the energy analysis of a vernacular museum building.
Parametric technologies, such as BIM, can adapt to dynamic conditions as the definition of the parameter itself dictates. Given that parameterized models operate on fixed rules, thousands of objects are reduced to a few parameters that describe their interrelationships. This leads to a degree of standardization in construction, which enables the implementation of BIM technology for a well-defined type of building. In that sense, BIM technology could cover a wide range of simulations and analyses needed for this particular building type through an integrated set of applications.
However, in this study, BIM software presented limitations when applied to the design of complex architectural elements of vernacular buildings. Considering the uniqueness of each vernacular building, standardization limits the flexibility needed to design vernacular components. New families were created to accurately represent certain building components of the museum, which was a time-consuming process that required high levels of familiarity with BIM software and constant communication with BIM experts.
Further, due to the complexity of the underground cave-like building and the lack of relevant techno-economic means, the creation of the corresponding BIM model was impossible. Nevertheless, based on the interview with the owner of the museum and the software application, it was concluded that the results of the energy analysis were representative of the real state of the museum. Although the exact time or type of imminent failures could not be determined through the software application, the most sensitive building components from an energy point of view were detected.
Among the limitations of BIM technology, its inability to capture tacit knowledge and adapt to local specificities was discussed. Environment-related elements, including soil properties and local vegetation, as well as local building techniques used for the construction of the vernacular museum could not be incorporated into BIM software. Thus, despite being parametric in nature, BIM lacks the ability to capture and encode tacit knowledge embodied in vernacular buildings in its current form.
Hence, the need to enable the incorporation of commenting abilities into BIM becomes evident to preserve local building practices and facilitate the opening of the design process to the world. A common pool of parameterized data structures could be developed and linked to codified international datasets. Thus, the construction industry could become more integrated since various building components could be described and communicated using a common language.
Future research could also explore the capabilities of BIM to analyze other aspects of manufacturing activities, such as the environmental footprint of building components and the capacity of BIM to be adapted to different local contexts. At the same time, open-source applications in the construction sector are areas of increasing interest that could mitigate problems of conventional design and build practices, such as interoperability and construction standardization.
Further, the addition of smart features and the incorporation of environment-related components into BIM technology should be investigated. The use of geospatial technologies for the detection of locally available construction materials could be considered to facilitate the evaluation of alternative design options on a context-specific basis.
The sustainability features embedded in vernacular design processes are gradually lost. The incorporation of such valuable knowledge in current design processes could provide a flexible way to accommodate human needs, reinforcing the link between society and the environment. Thus, future studies on vernacular buildings should be conducted to enable the preservation of local building techniques in contemporary design practices (for example, by examining the compliance of vernacular buildings with local zoning and building regulations and proposing relevant law reforms).
This paper is part of a project that has received funding from the European Research Council (ERC) under the European Union’s Horizon 2020 research and innovation programme (grant agreement No 802512). The research has also been supported by the European Regional Development Fund.
I am grateful to the following people who provided invaluable comments, guidance, and technical accuracy to the document: Konstantinos Vrellis, Vasilis Kostakis, Vasilis Niaros, Chris Giotitsas, and Athanasios Chassiakos. Any inaccuracies, omissions or misinterpretations are those of the author.
I have no conflict of interest or source of funds for this article.
[1] |
Wong JKW, Kuan KL (2014) Implementing "BEAM Plus" for BIM-based sustainability analysis. Automat Constr 44: 163-175. doi: 10.1016/j.autcon.2014.04.003
![]() |
[2] | Dong YH, Ng ST (2015) A life cycle assessment model for evaluating the environmental impacts of building construction in Hong Kong. Build Environ 89: 183-191. |
[3] | Koeppel S, Urge-Vorsatz D (2007) Assessment of policy instruments for reducing greenhouse gas emissions from buildings, Paris, France, UNEP. |
[4] | Rode P, Burdett R, Soares Gonçalves JC (2011) Buildings: investing in energy and resource efficiency, In UNEP, Towards a green economy: Pathways to sustainable development and poverty eradication. |
[5] |
Mirabella N, Röck M, Saade MRM, et al. (2018) Strategies to improve the energy performance of buildings: A review of their life cycle impact. Build 8: 105-122. doi: 10.3390/buildings8080105
![]() |
[6] |
Priavolou C, Niaros V (2019) Assessing the openness and conviviality of open source technology: The case of the WikiHouse. Sustain 11: 4746. doi: 10.3390/su11174746
![]() |
[7] | Wetter Μ (2011) A view on future building system modeling and simulation, In Hensen J. and R. Lamberts (eds), Building performance simulation for design and operation, Routledge, London. |
[8] |
Faludi J, Lepech MD, Loisos G (2012) Using Life Cycle Assessment methods to guide architectural decision-making for sustainable prefabricated modular buildings. J Green Build 7: 151-170. doi: 10.3992/jgb.7.3.151
![]() |
[9] | Kreider R, Messner J (2013) The uses of BIM: Classifying and selecting BIM uses, Version 0.9, September, The Pennsylvania State University, PA, USA. |
[10] | Kunz J, Gilligan B (2007) VDC/BIM Use in 2007: Significant value, dramatic growth, and apparent business opportunity, Center for Integrated Facility Engineering Technical Report, Stanford University, Stanford, California. |
[11] | Rosenberg T (2006) Building Information Modeling, Roetzel & Andress. Available from http://www.academia.edu/1283324/Building_Information_Modeling. |
[12] | Bernstein P, Pittman J (2004) Barriers to the adoption of Building Information Modeling in the building industry, Autodesk Buildings Solutions, San Rafael, California. |
[13] | Curl JS (2006) A Dictionary of Architecture and Landscape Architecture, Oxford: Oxford University Press. |
[14] | Gruen JP (2017) Vernacular architecture, In Encyclopedia of local history, 3rd eds, Amy H. Wilson, 697-698. |
[15] | Salman M (2018) Sustainability and vernacular architecture: Rethinking what identity is, Urban and architectural heritage conservation within sustainability, Kabila Hmood, IntechOpen. |
[16] |
Nguyena AT, Truong NSH, Rockwood D, et al. (2019) Studies on sustainable features of vernacular architecture in different regions across the world: A comprehensive synthesis and evaluation. Front Archit Res 8:535-548. doi: 10.1016/j.foar.2019.07.006
![]() |
[17] |
Huang Z, Yu M, Zheng L, et al. (2017) One-year Field Study on Indoor Environment of Huizhou Traditional Vernacular Dwellings in China. Proc Eng 205:1316-1322. doi: 10.1016/j.proeng.2017.10.092
![]() |
[18] | McLennan JF (2006) The philosophy of sustainable design, Kansas City, MO: EcoTone. |
[19] | Erarslan A (2019) A contemporary interpretation of vernacular architecture. The architecture of Nail Çakirhan, Turkey. Build Environ 7:5-25. |
[20] |
Dayaratne R (2018) Toward sustainable development: lessons from vernacular settlements of Sri Lanka. Front Archit Res 7:334-346. doi: 10.1016/j.foar.2018.04.002
![]() |
[21] |
Kamarudin Z (2015) Long-roofed houses of northeastern peninsular Malaysia: sustainability of its identity in the built environment. Proc Environ Sci 28:698-707. doi: 10.1016/j.proenv.2015.07.082
![]() |
[22] |
Barbero-Barrera MM, Gil-Crespo IJ, Maldonado-Ramos L (2014) Historical development and environment adaptation of the traditional cave-dwellings in Tajuña's valley, Madrid, Spain. Build Environ 82:536-545. doi: 10.1016/j.buildenv.2014.09.023
![]() |
[23] | Dutton W, Peltu M (1996) Information and communication technologies: Visions and realities. Oxford: Oxford University Press. |
[24] | Björk BC (1995) Requirements and information structures for building product data models, PhD Dissertation, Helsinki University of Technology, Espoo Finland. |
[25] | National Institute of building sciences, About the national BIM standard-United States, 2019. Available from https://www.nationalbimstandard.org/about. |
[26] |
Turk Ž (2016) Ten questions concerning Building Information Modelling. Build Environ 107: 274-284. doi: 10.1016/j.buildenv.2016.08.001
![]() |
[27] |
Ciribini A, Mastrolembo Ventura S, Paneroni M (2016) Implementation of an interoperable process to optimise design and construction phases of a residential building: a BIM pilot project. Automat Constr 71: 62-73. doi: 10.1016/j.autcon.2016.03.005
![]() |
[28] | Lahdou R, Zetterman D (2011) BIM for project managers, Department of Civil and Environmental Engineering, Division of Construction Management, Chalmers University of Technology, Göteborg. |
[29] |
Carvalho JP, Bragança L, Mateus R (2019) Optimising building sustainability assessment using BIM. Automat Constr 102:170-182. doi: 10.1016/j.autcon.2019.02.021
![]() |
[30] |
Migilinskas D, Popov V, Juocevicius V, et al. (2013) The benefits, obstacles and problems of practical BIM implementation. Proc Eng 57: 767-774. doi: 10.1016/j.proeng.2013.04.097
![]() |
[31] | Bui N, Merschbrock C, Munkvold, BE, et al. (2019) The role of an innovation community in supporting BIM deployment - The case of buildingSMART Norway. Proceedings of the 3rd International Conference on Building Information Modelling (BIM) in Design, Construction and Operations, Seville, Spain. |
[32] | Laakso M, Kiviniemi A (2012) The IFC Standard-A review of history, development, and standardization. Electr J ITcon 17: 134-161. |
[33] |
Oh M, Lee J, Wan Hong S et al. (2015) Integrated system for BIM-based collaborative design. Automat Constr 58: 196-206. doi: 10.1016/j.autcon.2015.07.015
![]() |
[34] | Lee D (2008) Identifying sustainability priorities and engaging stakeholders-The Hong Kong housing authority's challenges and experience, Hong Kong Housing Authority, Hong Kong. |
[35] | Eastman C, Teicholz P, Sacks R, et al. (2011) BIM handbook: A guide to Building Information Modeling for owners, managers, designers, engineers, and contractors. John Wiley & Sons, Hoboken. |
[36] | Heywood H (2019) 101 rules of thumb for sustainable buildings and cities, 1st eds, London: RIBA Publishing, 224 pages. |
[37] |
Wong K, Fan Q (2013) Building information modelling (BIM) for sustainable building design. Facilit 31: 138-157. doi: 10.1108/02632771311299412
![]() |
[38] |
Gervásio H, Santos P, Martins R, et al. (2014) A macro-component approach for the assessment of building sustainability in early stages of design. Build Environ 73: 256-270. doi: 10.1016/j.buildenv.2013.12.015
![]() |
[39] |
Khasreen M, Banfill PF, Menzies G (2009) Life-cycle assessment and the environmental impact of buildings: A review. Sustain 1: 1-28. doi: 10.3390/su1010001
![]() |
[40] |
Wong JKW, Zhou J (2015) Enhancing environmental sustainability over building life cycles through green BIM: A review. Automat Constr 57: 156-165. doi: 10.1016/j.autcon.2015.06.003
![]() |
[41] |
Oti AH, Tizani W (2015) BIM extension for the sustainability appraisal of conceptual steel design. Adv Eng Inform 29: 28-46. doi: 10.1016/j.aei.2014.09.001
![]() |
[42] |
Azhar S, Carlton WA, Olsen D, et al. (2011) Building information modeling for sustainable design and LEED® rating analysis. Automat Constr 20: 217-224. doi: 10.1016/j.autcon.2010.09.019
![]() |
[43] | Krygiel E, Nies B (2008) Green BIM: Successful sustainable design with Building Information Modeling, Hoboken, NJ: Wiley. |
[44] | Garmulewicz A (2015) 3D printing in the commons: knowledge and the nature of digital and physical resources. Doctoral thesis. University of Oxford. |
[45] | Moon HJ, Choi MS, Kim SK, et al. (2011) Case studies for the evaluation of interoperability between a BIM based architectural model and building performance analysis programs. In Proceedings of the 12th Conference of International Building Performance Simulation Association. |
[46] |
Miettinen R, Paavola S (2014) Beyond the BIM utopia: Approaches to the development and implementation of Building Information Modeling. Automat Constr 43: 84-91. doi: 10.1016/j.autcon.2014.03.009
![]() |
[47] | Yin RK (2002) Case study research design and methods, Thousand Oaks, CA: Sage. |
[48] |
Verner JM, Abdullah LM (2012) Exploratory case study research: Outsourced project failure. Inform Soft Tech 54: 866-886. doi: 10.1016/j.infsof.2011.11.001
![]() |
[49] |
Flyvbjerg B (2006) Five misunderstandings about case-study research. Qual Inq 12: 219-245. doi: 10.1177/1077800405284363
![]() |
[50] | Pavlos Vrellis Museum of Greek history of wax effigies, Prologue-Introduction, 2019. Available from https://www.vrellis.gr/ep/. |
![]() |
![]() |
1. | Christina Priavolou, Nikiforos Tsiouris, Vasilis Niaros, Vasilis Kostakis, Towards Sustainable Construction Practices: How to Reinvigorate Vernacular Buildings in the Digital Era?, 2021, 11, 2075-5309, 297, 10.3390/buildings11070297 | |
2. | Ying Hong, Ahmed W.A. Hammad, Ali Akbar Nezhad, Optimising the implementation of BIM: A 2-stage stochastic programming approach, 2022, 136, 09265805, 104170, 10.1016/j.autcon.2022.104170 | |
3. | Surya S., Binumol Tom, 2022, Using BIM for Residential Projects in Kerala-Challenges and Possibilities, 978-1-6654-6792-6, 1, 10.1109/ICNGIS54955.2022.10079874 | |
4. | Nuray Benli Yıldız, Zehra Kalpaklı, Nuray Özkaraca, Creation of Historical Building Information Modelling (HBIM) Library, A Case Study of Registered House (No:56), Akçakoca, 2024, 12, 2148-2446, 901, 10.29130/dubited.1241508 | |
5. | Nathalia Fonseca Arenas, Muhammad Shafique, Recent progress on BIM-based sustainable buildings: State of the art review, 2023, 15, 26661659, 100176, 10.1016/j.dibe.2023.100176 | |
6. | Asimina Kouvara, Christina Priavolou, Denise Ott, Philipp Scherer, Verena Helen van Zyl-Bulitta, Circular, Local, Open: A Recipe for Sustainable Building Construction, 2023, 13, 2075-5309, 2493, 10.3390/buildings13102493 |