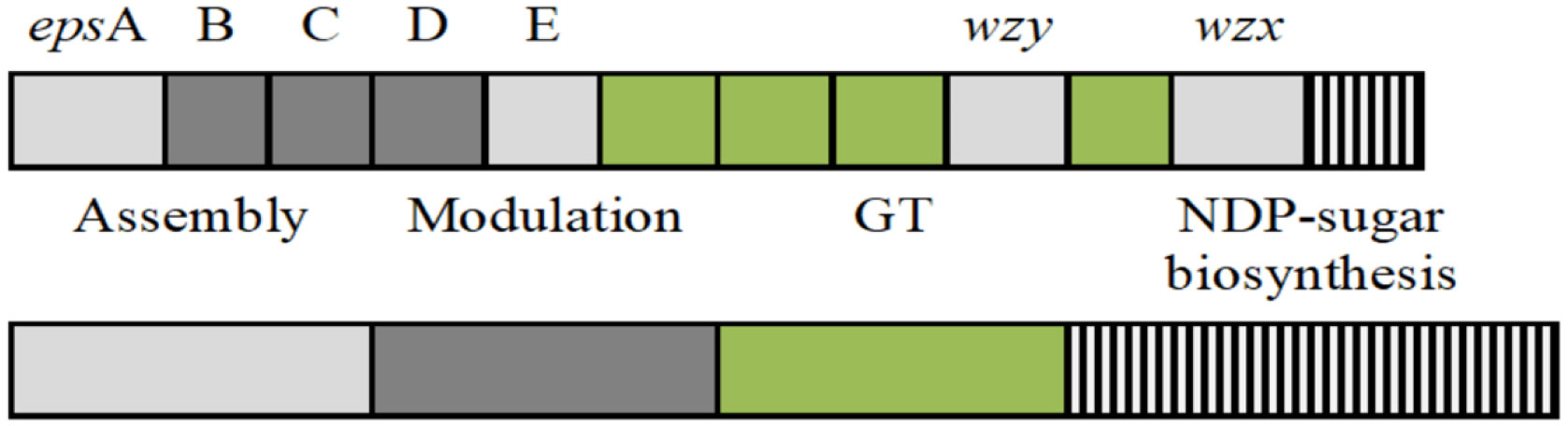
Citation: Phu-Tho Nguyen, Tho-Thi Nguyen, Duc-Cuong Bui, Phuoc-Toan Hong, Quoc-Khanh Hoang, Huu-Thanh Nguyen. Exopolysaccharide production by lactic acid bacteria: the manipulation of environmental stresses for industrial applications[J]. AIMS Microbiology, 2020, 6(4): 451-469. doi: 10.3934/microbiol.2020027
[1] | Jhuma Biswas, Amal K. Paul . Optimization of factors influencing exopolysaccharide production by Halomonas xianhensis SUR308 under batch culture. AIMS Microbiology, 2017, 3(3): 564-579. doi: 10.3934/microbiol.2017.3.564 |
[2] | Thomas Trunk, Hawzeen S. Khalil, Jack C. Leo . Bacterial autoaggregation. AIMS Microbiology, 2018, 4(1): 140-164. doi: 10.3934/microbiol.2018.1.140 |
[3] | Thomas Bintsis . Lactic acid bacteria as starter cultures: An update in their metabolism and genetics. AIMS Microbiology, 2018, 4(4): 665-684. doi: 10.3934/microbiol.2018.4.665 |
[4] | Hafidh Shofwan Maajid, Nurliyani Nurliyani, Widodo Widodo . Exopolysaccharide production in fermented milk using Lactobacillus casei strains AP and AG. AIMS Microbiology, 2022, 8(2): 138-152. doi: 10.3934/microbiol.2022012 |
[5] | Sugenendran Supramani, Rahayu Ahmad, Zul Ilham, Mohamad Suffian Mohamad Annuar, Anita Klaus, Wan Abd Al Qadr Imad Wan-Mohtar . Optimisation of biomass, exopolysaccharide and intracellular polysaccharide production from the mycelium of an identified Ganoderma lucidum strain QRS 5120 using response surface methodology. AIMS Microbiology, 2019, 5(1): 19-38. doi: 10.3934/microbiol.2019.1.19 |
[6] | Nur Raihan Abdullah, Faez Sharif, Nur Hafizah Azizan, Ismail Fitri Mohd Hafidz, Sugenendran Supramani, Siti Rokhiyah Ahmad Usuldin, Rahayu Ahmad, Wan Abd Al Qadr Imad Wan-Mohtar . Pellet diameter of Ganoderma lucidum in a repeated-batch fermentation for the trio total production of biomass-exopolysaccharide-endopolysaccharide and its anti-oral cancer beta-glucan response. AIMS Microbiology, 2020, 6(4): 379-400. doi: 10.3934/microbiol.2020023 |
[7] | Patrick Di Martino . Extracellular polymeric substances, a key element in understanding biofilm phenotype. AIMS Microbiology, 2018, 4(2): 274-288. doi: 10.3934/microbiol.2018.2.274 |
[8] | Marzena Połaska, Barbara Sokołowska . Bacteriophages—a new hope or a huge problem in the food industry. AIMS Microbiology, 2019, 5(4): 324-346. doi: 10.3934/microbiol.2019.4.324 |
[9] | Esther Menendez, Paula Garcia-Fraile . Plant probiotic bacteria: solutions to feed the world. AIMS Microbiology, 2017, 3(3): 502-524. doi: 10.3934/microbiol.2017.3.502 |
[10] | Hisako Nakagawa, Tadaaki Miyazaki . Beneficial effects of antioxidative lactic acid bacteria. AIMS Microbiology, 2017, 3(1): 1-7. doi: 10.3934/microbiol.2017.1.1 |
In recent years, the trend of using natural polymers in many fields has led to the development of research on producing exopolysaccharides (EPSs) from bacteria. The unique structural features have made bacterial EPSs of particular interest in the fields of chemistry, medicine and food industry [1]. Because of their ability to increases hold water, EPSs are widely used as viscous, stabilizing and emulsifying agents in the food industry [2] to improve the rheological property, texture and sensibility of bread and fermented milk products such as yogurt and cheese [3]. In addition to its technological properties, EPSs also have potential health benefits as antioxidant, anticancer, anti-inflammatory antiviral activities [4],[5] and cholesterol lowering effects [6].
Among EPS producing bacteria, Lactic acid bacteria (LAB) have has grasped the attention of researchers thank to their strong ability to produce EPSs. The LAB strains as Streptococcus, Lactococcus, Pediococcus, Lactobacillus, Leuconostoc and Weissellale are often used to produce EPSs [7]. LAB are recognized as safe microorganisms (GRAS-Generally Recognized As Safe) and also capable of creating EPSs with many different structures without any health risks [8]. In LAB, EPSs play an important role in controlling cell surface physicochemical characteristics [9], protecting bacterial cells from dehydration, negative environmental impacts, antibiotics, phagocytosis, and phage attacks [10]–[12]. EPSs take part in the structural components of extracellular matrix, in which cells are encapsulated during the development of cell membrane [13].
Previously, there have been several reviews to describe the stress response in LAB [14]–[16] focusing almost exclusively on the function of stress proteins (HS proteins, Csp, etc.) and their regulators (HrcA, CtsR) or that of proteins linked physically to the cell membrane (transport systems, sensors, housekeeping proteases, etc.). However, in order to understand clearly the role of stresses in EPS biosynthesis, a series of key questions must be addressed:
-Why are EPSs related to stress resistance?
-What type of stress to apply?
-Is it possible to control EPS biosynthesis using environmental stress?
Therefore, in this review, we will discuss EPS synthesis; the physiological functions of EPSs as well as the impact of environmental stresses on EPS production and the expression of genes involved in EPS biosynthesis in LAB. This assessment will clarify the relationship between environmental stresses and changes in LAB EPS synthesis. It also suggests that environmental stress can improve the productivity of EPSs from LAB and produce customized EPSs with desired functionality.
LAB synthesize two types of EPSs including homopolysaccharides and heteropolysaccharides [17]. Homopolysaccharide synthesis is a relatively simple biochemical process involving a specific GT (glucansucrase or fructansucrase) and an extracellular sugar donor (sucrose for the synthesis of glucans, but it can also be other fructose-containing oligosaccharide (e.g. raffinose) for the synthesis of fructans) [18],[19]. Heteropolysaccharide synthesis is a complex process which involves the specific role of several gene products (enzymes) encoded by the eps gene cluster and housekeeping genes. These gene products can be categorized into four groups (or modules) basing on their functions: polysaccharide assembly machinery (the priming glycosyltranferases, Wzx or flippase, Wzy or polymerase and EpsA), phosphoregulatory system managing polysaccharide assembly (EpsB, EpsC and EpsD), glycosyltranferases and sugar nucleotide biosynthetic pathways. Genes encoding acetyl- and pyruvyl transferase involved in the chemical decoration of EPSs also present in the cluster (Figure 1) [20].
In general, EPS biosynthesis is summarized in 3 main steps (Figure 2). Firstly, it is the generation of activated sugar precursors (or sugar nucleotides such as uridine diphosphate glucose and thymidine diphosphate glucose) for repeating units. The sugar nucleotides are synthesized in multistep pathways from glycolytic intermediates, generally glucose-6-phosphate or fructose-6-phosphate. This complex process requires the function of several housekeeping gene products such as phosphoglucomutase (converts glucose-6-phosphate to glucose-1-phosphate) [21]; UDP-glucose pyrophosphorylase and dTDP-glucose pyrophosphorylase (converts glucose-1-phosphate to sugar nucleotides UDP-glucose and dTDP-glucose, respectively) [22]. The producing potential of different sugar nucleotides is intrinsically determined by the gene content of each LAB, which ultimately dictates the type of monomers found in EPSs. EPSs produced by LAB consist of repeating units which is usually composed of two or more (usually 3–8) types of monosaccharides [22]–[24].
Secondly, the synthesis process of repeating units begins by attaching the first sugar nucleotide to the isoprenoid lipid carrier, undecaprenyl phosphate, which is attached to the cytoplasmic membrane of the cell, and being catalyzed by priming glycosyltransferase. This is followed by the sequential addition of sugar nucleotides to form repeating units and glycosyltransferases encoded by gense in the eps gene clusters catalyze for this process [25]. Finally, it is the polymerization and export of repeating units from the inner part to the outer part of cell membrane. Basically, three different proteins which are also encoded in the eps gene cluster carry out polymerization and export process: A flippase (encoded by wzx or cpsJ) or a translocase moves the lipid carrier-repeating unit complex from the inner surface of cytoplasm membrane to periplasmic. Then, a polymerase (encoded by wzy or cpsH) catalyzes the coupling of repeating units [21]. Lastly, a chain length determination protein separates lipid carrier-repeating unit complex to stop polymerization and export process simultaneously determines the chain length of final EPSs [21].
EPSs are synthesized to serve various functions in the bacteria. One of these is to ensure bacteria survive under stress conditions. The function of EPSs in LAB's stress resistance is discussed in the next section.
EPSs are the important structural component of LAB cell wall [26]. EPSs form a layer surrounding cells to protect them against adverse environmental conditions such as dehydration, extreme temperature, acid, osmotic stress, phagocytosis, macrophages, and antibiotics [25],[27],[28]. Other roles of EPSs include biofilm formation, cell adhesion mechanisms [29] and the determinant of strain-specific characteristics in host interaction [30].
To adapt to environmental stresses, LAB can alter their cell surface by producing more EPSs [31]. The increased production of EPSs results in thicker and firmer cell walls (Figure 3). As a result, it increases the LAB's resistance to stresses. This feature may be useful to exploit for improving the stamina of the probiotic starters as well as the ability to produce EPSs in LAB. Numerous studies have also demonstrated that, after being pre-stressed, LAB's viability is improved significantly [31],[32].
As discussed, LAB enhance EPSs synthesis to create a physical barrier on the cell surface which separates the cell from stress. Especially, in low pH conditions, this EPS layer restricts the access of exogenous acids to bacterial cells due to the anions bound to EPSs as phosphate groups [33]. Phosphate residues confer a net negative charge to EPSs [27]. The presence of phosphate in EPSs is also observed in many studies [34]–[37]. According to these viewpoints, LAB may produce anionic EPSs carrying phosphate groups under acid stress conditions and they cause negative charge on cell surface to prevent proton diffusion into cells (Figure 4).
In the case of osmotic stress, a sudden increase in osmotic pressure made by stress results in water movement from the inside to the outside of cell, causing a detrimental loss of cell turgor pressure and changing intracellular solute concentration, which ultimately can seriously affect cell viability [38]. In response to osmotic stress, LAB synthesize EPSs to protect themselves by holding water around cells to prevent dehydration (Figure 4) [39]. The water holding capacity of EPSs is due to the presence of OH groups in their structure. Another substance such as glycerol known for high water holding capacity can sometimes be included in the structure of EPSs. The presence of glycerol was recorded in EPSs produced by Latilactobacillus sakei [34]. Furthermore, external protective compounds such as water stress proteins which aid in the survival of cells from desiccation can accumulate in extracellular glycan and show homologies with carbohydrate-modifying enzymes [40].
A lot of bacteria respond to carbon dioxide stress by producing EPSs to create a barrier for slowing the diffusion of toxic substance into cells, which in this case would be carbon dioxide [41],[42]. Similarly, EPSs also provides support for LAB to resist metal stress. The negatively charged groups in EPSs bind cations and protect bacterial cells against toxic metals [43]. In addition, it has been shown that EPSs are related to oxidative stress resistance in LAB. The supplementation of EPSs into culture medium could appreciate the growth of L. mesenteroides by 10 times under oxidative stress and influence promoting the aerobic growth of oxygen-sensitive strains such as Lactobacillus and Bifidobacterium [44]. In oxidative stress conditions, the production of harmful reactive oxygen species may be increased. EPSs can scavenge of these reactive oxygen species to prevent cell damage (Figure 4) [45]. Furthermore, EPSs also reduce oxidative stress by extrusion of dissolved oxygen from aqueous culture medium [44].
The ability to protect cells from environmental stresses depends on EPS-phenotype. Terms such as ‘ropy’, ‘mucoid’, and ‘slime’ have been used to describe the different EPS producing phenotypes of LAB [46]. LAB strains with the ropy-exopolysaccharide production show better resistance to stress. According to a report, the ropy phenotype of Lactiplantibacillus plantarum is related to better tolerance to low pH [29].
Together with the cell protection function, the positive advantages of EPSs are highlighted through the essential contribution to the human health such as prebiotic, anticoagulant, antioxidant, anti-inflammatory, antiviral, cholesterol lowering effects and even anticancer activity [47].
LAB's EPSs have been showed an essential functional role in blood coagulation prevention. The strong anticoagulant activity of EPSs in sulphate derivatives has been demonstrated. Heparin Cofactor II is a potent inhibitor of thrombin in the coagulation pathway and the sulphated EPSs provides an acidic medium condittion to facilitate the inhibitory effect of Heparin Cofactor II on thrombin [48],[49]. The sulphated sites and stereochemistry of EPSs activate HC II according to the allosteric mechanism [50]. One study proved that EPS47FE and EPS68FE which are secreted by L. plantarum 47FE and Lactiplantibacillus pentosus 68FE, respectively, exhibit strong anticoagulant and fibrinolytic activity [51].
Prebiotic effects were also observed at LAB's EPSs [52],[53]. EPSs from LAB can be used by probiotic strains [54] and have the capacity to stimulate the growth of probiotic bacteria and maintain the balance of intestinal microflora [55],[56]. The prebiotic potential of LAB's EPSs has demonstrated in many studies. The α-D-glucan synthesized by L. plantarum can stimulate probiotic bacteria growth. It is low-digested by artificial gastric juice and show to put non-probiotic bacteria off growing that Enterobacteriaceae is a representative instance [57]. In vitro EPSs produced by Weissella cibaria, Weissella confusa, L. plantarum and Pediococcus pentosaceus could be used as a prebiotic ingredient in the food industry to modulate gut microbiota towards health benefits [58].
Another health promoting functions of EPSs produced by LAB are cholesterol lowering effects [50]. In an in vitro assay, EPSs produced by L. plantarum BR2 show cholesterol lowering properties (45%) [59]. Based on animal and in vitro experiments, several hypotheses to explain the cholesterol lowering mechanism of EPSs have been proposed including bile removal, anabolism and cholesterol conversion, co-precipitation effects, etc...[60],[61].
Free radicals usually cause serious health problems. Therefore, EPSs are such an important natural antioxidant to prevent the free radicals. LAB's EPSs also exhibit high antioxidant activity. One evidence showed that EPSs from Lactobacillus gasseri FR4 have a good free radical activity, while hydroxyl and superoxide radical capture activities are dependent on EPS concentration [62]. Additionally, under in-vivo conditions, LAB'S EPSs have been shown to increase the activity of hepatic superoxide effutase, serum catalase, and glutathione S-transferase simutaneously reducing serum malondialdehydes and monoamine oxide activity. These are excellent antioxidant and anti-aging evidence created by EPSs [63],[64].
In recent decades, the immunomodulatory potential of EPSs has received a lots of scientific consideration. Many in-vitro studies have demontrated that EPSs produced by different LAB species have the immunomodulatory ability [17]. The phosphate group (a good inducer of the immune response) plays a critical role and characterizes the immunomodulatory effects of EPSs. Phosphate molecules can activate various immune cells (such as macro-phages and lymphocytes) and initiate immune responses [65]. According to these results, it can be speculated that EPS generated under acid stress (it seems that acidic EPSs [65]) may exhibit stronger immunological properties.
Cancer is one of the health problems getting a lot of attention today and it is usually treated via chemotherapy method. However, chemotherapy can cause some unexpected effects which can range from minor to severe and life-threatening [66]. Therefore, other pharmaceutical products are being researched to help cure cancer that LAB's EPSs are the helpful ones due to its anti-tumor effects [65]. EPSs from L. plantarum 70810 can significantly inhibit the proliferation of tumor cells such as HepG-2, BGC-823, especially HT-29 [67]. In vitro evaluation of anticancer properties of Lactobacillus acidophilus EPSs in colon cancer cell lines demontrated that they were able to inhibit the expression of genes involved in angiogenesis and tumor survival [68]. In another study, EPSs from Levilactobacillus brevis MSR10 are used to synthesize the silver nanoparticles (AgNPs). According to the results obtained, these AgNPs not only had high antimicrobial and antioxidant capabilities but also significantly reduced the percentage of live HT-29 cells [69].
Many recent researches have been conducted to show the antiviral bioavailability of EPSs and they are considered to be an immune stimulant affect in a number of ways in the immune system, contributing to the protection of human cells against certain viruses [65]. A study has proven that EPSs extracted from L. plantarum LRCC5310 were able to resist human rotavirus in vitro [70].
Under environmental stresses, LAB have different adaptation mechanisms which involve the accumulation of compatible solutes and energy storage compounds; regulation of energy production pathways, as well as the modulation of cell envelope, i.e., membrane, cell wall, surface layers, and EPSs [71]. In this review, we divide environmental stresses into common groups including nutrient factors (carbon sources, nitrogen source, carbon dioxide, oxigen, mineral salts, etc.); physiological factors (pH, osmotic stress, temperature, etc.) and co-cultivation to discuss the impact of stresses on EPS production in LAB.
The composition of nutrients is one of the factors which affects the growth and metabolism of cells [72]. Thus, EPS synthesis is also influenced by culture medium compositions [73]. The starvation or oversupply of nutrients such as nitrogen, sugars, carbon dioxide, etc. may change EPS synthesis [74]–[76]. Effects of nutritional stress on EPS synthesis in LAB have been proved by prior studies. Marshall et al. demonstrated that EPS production in Lactobacillus lactis subsp. cremoris LC 330 is stimulated by nitrogen limitation [77]. In contrast, Lactobacillus delbrueckii ssp. bulgaricus was recorded increased EPS production in additional nitrogen-enriched [78].
Excessive sugar presence in the culture medium also increases EPS production in LAB. The possible explanations for the increased EPS synthesis under stress of high sugar concentration are osmosis, unlimited supply of sugar building blocks and high energy availability [75]. The increased sucrose concentration in the MRS medium was suitable for EPS overproduction in Lactobacillus confusus TISTR 1498 [79]. It has also been showed that Lactobacillus strains (L. delbrueckii bulgaricus, Lactobacillus helveticus and Lacticaseibacillus casei) yield the highest EPSs when growing on fermentation medium comprising 20% sucrose as carbon source [80]. Likewise, the synthesis of EPSs in Fructilactobacillus sanfranciscensis LTH2590 rose by increasing the sucrose concentration in the medium and reached about 40 g/L at sucrose concentration of 160 g/L [81]. In the case of Leuconostoc mesenteroides NRRL B-1299, culture medium with sucrose concentration over 5 g/L caused more dextran production [82]. Similar to succorse, the high concentration of glucose is also advantageous for the production of EPSs. As previously reports, the EPS production of Streptococcus thermophilus (W22) and L. delbrueckii subsp. bulgaricus (B3, G12) was stimulated by high glucose concentration [83]. It was also shown that the presence of excess sugar in medium has a improving effect on EPS production in L. casei and Lacticaseibacillus rhamnosus, although the growth is apparently decreased [84],[85].
In some LAB strains, carbon dioxide can used as a carbon source for growth because it is a substrate in carbamoyl phosphate synthesis and other metabolic reactions in LAB [86]. Carbon dioxide regulates physiology and energy metabolism by regulating enzymes involved in glycolysis [87]. The impact of carbon dioxide stress on EPS production in LAB has also recorded in several studies. EPS production depended entirely on carbon dioxide concentration and the maximum EPS yield, produced by Bifidobacterium longum JBL05, increased proportionally to carbon dioxide concentration in the range of 0–20% [88]. L. casei growing in carbon dioxide-rich environment was surrounded by a membrane like EPS component [89]. In contrast to carbon dioxide stress, under dissolved oxygen concentration above 0.05 ppm, B. longum declined growth and EPS accumulation [90]. These results suggest that the EPS synthesis of B. longum varies under different stress conditions. Although oxidative stress reduces the accumulation of EPSs in B. longum, it increases EPS production in B. scardovii and B. adolescentis. One evidence has shown an increase in EPS production and the cell surface hydrophobicity of B. scardovii and B. adolescentis under oxidative stress [91].
EPS production in LAB may be stimulated by various physical stresses as a cellular defense response, which could also enhance the formation of biofilms [92]. The rate of EPSs in biofilms can account for about 50–90% of total organic matter amount [93],[94] and EPSs, together with proteins, nucleic acids and lipids, form the structure of a biofilm matrix [95]. Low pH was found to significantly decrease the formation of biofilm in L. rhamnosus GG, while it enhanced biofilm formation in Limosilactobacillus reuteri strains [96],[97]. Although studies have not focused on the effect of low pH stress on EPS production in LAB bacteria, an increase in EPS production under low pH has been observed in several reports. The EPS production of Lactobacillus helveticus ATCC 15807 under controlled pH of 6.2 was lower than that observed at pH 4.5 [98]. Likewise, EPS production in Ligilactobacillus salivarius UCO_979C-2, adapted variant strain, after 24 h at pH 2.6 was 690 mg/L, compared to native L. salivarius UCO_979C-1 strain that was only 450 mg/L at pH 6.4 [99].
The negative effects of osmotic stress on cells may be limited because of the presence of EPSs. Therefore, the presence of substances caused high osmotic pressures, such as NaCl, can stimulate EPS synthesis on the cell wall. As previously described by Seesuriyachan et al., the EPS synthesis of L. confusus TISTR 1498 did not depend on biomass and stress of high NaCl concentration could enhance EPS production in solid state fermentation [79]. Similarly, Leuconostoc mesenteroides/pseudomesenteroides 406 achieved maximum EPS yield in the presence of 5% NaCl [100]. In contrast, the inhibition of EPS production by NaCl was recorded in L. helveticus ATCC 15807 [98].
Excessive temperature causes protein denaturation, nucleic acid and membrane damage [101]. However, when bacteria are exposed to extreme temperature, they reprogram their metabolism to deal with temperature changes [102]. One of the metabolic changes is an increase in EPS synthesis. High temperature stress is also recorded to affect EPS production in LAB. Nguyen et al. demonstrated that sub-lethal thermal stress increases EPS production and improves the viability of B. bifidum [31].
In biotechnology, co-culture has been shown to make microorganisms more resistant to environmental changes and can perform more complex metabolic activities through the culture combination of various strains [103],[104]. Consequently, co-culture can also affect EPS synthesis. The effect of co-cultivation on improving EPS production of LAB is often studied in combination with Saccharomyces cerevisiae. Lactobacillus kefiranofaciens JCM 6985 enhanced the production of kefiran, an exopolysaccharide, in co-culture with S. cerevisiae IFO 0216 [105]. The EPS production of L. rhamnosus strains was also increased by 39–42% and a higher level of EPS operon expression was observed for L. rhamnosus RW-9595M in co-culture [106]. Similarly, L. paracasei co-cultured with Saccharomyces cerevisiae resulted in the overexpression of gene (coding for polyprenyl glycosylphosphotransferase) involved in EPS production [107]. In facts, the enhancement of EPS production by LAB in co-culture with Saccharomyces cerevisiae is induced by direct and physical contact with components on the surface of yeast cell [105]. In a high viscosity environment, LAB can be stressed by themselves own acids. LAB adhesion to yeast cell will activate EPS production in LAB because this adhesion leads to efficient lactic acid consumption by yeast cells [105].
In general, the biosynthesis of EPS can be altered either up or down under different stress conditions. These changes may be related to expression level of genes involved in EPS synthesis. To clarify this hypothesis, we have discussed the expression of esp genes under environmental stresses. Details are presented in section 6.
Bacteria respond to stresses by activating various regulatory mechanisms including activities involved in metabolisms, cell envelope and gene expression, giving them the potentiality to adapt to extreme environmental conditions (Figure 5) [108]. Changes in gene expression establish the principal component of the bacterial response [109] and can alter the biosynthesis of EPSs under stress conditions [110].
The correlation between stress and the expression of genes involved in EPS biosynthesis has been documented in LAB. Increasing expression of gtf01207 gene, encoding for a priming glycosyltransferase related to EPS synthesis, was observed in B. animalis subsp. Lactis after exposure to stress of acid, bile salts and osmosis [92],[111]. According to another study, when the pH of culture medium decreased from pH 6.5 to pH 5.5, the expression level of epsNMLKJ genes in Streptococus thermophilus ASCC 1275 increased. However, the expression of genes involved in the synthesis of sugar nucleotides such as dTDP-rhamnose and UDP-GlcNAc reduced [112]. Also in this study, when temperature increases from 37 °C to 40 °C, there are not changes in the expression level of epsNMLKJ cluster, but the expression of eps1C and eps1D genes increase while that of eps2C and eps2D decrease [112]. Expression of gtf gene encoding for enzyme which produces beta-glucan (membrane-linked glycosyltransferase enzyme) in Lacticaseibacillus paracasei caused 60 times higher heat tolerance, 20 times higher acid tolerance compare to control strain [113]. Evaluation of gene expression is not only based on mARN but also on genetic products which are enzymes formed after decoding. Glyceraldehyd-3-phosphate dehydrogenase, proved to be necessary for EPS production of Xanthomonas campestris pv. [81], increased heterological expression in L. rhamnosus HN001 during heat stress. In contrast, the heterological expression levels of glyceraldehyd-3-phosphate dehydrogenase and phosphoglycerate kinase, related to EPS biosynthesis of Xanthomonas axonopodis pv. Glycines) [114], decreased under osmotic stress [115]. In general, environmental stress can alter the expression of genes involved in EPS biosynthesis. The result of this response may increase EPS production in LAB.
In order to survive under environmental stress conditions, LAB react by synthesizing EPSs to form a protective barrier around the cells. This EPS synthesis is catalyzed by enzymes encoded by genes in the eps cluster and the impact of environmental stresses can alter the expression of these genes resulting in increased EPS production. Accordingly, environmental stress may be considered as a major factor to control LAB's EPS biosynthesis. The impact of different stresses on EPS synthesis is summarized in Table 1. In general, the synthesis of EPSs in LAB under stress conditions depends on the type of stress and bacterial species. Within the same species, EPS production may not be the same under different stress conditions. For instance, EPS production in L. helveticus ATCC 15807 is stimulated by stress at low pH but inhibited by sodium chloride stress. Conversely, a specific stress may stimulate EPS production in one species but inhibit it in another (Table 1).
Stress exposure | Strains | Effect on EPS production: Inhibition (-) Stimulation (+) | References |
Low pH | L. rhamnosus GG | − | Lebeer et al., 2007 [97] |
L. reuteri | + | Slížová et al., 2015 [98] | |
L. helveticus ATCC 15807 | + | Torino et al., 2005 [99] | |
L. salivarius UCO_979C-2 | + | Sanhueza et al., 2015 [100] | |
High temperature | B. bifidum | + | Nguyen et al., 2014 [31] |
Sodium chloride | L. helveticus ATCC 15807 | − | Torino et al., (2005) [99] |
Leuconostoc mesenteroides/pseudomesenteroides 406 | + | Silvia-Simona GROSU-TUDOR, 2014 [101] | |
L. confusus TISTR 1498 | + | Seesuriyachan, 2012 [80] | |
Carbon dioxide | B. longum JBL05 | + | Ninomiya et al., 2009 [89] |
L. casei | + | Santillan et al., 2015 [90] | |
Oxidation | B. longum | − | Golowczyc et al., 2011 [91] |
B. scardovii; B. adolescentis | + | Qian, Borowski, & Calhoon, 2011 [92] | |
Excessive nitrogen source | L. delbrueckii ssp. bulgaricus | + | García-Garibay & Marshall, 2008 [78] |
Excessive carbon source | L. confusus TISTR 1498 | + | Seesuriyachan et al., 2012 [80] |
L. (delbrueckii bulgaricus, helveticus and casei) | + | Hussein et al., 2015 [81] | |
F. sanfranciscensis LTH2590 | + | Korakli, Pavlovic, & Vogel, 2003 [82] | |
Leuconostoc mesenteroides NRRL B-1299 | + | Dols, Remaud-Simeon, & Monsan, 1997 [83] | |
S. thermophilus W22 and L. delbrueckii subsp. bulgaricus (B3, G12) | + | Yuksekdag & Aslim, 2008 [84] | |
L. Casei CG11 | + | Cerning et al., 1994 [85] | |
L. rhamnosus C83 | + | Gamar, Blondeau, & Simonet, 2003 [86] | |
Co-cultivation | L. kefiranofaciens JCM 6985 | + | Tada et al., 2007 [106] |
L. rhamnosus (ATCC 9595, R0011, and RW-9595M) | + | Bertsch, Roy, & LaPointe, 2019 [107] | |
L. paracasei ATCC 334 | + | Yamasaki-Yashiki, Sawada, Kino-oka, & Katakura, 2016 [108] |
The EPSs produced by LAB can be the key ingredients showing promising functional roles for various utilities in food, medicine, etc. However, low EPS productivity could be a problem limiting commercial applications of these EPSs. Currently, EPS production improvement studies often focus on optimizing culture mediums, using genetic engineering, using cheap fermentation substrates, and environmental stress [116]. As discussed, EPSs protect LAB from negative environmental effects. Consequently, environmental stresses can promote EPS synthesis in LAB. This feature can be useful to exploit to improve the stamina of probiotic starters and the yield of EPSs.
In addition, the biological activities of EPSs such as prebiotic, anti-oxidant, anti-inflammatory, ... are related to the monosaccharide compositions of EPSs. It has been proved that EPSs with distinct monosaccharide compositions vary in their therapeutic effects [117]. For instance, the proportion of monosaccharides (galactose > rhamnose > glucose) in the composition of EPSs produced by L. reuteri Mh-001 was demonstrated to relate to their anti-inflammatory activity, in particular galactose content enhances EPS anti-inflammatory effects on the macrophages [118]. Similarly, rhamnose-containing EPSs have been used in cosmetic applications because of owning to their emulsifying activity [119]. For further studies, we believe that environmental stresses may be an effective method which positively alters EPS biosynthesis to generate a new EPS type with higher biological activity for industrial applications.
[1] |
Paul P, Ramraj SK, Neelakandan Y, et al. (2011) Production and purification of a novel exopolysaccharide from lactic acid bacterium Streptococcus phocae PI80 and its functional characteristics activity in vitro. Bioresour Technol 102: 4827-4833. doi: 10.1016/j.biortech.2010.12.118
![]() |
[2] |
Singh P, Saini P (2017) Food and health potentials of exopolysaccharides derived from Lactobacilli. Microbiol Res J Int 22: 1-14. doi: 10.9734/MRJI/2017/36935
![]() |
[3] |
Garai-Ibabe G, Dueñas M, Irastorza A, et al. (2010) Naturally occurring 2-substituted (1,3)-β-D-glucan producing Lactobacillus suebicus and Pediococcus parvulus strains with potential utility in the production of functional foods. Bioresour Technol 101: 9254-9263. doi: 10.1016/j.biortech.2010.07.050
![]() |
[4] |
Liu C, Lu J, Lu L, et al. (2010) Isolation, structural characterization and immunological activity of an exopolysaccharide produced by Bacillus licheniformis 8-37-0-1. Bioresour Technol 101: 5528-5533. doi: 10.1016/j.biortech.2010.01.151
![]() |
[5] |
Pan D, Mei X (2010) Antioxidant activity of an exopolysaccharide purified from Lactococcus lactis subsp. lactis 12. Carbohydr Polym 80: 908-914. doi: 10.1016/j.carbpol.2010.01.005
![]() |
[6] |
Nakajima H, Suzuki Y, Hirota T (2006) Cholesterol lowering activity of ropy fermented milk. J Food Sci 57: 1327-1329. doi: 10.1111/j.1365-2621.1992.tb06848.x
![]() |
[7] | Patel A, Prajapati J (2013) Food and health applications of exopolysaccharides produced by Lactic acid Bacteria. Adv Dairy Res 1: 1-7. |
[8] |
Surayot U, Wang J, Seesuriyachan P, et al. (2014) Exopolysaccharides from lactic acid bacteria: Structural analysis, molecular weight effect on immunomodulation. Int J Biol Macromol 68: 223-240. doi: 10.1016/j.ijbiomac.2014.05.005
![]() |
[9] |
Polak-Berecka M, Waśko A, Paduch R, et al. (2014) The effect of cell surface components on adhesion ability of Lactobacillus rhamnosus. Antonie van Leeuwenhoek 106: 751-762. doi: 10.1007/s10482-014-0245-x
![]() |
[10] |
Badel-Berchoux S, Bernardi T, Michaud P (2011) New perspective for Lactobacilli exopolysaccharides. Biotechnol Adv 29: 54-66. doi: 10.1016/j.biotechadv.2010.08.011
![]() |
[11] |
Lebeer S, Vanderleyden J, De Keersmaecker SCJ (2008) Genes and molecules of Lactobacilli Supporting Probiotic Action. Microbiol Mol Biol Rev 72: 728-764. doi: 10.1128/MMBR.00017-08
![]() |
[12] |
Gauri DS, Mandal SM, Mondal K, et al. (2009) Enhanced production and partial characterization of an extracellular polysaccharide from newly isolated Azotobacter sp. SSB81. Bioresour Technol 100: 4240-4243. doi: 10.1016/j.biortech.2009.03.064
![]() |
[13] |
Marvasi M, Visscher P, Casillas-Martínez L (2010) Exopolymeric substances (EPS) from Bacillus subtilis: Polymers and genes encoding their synthesis. FEMS Microbiol Lett 313: 1-9. doi: 10.1111/j.1574-6968.2010.02085.x
![]() |
[14] |
Guchte M, Serror P, Chervaux C, et al. (2002) Stress response in lactic acid bacteria. Antonie van Leeuwenhoek 82: 187-216. doi: 10.1023/A:1020631532202
![]() |
[15] |
Papadimitriou K, Alegría Á, Bron PA, et al. (2016) Stress physiology of lactic acid bacteria. Microbiol Mol Biol Rev 80: 837-890. doi: 10.1128/MMBR.00076-15
![]() |
[16] | Serrazanetti DI, Gottardi D, Montanari C, et al. (2013) Dynamic stresses of lactic acid bacteria associated to fermentation processes. Lactic Acid Bacteria–R & D for Food, Health and Livestock Purposes Rijeka: InTech, 539-570. |
[17] |
Riaz Rajoka MS, Wu Y, Mehwish HM, et al. (2020) Lactobacillus exopolysaccharides: New perspectives on engineering strategies, physiochemical functions, and immunomodulatory effects on host health. Trends Food Sci Technol 103: 36-48. doi: 10.1016/j.tifs.2020.06.003
![]() |
[18] |
van Hijum SAFT, Kralj S, Ozimek LK, et al. (2006) Structure-function relationships of glucansucrase and fructansucrase enzymes from lactic acid bacteria. Microbiol Mol Biol Rev 70: 157-176. doi: 10.1128/MMBR.70.1.157-176.2006
![]() |
[19] |
Galle S, Arendt E (2014) Exopolysaccharides from sourdough lactic acid bacteria. Crit Rev Food Sci Nutr 54: 891-901. doi: 10.1080/10408398.2011.617474
![]() |
[20] |
Zeidan AA, Poulsen VK, Janzen T, et al. (2017) Polysaccharide production by lactic acid bacteria: from genes to industrial applications. FEMS Microbiol Rev 41: S168-S200. doi: 10.1093/femsre/fux017
![]() |
[21] |
Laws A, Gu Y, Marshall V (2001) Biosynthesis, characterisation, and design of bacterial exopolysaccharides from lactic acid bacteria. Biotechnol Adv 19: 597-625. doi: 10.1016/S0734-9750(01)00084-2
![]() |
[22] |
Welman A, Maddox I (2003) Exopolysaccharides from lactic acid bacteria: Perspectives and challenges. Trends Biotechnol 21: 269-274. doi: 10.1016/S0167-7799(03)00107-0
![]() |
[23] | Ruas-Madiedo P, Salazar N, de los Reyes-Gavilán CG (2009) Biosynthesis and chemical composition of exopolysaccharides produced by lactic acid bacteria. Bacterial Polysaccharides: Current Innovations and Future Trends Norwich: Caister Academic Press, 279-310. |
[24] | Neves AR, Pool W, Kok J, et al. (2005) Overview on sugar metabolism and its control in–The input from in vivo NMR. FEMS Microbiol Rev 29: 531-554. |
[25] |
De Vuyst L, Degeest B (1999) Heteropolysaccharides from lactic acid bacteria. FEMS Microbiol Rev 23: 153-177. doi: 10.1016/S0168-6445(98)00042-4
![]() |
[26] |
Kleerebezem M, Hols P, Bernard E, et al. (2010) The extracellular biology of the Lactobacilli. FEMS Microbiol Rev 34: 199-230. doi: 10.1111/j.1574-6976.2009.00208.x
![]() |
[27] |
Looijesteijn PJ, Trapet L, Vries Ed, et al. (2001) Physiological function of exopolysaccharides produced by Lactococcus lactis. Int J Food Microbiol 64: 71-80. doi: 10.1016/S0168-1605(00)00437-2
![]() |
[28] |
Ruas-Madiedo P, Hugenholtz J, Zoon P (2002) An overview of the functionality of exopolysaccharides produced by lactic acid bacteria. Int Dairy J 12: 163-171. doi: 10.1016/S0958-6946(01)00160-1
![]() |
[29] |
Caggianiello G, Kleerebezem M, Spano G (2016) Exopolysaccharides produced by lactic acid bacteria: from health-promoting benefits to stress tolerance mechanisms. Appl Microbiol Biotechnol 100: 3877-3886. doi: 10.1007/s00253-016-7471-2
![]() |
[30] | Lee IC, Caggianiello G, Swam I, et al. (2016) Strain-specific features of extracellular polysaccharides and their impact on host interactions of Lactobacillus plantarum. Appl Environ Microbiol 82: AEM.00306-00316. |
[31] |
Huu Thanh N, Razafindralambo H, Blecker C, et al. (2014) Stochastic exposure to sub-lethal high temperature enhances exopolysaccharides (EPS) excretion and improves Bifidobacterium bifidum cell survival to freeze-drying. Biochem Eng J 88: 85-94. doi: 10.1016/j.bej.2014.04.005
![]() |
[32] |
Desmond C, Stanton C, Fitzgerald GF, et al. (2002) Environmental adaptation of probiotic lactobacilli towards improvement of performance during spray drying. Int Dairy J 12: 183-190. doi: 10.1016/S0958-6946(02)00040-7
![]() |
[33] |
Donoghue H, Newman H (1976) Effect of glucose and sucrose on survival in batch culture of Streptococcus mutans C67-1 and a noncariogenic mutant, C67-25. Infect Immun 13: 16-21. doi: 10.1128/IAI.13.1.16-21.1976
![]() |
[34] |
Robijn G, Berg D, Haas H, et al. (1995) Determination of the structure of the exopolysaccharide produced by Lactobacillus sake 0–1. Carbohydr Res 276: 117-136. doi: 10.1016/0008-6215(95)00172-P
![]() |
[35] |
Robijn GW, Wienk HLJ, van den Berg DJC, et al. (1996) Structural studies of the exopolysaccharide produced by Lactobacillus paracasei 34-1. Carbohydr Res 285: 129-139. doi: 10.1016/S0008-6215(96)90178-0
![]() |
[36] |
Kitazawa H, Ishii Y, Uemura J, et al. (2000) Augmentation of macrophage functions by an extracellular phosphopolysaccharide from Lactobacillus delbrueckii ssp. bulgaricus. Food Microbiol 17: 109-118. doi: 10.1006/fmic.1999.0294
![]() |
[37] |
Tallon R, Bressollier P, Urdaci MC (2003) Isolation and characterization of two exopolysaccharides produced by Lactobacillus plantarum EP56. Res Microbiol 154: 705-712. doi: 10.1016/j.resmic.2003.09.006
![]() |
[38] |
Waśko A, Polak-Berecka M, Gustaw W (2013) Increased viability of probiotic Lactobacillus rhamnosus after osmotic stress. Acta Aliment 42: 520-528. doi: 10.1556/AAlim.42.2013.4.7
![]() |
[39] |
Morales O, López-Cortés A, Hernandez-Duque G, et al. (2001) Extracellular polymers of microbial communities colonizing limestone surfaces. Methods Enzymol 336: 331-339. doi: 10.1016/S0076-6879(01)36599-0
![]() |
[40] | Hill DR, Hladun Sl Fau-Scherer S, Scherer S Fau-Potts M, et al. (1994) Water stress proteins of Nostoc commune (Cyanobacteria) are secreted with UV-A/B-absorbing pigments and associate with 1,4-beta-D-xylanxylanohydrolase activity. J Biol Chem 269: 7726-7734. |
[41] |
Cotter P, Hill C (2003) Surviving the acid test: responses of gram-positive bacteria to low pH. Microbiol Mol Biol Rev 67: 429-453. doi: 10.1128/MMBR.67.3.429-453.2003
![]() |
[42] |
Chowdhury R, Sahu G, Das J (1996) Stress response in pathogenic bacteria. J Biosci 21: 149-160. doi: 10.1007/BF02703105
![]() |
[43] |
Ordax M, Marco-Noales E, López MM, et al. (2010) Exopolysaccharides favor the survival of Erwinia amylovora under copper stress through different strategies. Res Microbiol 161: 549-555. doi: 10.1016/j.resmic.2010.05.003
![]() |
[44] |
Yan M, Wang B-H, Xu X, et al. (2018) Extrusion of dissolved oxygen by exopolysaccharide from Leuconostoc mesenteroides and its implications in relief of the oxygen stress. Front Microbiol 9: 2467-2467. doi: 10.3389/fmicb.2018.02467
![]() |
[45] |
Zhang L, Liu C, Li D, et al. (2013) Antioxidant activity of an exopolysaccharide isolated from Lactobacillus plantarum C88. Int J Biol Macromol 54: 270-275. doi: 10.1016/j.ijbiomac.2012.12.037
![]() |
[46] |
Ruas-Madiedo P, de los Reyes-Gavilán CG (2005) Invited review: methods for the screening, isolation, and characterization of exopolysaccharides produced by lactic acid bacteria. J Dairy Sci 88: 843-856. doi: 10.3168/jds.S0022-0302(05)72750-8
![]() |
[47] | Vurmaz M, Sahin E, Dertli E (2019) Potential Health Promoting Functions of Exopolysaccharides (EPS) from Lactic Acid Bacteria (LAB). 3rd International Conference on Advanced Engineering Technologies Bayburt: Bayburt University, 1379-1382. |
[48] |
Li H, Mao W, Hou Y, et al. (2012) Preparation, structure and anticoagulant activity of a low molecular weight fraction produced by mild acid hydrolysis of sulfated rhamnan from Monostroma latissimum. Bioresour Technol 114: 414-418. doi: 10.1016/j.biortech.2012.03.025
![]() |
[49] | Li N, Liu X, He XX, et al. (2016) Structure and anticoagulant property of a sulfated polysaccharide isolated from the green seaweed Monostroma angicava. Carbohydr Polym 159. |
[50] |
Zhou Y, Cui Y, Qu X (2019) Exopolysaccharides of lactic acid bacteria: Structure, bioactivity and associations: A review. Carbohydr Polym 207: 317-332. doi: 10.1016/j.carbpol.2018.11.093
![]() |
[51] |
Abo Saif FAA, Sakr EAE (2020) Characterization and bioactivities of exopolysaccharide produced from probiotic Lactobacillus plantarum 47FE and Lactobacillus pentosus 68FE. Bioact Carbohydr Diet Fibre 24: 100231. doi: 10.1016/j.bcdf.2020.100231
![]() |
[52] |
Bello FD, Walter J, Hertel C, et al. (2001) In vitro study of prebiotic properties of levan-type exopolysaccharides from lactobacilli and non-digestible carbohydrates using denaturing gradient gel electrophoresis. Syst Appl Microbiol 24: 232-237. doi: 10.1078/0723-2020-00033
![]() |
[53] | O'Connor EB, Barrett E, Fitzgerald G, et al. (2006) Production of vitamins, exopolysaccharides and bacteriocins by probiotic bacteria. Probiotic Dairy Products Hoboken: John Wiley & Sons Inc., 359-388. |
[54] |
Tsuda H, Miyamoto T (2010) Production of Exopolysaccharide by Lactobacillus plantarum and the Prebiotic Activity of the Exopolysaccharide. Food Sci Technol Res 16: 87-92. doi: 10.3136/fstr.16.87
![]() |
[55] | Balzaretti S, Taverniti V, Guglielmetti S, et al. (2016) A novel rhamnose-rich hetero-exopolysaccharide isolated from Lactobacillus paracasei DG activates THP-1 human monocytic cells. Appl Environ Microbiol 83: e02702-02716. |
[56] | Bengoa A, Llamas-Arriba M, Iraporda C, et al. (2017) Impact of growth temperature on exopolysaccharide production and probiotic properties of Lactobacillus paracasei strains isolated from kefir grains. Food Microbiol 69. |
[57] |
Das D, Baruah R, Goyal A (2014) A food additive with prebiotic properties of an alpha-D-glucan from Lactobacillus plantarum DM5. Int J Biol Macromol 69: 20-26. doi: 10.1016/j.ijbiomac.2014.05.029
![]() |
[58] |
Hongpattarakere T, Cherntong N, Wichienchot S, et al. (2012) In vitro prebiotic evaluation of exopolysaccharides produced by marine isolated lactic acid bacteria. Carbohydr Polym 87: 846-852. doi: 10.1016/j.carbpol.2011.08.085
![]() |
[59] |
Sasikumar K, Vaikkath D, Devendra L, et al. (2017) An exopolysaccharide (EPS) from a Lactobacillus plantarum BR2 with potential benefits for making functional foods. Bioresour Technol 241. doi: 10.1016/j.biortech.2017.05.075
![]() |
[60] |
Ishimwe N, Daliri E, Lee B, et al. (2015) The perspective on cholesterol lowering mechanisms of probiotics. Mol Nutr Food Res 59. doi: 10.1002/mnfr.201400548
![]() |
[61] |
Michael D, Davies T, Moss J, et al. (2017) The anti-cholesterolaemic effect of a consortium of probiotics: An acute study in C57BL/6J mice. Sci Rep 7: 2883. doi: 10.1038/s41598-017-02889-5
![]() |
[62] |
Rani RP, Anandharaj M, David Ravindran A (2018) Characterization of a novel exopolysaccharide produced by Lactobacillus gasseri FR4 and demonstration of its in vitro biological properties. Int J Biol Macromol 109: 772-783. doi: 10.1016/j.ijbiomac.2017.11.062
![]() |
[63] |
Pan D, Mei X (2010) Antioxidant activity of an exopolysaccharide purified from Lactococcus lactis subsp. lactis 12. Carbohydr Polym 80: 908-914. doi: 10.1016/j.carbpol.2010.01.005
![]() |
[64] |
Guo Y, Pan D, Li H, et al. (2013) Antioxidant and immunomodulatory activity of selenium exopolysaccharide produced by Lactococcus lactis subsp. lactis. Food Chem 138: 84-89. doi: 10.1016/j.foodchem.2012.10.029
![]() |
[65] |
Saadat YR, Khosroushahi AY, Gargari BP (2019) A comprehensive review of anticancer, immunomodulatory and health beneficial effects of the lactic acid bacteria exopolysaccharides. Carbohydr Polym 217: 79-89. doi: 10.1016/j.carbpol.2019.04.025
![]() |
[66] |
Nurgali K, Jagoe RT, Abalo R (2018) Editorial: adverse effects of cancer chemotherapy: anything new to improve tolerance and reduce sequelae? Front Pharmacol 9: 245. doi: 10.3389/fphar.2018.00245
![]() |
[67] |
Wang K, Li W, Rui X, et al. (2014) Characterization of a novel exopolysaccharide with antitumor activity from Lactobacillus plantarum 70810. Int J Biol Macromol 63: 133-139. doi: 10.1016/j.ijbiomac.2013.10.036
![]() |
[68] |
Deepak V, Ramachandran S, Balahmar RM, et al. (2016) In vitro evaluation of anticancer properties of exopolysaccharides from Lactobacillus acidophilus in colon cancer cell lines. In Vitro Cell Dev Biol Anim 52: 163-173. doi: 10.1007/s11626-015-9970-3
![]() |
[69] |
Riaz Rajoka MS, Mehwish HM, Zhang H, et al. (2020) Antibacterial and antioxidant activity of exopolysaccharide mediated silver nanoparticle synthesized by Lactobacillus brevis isolated from Chinese koumiss. Colloids Surf B 186: 110734. doi: 10.1016/j.colsurfb.2019.110734
![]() |
[70] |
Kim K, Lee G, Thanh HD, et al. (2018) Exopolysaccharide from Lactobacillus plantarum LRCC5310 offers protection against rotavirus-induced diarrhea and regulates inflammatory response. J Dairy Sci 101: 5702-5712. doi: 10.3168/jds.2017-14151
![]() |
[71] |
Gaucher F, Bonnassie S, Rabah H, et al. (2019) Review: adaptation of beneficial propionibacteria, lactobacilli, and bifidobacteria improves tolerance toward technological and digestive stresses. Front Microbiol 10: 841. doi: 10.3389/fmicb.2019.00841
![]() |
[72] |
Phillips KN, Godwin CM, Cotner JB (2017) The effects of nutrient imbalances and temperature on the biomass stoichiometry of freshwater bacteria. Front Microbiol 8: 1692. doi: 10.3389/fmicb.2017.01692
![]() |
[73] | Adebayo-Tayo BC, Onilude AO (2008) Comparative influence of medium composition on biomassgrowth, lactic acid and exopolysaccharides production bysome strains of lactic acid bacteria. The Internet J Microbiol 7. |
[74] |
Mbye M, Baig MA, AbuQamar SF, et al. (2020) Updates on understanding of probiotic lactic acid bacteria responses to environmental stresses and highlights on proteomic analyses. Compr Rev Food Sci Food Saf 19: 1110-1124. doi: 10.1111/1541-4337.12554
![]() |
[75] |
Cirrincione S, Breuer Y, Mangiapane E, et al. (2018) ‘Ropy’ phenotype, exopolysaccharides and metabolism: Study on food isolated potential probiotics LAB. Microbiol Res 214: 137-145. doi: 10.1016/j.micres.2018.07.004
![]() |
[76] |
Ninomiya K, Matsuda K, Kawahata T, et al. (2009) Effect of CO2 concentration on the growth and exopolysaccharide production of Bifidobacterium longum cultivated under anaerobic conditions. J Biosci Bioeng 107: 535-537. doi: 10.1016/j.jbiosc.2008.12.015
![]() |
[77] |
Marshall VM, Cowie EN, Moreton RS (2009) Analysis and production of two exopolysaccharides from Lactococcus lactis subsp. cremoris LC330. J Dairy Res 62: 621-628. doi: 10.1017/S0022029900031356
![]() |
[78] | García-Garibay M, Marshall V (2008) Polymer production by Lactobacillus delbrueckii ssp. J Appl Microbiol 70: 325-328. |
[79] |
Seesuriyachan P, Kuntiya A, Hanmoungjai P, et al. (2012) Optimization of exopolysaccharide overproduction by Lactobacillus confusus in solid state fermentation under high salinity stress. Biosci Biotechnol Biochem 76: 912-917. doi: 10.1271/bbb.110905
![]() |
[80] |
Hussein M-D, Ghaly M, Osman M, et al. (2015) Production and prebiotic activity of exopolysaccharides derived from some probiotics. Egypt Pharm J 14: 1-9. doi: 10.4103/1687-4315.154687
![]() |
[81] |
Korakli M, Pavlovic M, Vogel R (2003) Exopolysaccharide and kestose production by Lactobacillus sanfranciscensis LTH2590. Appl Environ Microbiol 69: 2073-2079. doi: 10.1128/AEM.69.4.2073-2079.2003
![]() |
[82] |
Dols M, Remaud-Simeon M, Monsan PF (1997) Dextransucrase production by Leuconostoc mesenteroides NRRL B-1299. Comparison with L. mesenteroides NRRL B-512F. Enzyme Microb Technol 20: 523-530. doi: 10.1016/S0141-0229(96)00189-5
![]() |
[83] |
Yuksekdag ZN, Aslim B (2008) Influence of different carbon sources on exopolysaccharide production by Lactobacillus delbrueckii subsp. bulgaricus (B3, G12) and Streptococcus thermophilus (W22). Braz Arch Biol Technol 51: 581-585. doi: 10.1590/S1516-89132008000300019
![]() |
[84] |
Cerning J, Renard C, Thibault J, et al. (1994) Carbon source requirements for exopolysaccharide production by Lactobacillus casei CG11 and partial structure analysis of the polymer. Appl Environ Microbiol 60: 3914-3919. doi: 10.1128/AEM.60.11.3914-3919.1994
![]() |
[85] |
Gamar L, Blondeau K, Simonet JM (2003) Physiological approach to extracellular polysaccharide production by Lactobacillus rhamnosus strain C83. J Appl Microbiol 83: 281-287. doi: 10.1046/j.1365-2672.1997.00228.x
![]() |
[86] | Arsène-Ploetze F, Bringel F (2004) Role of inorganic carbon in lactic acid bacteria metabolism.84: 49-59. |
[87] |
Arioli S, Roncada P, Salzano AM, et al. (2009) The relevance of carbon dioxide metabolism in Streptococcus thermophilus. Microbiology 155: 1953-1965. doi: 10.1099/mic.0.024737-0
![]() |
[88] |
Ninomiya K, Matsuda K, Kawahata T, et al. (2009) Effect of CO2 concentration on the growth and exopolysaccharide production of Bifidobacterium longum cultivated under anaerobic conditions. J Biosci Bioeng 107: 535-537. doi: 10.1016/j.jbiosc.2008.12.015
![]() |
[89] | Santillan EF, Shanahan T, Omelon C, et al. (2015) Isolation and characterization of a CO2-tolerant Lactobacillus strain from Crystal Geyser, Utah, U.S.A. Front Earth Sci 3: 41. |
[90] |
Golowczyc M, Silva J, Teixeira P, et al. (2011) Cellular injuries of spray-dried Lactobacillus spp. isolated from kefir and their impact on probiotic properties. Int J Food Microbiol 144: 556-560. doi: 10.1016/j.ijfoodmicro.2010.11.005
![]() |
[91] |
Qian Y, Borowski WJ, Calhoon WD (2011) Intracellular granule formation in response to oxidative stress in Bifidobacterium. Int J Food Microbiol 145: 320-325. doi: 10.1016/j.ijfoodmicro.2010.11.026
![]() |
[92] |
Amund D, Ouoba L, Sutherland J, et al. (2014) Assessing the effects of exposure to environmental stress on some functional properties of Bifidobacterium animalis ssp. lactis. Benefi Microbes 5: 1-9. doi: 10.3920/BM2013.0099
![]() |
[93] |
Donlan R (2002) Biofilms: Microbial Life on Surfaces. Emerging Infect Dis 8: 881-890. doi: 10.3201/eid0809.020063
![]() |
[94] |
Flemming H-C, Wingender J (2001) Relevance of microbial extracellular polymeric substances (EPSs) - Part I: Structural and ecological aspects. Water Sci Technol 43: 1-8. doi: 10.2166/wst.2001.0326
![]() |
[95] | Ciszek-Lenda M (2011) Biological functions of exopolysaccharides from probiotic bacteria. Cent Eur J Immun 36: 51-55. |
[96] |
Lebeer S, Verhoeven T, Velez M, et al. (2007) Impact of environmental and genetic factors on biofilm formation by the probiotic strain Lactobacillus rhamnosus GG. Appl Environ Microbiol 73: 6768-6775. doi: 10.1128/AEM.01393-07
![]() |
[97] |
Slížová M, Nemcova R, Madar M, et al. (2015) Analysis of biofilm formation by intestinal lactobacilli. Can J Microbiol 61: 1-10. doi: 10.1139/cjm-2015-0007
![]() |
[98] |
Torino MI, Hébert EM, Mozzi F, et al. (2005) Growth and exopolysaccharide production by Lactobacillus helveticus ATCC 15807 in an adenine-supplemented chemically defined medium. J Appl Microbiol 99: 1123-1129. doi: 10.1111/j.1365-2672.2005.02701.x
![]() |
[99] |
Sanhueza Carrera E, Paredes-Osses E, González C, et al. (2015) Effect of pH in the survival of Lactobacillus salivarius strain UCO_979C wild type and the pH acid acclimated variant. Electron J Biotechnol 18: 343-346. doi: 10.1016/j.ejbt.2015.06.005
![]() |
[100] | Grosu-Tudor SS, Zamfir M (2014) Exopolysaccharide production by selected lactic acid bacteria isolated from fermented vegetables. Sci Bulletin Series F Biotechnol 18: 107-114. |
[101] |
Ananta E, Volkert M, Knorr D (2005) Cellular injuries and storage stability of spray-dried Lactobacillus rhamnosus GG. Int Dairy J 15: 399-409. doi: 10.1016/j.idairyj.2004.08.004
![]() |
[102] |
Nguyen HT, Truong DH, Kouhounde S, et al. (2016) Biochemical engineering approaches for increasing viability and functionality of probiotic bacteria. Int J Mol Sci 17. doi: 10.3390/ijms17060867
![]() |
[103] |
Bader J, Mast-Gerlach E, Popović M, et al. (2010) Relevance of microbial coculture fermentations in biotechnology. J Appl Microbiol 109: 371-387. doi: 10.1111/j.1365-2672.2009.04659.x
![]() |
[104] | Smid E, Lacroix C (2012) Microbe-microbe interactions in mixed culture food fermentations. Curr Opin Biotechnol 24. |
[105] |
Tada S, Katakura Y, Ninomiya K, et al. (2007) Fed-Batch coculture of Lactobacillus kefiranofaciens with Saccharomyces cerevisiae for effective production of kefiran. J Biosci Bioeng 103: 557-562. doi: 10.1263/jbb.103.557
![]() |
[106] |
Bertsch A, Roy D, LaPointe G (2019) Enhanced exopolysaccharide production by Lactobacillus rhamnosus in co-culture with Saccharomyces cerevisiae. Appl Sci 9: 4026. doi: 10.3390/app9194026
![]() |
[107] | Yamasaki-Yashiki S, Sawada H, Kino-oka M, et al. (2016) Analysis of gene expression profiles of Lactobacillus Paracasei induced by direct contact with Saccharomyces Cerevisiae through recognition of yeast mannan. Biosci Microbiota Food Health 36. |
[108] | Zhang X, Li Z, Pang S, et al. (2020) The impact of cell structure, metabolism and group behavior for the survival of bacteria under stress conditions. Arch Microbiol . |
[109] |
Jin J, Zhang B, Guo H, et al. (2012) Mechanism analysis of acid tolerance response of Bifidobacterium longum subsp. longum BBMN 68 by gene expression profile using RNA-sequencing. PLOS One 7: e50777. doi: 10.1371/journal.pone.0050777
![]() |
[110] |
Rasulov BA, Dai J, Pattaeva MA, et al. (2020) Gene expression abundance dictated exopolysaccharide modification in Rhizobium radiobacter SZ4S7S14 as the cell's response to salt stress. Int J Biol Macromol 164: 4339-4347. doi: 10.1016/j.ijbiomac.2020.09.038
![]() |
[111] |
Ruas-Madiedo P, Gueimonde M, Arigoni F, et al. (2009) Bile affects the synthesis of exopolysaccharides by Bifidobacterium animalis. Appl Environ Microbiol 75: 1204-1207. doi: 10.1128/AEM.00908-08
![]() |
[112] |
Wu Q, Shah NP (2018) Comparative mRNA-Seq analysis reveals the improved EPS production machinery in Streptococcus thermophilus ASCC 1275 during optimized milk fermentation. Front Microbiol 9: 445. doi: 10.3389/fmicb.2018.00445
![]() |
[113] |
Stack H, Kearney N, Stanton C, et al. (2010) Association of beta-glucan endogenous production with increased stress tolerance of intestinal lactobacilli. Appl Environ Microbiol 76: 500-507. doi: 10.1128/AEM.01524-09
![]() |
[114] |
Guo W, Gao J, Wang HJ, et al. (2020) Phosphoglycerate kinase is involved in carbohydrate utilization, extracellular polysaccharide biosynthesis, and cell motility of Xanthomonas axonopodis pv. glycines independent of Clp. Front Microbiol 11: 91. doi: 10.3389/fmicb.2020.00091
![]() |
[115] |
Prasad J, McJarrow P, Gopal P (2003) Heat and osmotic stress responses of probiotic Lactobacillus rhamnosus HN001 (DR20) in relation to viability after drying. Appl Environ Microbiol 69: 917-925. doi: 10.1128/AEM.69.2.917-925.2003
![]() |
[116] |
Xu Y, Cui Y, Yue F, et al. (2019) Exopolysaccharides produced by lactic acid bacteria and Bifidobacteria: Structures, physiochemical functions and applications in the food industry. Food Hydrocolloids 94: 475-499. doi: 10.1016/j.foodhyd.2019.03.032
![]() |
[117] | Wang Q, Wang F, Xu Z, et al. (2017) Bioactive mushroom polysaccharides: a review on monosaccharide composition, biosynthesis and regulation. Molecules 22. |
[118] |
Chen YC, Wu YJ, Hu CY (2019) Monosaccharide composition influence and immunomodulatory effects of probiotic exopolysaccharides. Int J Biol Macromol 133: 575-582. doi: 10.1016/j.ijbiomac.2019.04.109
![]() |
[119] |
Kumar AS, Mody K, Jha B (2007) Bacterial exopolysaccharides-a perception. J Basic Microbiol 47: 103-117. doi: 10.1002/jobm.200610203
![]() |
1. | Kippeum Lee, Hyeon Ji Kim, Soo A Kim, Soo-Dong Park, Jae-Jung Shim, Jung-Lyoul Lee, Exopolysaccharide from Lactobacillus plantarum HY7714 Protects against Skin Aging through Skin–Gut Axis Communication, 2021, 26, 1420-3049, 1651, 10.3390/molecules26061651 | |
2. | Daniel Abarquero, Erica Renes, José María Fresno, María Eugenia Tornadijo, Study of exopolysaccharides from lactic acid bacteria and their industrial applications: a review, 2022, 57, 0950-5423, 16, 10.1111/ijfs.15227 | |
3. | Jiali Wang, Chengshun Lu, Qiang Xu, Zhongyuan Li, Yajian Song, Sa Zhou, Le Guo, Tongcun Zhang, Xuegang Luo, Comparative Genomics Analysis Provides New Insights into High Ethanol Tolerance of Lactiplantibacillus pentosus LTJ12, a Novel Strain Isolated from Chinese Baijiu, 2022, 12, 2304-8158, 35, 10.3390/foods12010035 | |
4. | Caixia Pei, Hua Lu, Jiayin Ma, Jerry Eichler, Ziqiang Guan, Linlu Gao, Li Liu, Hui Zhou, Jinghua Yang, Cheng Jin, AepG is a glucuronosyltransferase involved in acidic exopolysaccharide synthesis and contributes to environmental adaptation of Haloarcula hispanica, 2023, 299, 00219258, 102911, 10.1016/j.jbc.2023.102911 | |
5. | Seul-Gi Jeong, In Seong Choi, Ho Myeong Kim, Ji Yoon Chang, Hae Woong Park, Supercooling Pretreatment Improves the Shelf-Life of Freeze-Dried Leuconostoc mesenteroides WiKim32, 2022, 32, 1017-7825, 1599, 10.4014/jmb.2209.09022 | |
6. | Trung-Son Le, Phu-Tho Nguyen, Song-Hao Nguyen-Ho, Tang-Phu Nguyen, Thi-Tho Nguyen, My-Ngan Thai, To-Uyen Nguyen-Thi, Minh-Chon Nguyen, Quoc-Khanh Hoang, Huu-Thanh Nguyen, Expression of genes involved in exopolysaccharide synthesis in Lactiplantibacillus plantarum VAL6 under environmental stresses, 2021, 203, 0302-8933, 4941, 10.1007/s00203-021-02479-0 | |
7. | Jinsong Wu, Xiangpeng Han, Meizhi Ye, Yao Li, Xi Wang, Qingping Zhong, Exopolysaccharides synthesized by lactic acid bacteria: biosynthesis pathway, structure-function relationship, structural modification and applicability, 2022, 1040-8398, 1, 10.1080/10408398.2022.2043822 | |
8. | Jieran An, Yuchen Zhang, Zhaoer Zhao, Ran Huan, Huaxi Yi, Hui Wang, Chunguang Luan, Shengbao Feng, Heqiang Huang, Shanwen Li, Deliang Wang, Zhengyuan Zhai, Yanling Hao, Zhenjiang Zech Xu, Molecular Organization and Functional Analysis of a Novel Plasmid-Borne cps Gene Cluster from Lactiplantibacillus plantarum YC41 , 2023, 2165-0497, 10.1128/spectrum.04150-22 | |
9. | Odysseas Sotirios Stergiou, Konstantinos Tegopoulos, Despoina Eugenia Kiousi, Margaritis Tsifintaris, Aristotelis C. Papageorgiou, Chrysoula C. Tassou, Nikos Chorianopoulos, Petros Kolovos, Alex Galanis, Whole-Genome Sequencing, Phylogenetic and Genomic Analysis of Lactiplantibacillus pentosus L33, a Potential Probiotic Strain Isolated From Fermented Sausages, 2021, 12, 1664-302X, 10.3389/fmicb.2021.746659 | |
10. | Ceren Mutlu, Cihadiye Candal-Uslu, Hazal Özhanlı, Sultan Arslan-Tontul, Mustafa Erbas, Modulating of food glycemic response by lactic acid bacteria, 2022, 47, 22124292, 101685, 10.1016/j.fbio.2022.101685 | |
11. | Hae Ran Park, Ji Hee Lee, Hyun Jung Ji, Sangyong Lim, Ki Bum Ahn, Ho Seong Seo, Radioprotection of deinococcal exopolysaccharide BRD125 by regenerating hematopoietic stem cells, 2022, 12, 2234-943X, 10.3389/fonc.2022.898185 | |
12. | Corinna Stühmeier-Niehe, Luca Lass, Miriam Brocksieper, Panagiotis Chanos, Christian Hertel, Pre-Treatment of Starter Cultures with Mild Pulsed Electric Fields Influences the Characteristics of Set Yogurt, 2023, 12, 2304-8158, 442, 10.3390/foods12030442 | |
13. | Thi-Tho Nguyen, Phu-Tho Nguyen, Minh-Nhut Pham, Hary Razafindralambo, Quoc-Khanh Hoang, Huu-Thanh Nguyen, Synbiotics: a New Route of Self-production and Applications to Human and Animal Health, 2022, 14, 1867-1306, 980, 10.1007/s12602-022-09960-2 | |
14. | Navneet Kaur, Priyankar Dey, Bacterial Exopolysaccharides as Emerging Bioactive Macromolecules: From Fundamentals to Applications, 2022, 09232508, 104024, 10.1016/j.resmic.2022.104024 | |
15. | Yifan Zhong, Shanshan Wang, Hanqiu Di, Zhaoxi Deng, Jianxin Liu, Haifeng Wang, Gut health benefit and application of postbiotics in animal production, 2022, 13, 2049-1891, 10.1186/s40104-022-00688-1 | |
16. | Elena Bancalari, Monica Gatti, Benedetta Bottari, Diego Mora, Stefania Arioli, Disclosing Lactobacillus delbrueckii subsp. bulgaricus intraspecific diversity in exopolysaccharides production, 2022, 102, 07400020, 103924, 10.1016/j.fm.2021.103924 | |
17. | Tongthong Tongthong, Warerat Kaewduangduen, Pornpimol Phuengmaung, Wiwat Chancharoenthana, Asada Leelahavanichkul, Lacticaseibacillus rhamnosus dfa1 Attenuate Cecal Ligation-Induced Systemic Inflammation through the Interference in Gut Dysbiosis, Leaky Gut, and Enterocytic Cell Energy, 2023, 24, 1422-0067, 3756, 10.3390/ijms24043756 | |
18. | Chiara La Torre, Alessia Fazio, Paolino Caputo, Antonio Tursi, Patrizia Formoso, Erika Cione, Influence of Three Extraction Methods on the Physicochemical Properties of Kefirans Isolated from Three Types of Animal Milk, 2022, 11, 2304-8158, 1098, 10.3390/foods11081098 | |
19. | Hafize Fidan, Tuba Esatbeyoglu, Vida Simat, Monica Trif, Giulia Tabanelli, Tina Kostka, Chiara Montanari, Salam A. Ibrahim, Fatih Özogul, Recent developments of lactic acid bacteria and their metabolites on foodborne pathogens and spoilage bacteria: Facts and gaps, 2022, 47, 22124292, 101741, 10.1016/j.fbio.2022.101741 | |
20. | Maria Kanwal, Rao Arsalan Khushnood, Fazal Adnan, Abdul Ghafar Wattoo, Amna Jalil, Assessment of the MICP potential and corrosion inhibition of steel bars by biofilm forming bacteria in corrosive environment, 2023, 137, 09589465, 104937, 10.1016/j.cemconcomp.2023.104937 | |
21. | Yolanda González-García, Juan C. Meza-Contreras, José A. Gutiérrez-Ortega, Ricardo Manríquez-González, In Vivo Modification of Microporous Structure in Bacterial Cellulose by Exposing Komagataeibacter xylinus Culture to Physical and Chemical Stimuli, 2022, 14, 2073-4360, 4388, 10.3390/polym14204388 | |
22. | Ahmed Zayed, Mai K. Mansour, Mohamed S. Sedeek, Mohamed H. Habib, Roland Ulber, Mohamed A. Farag, Rediscovering bacterial exopolysaccharides of terrestrial and marine origins: novel insights on their distribution, biosynthesis, biotechnological production, and future perspectives, 2021, 0738-8551, 1, 10.1080/07388551.2021.1942779 | |
23. | Phu-Tho Nguyen, Thi-Tho Nguyen, Thi-To-Uyen Nguyen, Quoc-Khanh Hoang, Huu-Thanh Nguyen, Improve the viability and extracellular polymeric substances bioactivity of Lactiplantibacillus plantarum VAL6 using the environmental adaptation, 2022, 131, 09603085, 149, 10.1016/j.fbp.2021.11.006 | |
24. | Hadi Pourjafar, Fereshteh Ansari, Alireza Sadeghi, Shohre Alian Samakkhah, Seid Mahdi Jafari, Functional and health-promoting properties of probiotics’ exopolysaccharides; isolation, characterization, and applications in the food industry, 2022, 1040-8398, 1, 10.1080/10408398.2022.2047883 | |
25. | Ho Myeong Kim, Seul-Gi Jeong, In Min Hwang, Hae Woong Park, Efficient Citrus (Citrus unshiu) Byproduct Extract-Based Approach forLactobacillus sakeiWiKim31 Shelf-Life Extension, 2021, 6, 2470-1343, 35334, 10.1021/acsomega.1c04335 | |
26. | Thi-Tho Nguyen, Phu-Tho Nguyen, Thi-To-Uyen Nguyen, Thi-Bich-Nhu Nguyen, Nhi-Binh Bui, Huu-Thanh Nguyen, Efficacy of the incorporation between self-encapsulation and cryoprotectants on improving the freeze-dried survival of probiotic bacteria, 2022, 132, 1365-2672, 3217, 10.1111/jam.15473 | |
27. | Hikmate Abriouel, Julia Manetsberger, Natacha Caballero Gómez, Nabil Benomar, In silico genomic analysis of the potential probiotic Lactiplantibacillus pentosus CF2-10N reveals promising beneficial effects with health promoting properties, 2022, 13, 1664-302X, 10.3389/fmicb.2022.989824 | |
28. | Sivasankari Marimuthu, Sharon Mano Pappu J, Karthikeyan Rajendran, Artificial neural network modeling and statistical optimization of medium components to enhance production of exopolysaccharide by Bacillus sp. EPS003, 2023, 53, 1082-6068, 136, 10.1080/10826068.2022.2098322 | |
29. | Maria Dimopoulou, Marguerite Dols-Lafargue, Exopolysaccharides Producing Lactic Acid Bacteria in Wine and Other Fermented Beverages: For Better or for Worse?, 2021, 10, 2304-8158, 2204, 10.3390/foods10092204 | |
30. | Soyoung Park, Kandasamy Saravanakumar, Anbazhagan Sathiyaseelan, Ki-Seok Han, Jooeun Lee, Myeong-Hyeon Wang, Polysaccharides of Weissella cibaria Act as a Prebiotic to Enhance the Probiotic Potential of Lactobacillus rhamnosus, 2022, 0273-2289, 10.1007/s12010-022-04104-2 | |
31. | Ririn Puspadewi, Anggi Gumilar, Tiara Damara Kartikasari, The Ability of Lactobacillus Plantarum to Produce Exopolysaccharides with Additional Nutrients, 2022, 1104, 1755-1307, 012011, 10.1088/1755-1315/1104/1/012011 | |
32. | Fitsum Dejene, Belayneh Regasa Dadi, Dagimawie Tadesse, Elena Sorrentino, In Vitro Antagonistic Effect of Lactic Acid Bacteria Isolated from Fermented Beverage and Finfish on Pathogenic and Foodborne Pathogenic Microorganism in Ethiopia, 2021, 2021, 1687-9198, 1, 10.1155/2021/5370556 | |
33. | Bakhtiyar Islamov, Olga Petrova, Polina Mikshina, Aidar Kadyirov, Vladimir Vorob’ev, Yuri Gogolev, Vladimir Gorshkov, The Role of Pectobacterium atrosepticum Exopolysaccharides in Plant–Pathogen Interactions, 2021, 22, 1422-0067, 12781, 10.3390/ijms222312781 | |
34. | Youssef M. M. Mohammed, Mona M. G. Saad, Samir A. M. Abdelgaleil, Production, characterization and bio-emulsifying application of exopolysaccharides from Rhodotorula mucilaginosa YMM19, 2021, 11, 2190-572X, 10.1007/s13205-021-02898-2 | |
35. | Evandro L de Souza, Kataryne ÁR de Oliveira, Maria EG de Oliveira, Influence of lactic acid bacteria metabolites on physical and chemical food properties, 2023, 49, 22147993, 100981, 10.1016/j.cofs.2022.100981 | |
36. | Aneliya Georgieva, Mariana Petkova, Emanoila Todorova, Velitchka Gotcheva, Angel Angelov, P. Mollov, G. Ivanov, K. Mihalev, G. Kostov, S. Dragoev, Isolation and selection of sauerkraut lactic acid bacteria producing exopolysaccharides, 2023, 58, 2117-4458, 02001, 10.1051/bioconf/20235802001 | |
37. | Raquel Bello-Morales, Sabina Andreu, Vicente Ruiz-Carpio, Inés Ripa, José Antonio López-Guerrero, Extracellular Polymeric Substances: Still Promising Antivirals, 2022, 14, 1999-4915, 1337, 10.3390/v14061337 | |
38. | Chu Wu, Yun Wang, Yujie Yang, Pestalotiopsis Diversity: Species, Dispositions, Secondary Metabolites, and Bioactivities, 2022, 27, 1420-3049, 8088, 10.3390/molecules27228088 | |
39. | Olga V. Averina, Elena U. Poluektova, Mariya V. Marsova, Valery N. Danilenko, Biomarkers and Utility of the Antioxidant Potential of Probiotic Lactobacilli and Bifidobacteria as Representatives of the Human Gut Microbiota, 2021, 9, 2227-9059, 1340, 10.3390/biomedicines9101340 | |
40. | Shuo Yang, Xiaoqing Xu, Qing Peng, Lan Ma, Yu Qiao, Bo Shi, Exopolysaccharides from lactic acid bacteria, as an alternative to antibiotics, on regulation of intestinal health and the immune system, 2023, 24056545, 10.1016/j.aninu.2023.02.004 | |
41. | Min Li, Weicheng Li, Dongyu Li, Juanjuan Tian, Luyao Xiao, Lai-Yu Kwok, Wei Li, Zhihong Sun, Structure characterization, antioxidant capacity, rheological characteristics and expression of biosynthetic genes of exopolysaccharides produced by Lactococcus lactis subsp. lactis IMAU11823, 2022, 384, 03088146, 132566, 10.1016/j.foodchem.2022.132566 | |
42. | Thi-Tho Nguyen, Phu-Tho Nguyen, Thi-Thuy-Vy Nguyen, Thi-To-Uyen Nguyen, Thi-Bich-Nhu Nguyen, Nhi-Binh Bui, Quoc-Khanh Hoang, Huu-Thanh Nguyen, Correlation Between the Amount of Extracellular Polymeric Substances and the Survival Rate to Freeze-Drying of Probiotics, 2022, 79, 0343-8651, 10.1007/s00284-022-02862-0 | |
43. | Muhammad Latif, Syed Asad Hussain Bukhari, Abdullah A. Alrajhi, Fahad S. Alotaibi, Maqshoof Ahmad, Ahmad Naeem Shahzad, Ahmed Z. Dewidar, Mohamed A. Mattar, Inducing Drought Tolerance in Wheat through Exopolysaccharide-Producing Rhizobacteria, 2022, 12, 2073-4395, 1140, 10.3390/agronomy12051140 | |
44. | Giovanna Iosca, Luciana De Vero, Giulia Di Rocco, Giancarlo Perrone, Maria Gullo, Andrea Pulvirenti, Anti-Spoilage Activity and Exopolysaccharides Production by Selected Lactic Acid Bacteria, 2022, 11, 2304-8158, 1914, 10.3390/foods11131914 | |
45. | Cristina Mihaela Nicolescu, Marius Bumbac, Claudia Lavinia Buruleanu, Elena Corina Popescu, Sorina Geanina Stanescu, Andreea Antonia Georgescu, Siramona Maria Toma, Biopolymers Produced by Lactic Acid Bacteria: Characterization and Food Application, 2023, 15, 2073-4360, 1539, 10.3390/polym15061539 | |
46. | Sakineh Abbasi, Parisa Mohammadi, Improving drought tolerance and glycyrrhizin content of licorice plant by EPS-producing cyanobacteria, 2023, 197, 09266690, 116578, 10.1016/j.indcrop.2023.116578 | |
47. | Fedrick C. Mgomi, Yi-ran Yang, Geng Cheng, Zhen-quan Yang, Lactic acid bacteria biofilms and their antimicrobial potential against pathogenic microorganisms, 2023, 25902075, 100118, 10.1016/j.bioflm.2023.100118 | |
48. | Jie Xiong, Dong-mei Liu, Yan-yan Huang, Exopolysaccharides from Lactiplantibacillus plantarum: isolation, purification, structure–function relationship, and application, 2023, 1438-2377, 10.1007/s00217-023-04237-6 | |
49. | Luis-Fernando Patlan-Velázquez, Luis-Guillermo González-Olivares, Mariano García-Garibay, Sergio Alatorre-Santamaría, Lorena Gómez-Ruiz, Gabriela Rodríguez-Serrano, Alma Cruz-Guerrero, Effect of biogenic exopolysaccharides in characteristics and stability of a novel Requeson-type cheese, 2024, 59, 22124292, 103896, 10.1016/j.fbio.2024.103896 | |
50. | Aaron Leininger, Sidan Lu, Jinyue Jiang, Yanhong Bian, Harold D. May, Zhiyong Jason Ren, The convergence of lactic acid microbiomes and metabolites in long-term electrofermentation, 2024, 22, 26664984, 100459, 10.1016/j.ese.2024.100459 | |
51. | Nourhan S. Shehata, Bassma H. Elwakil, Salma S. Elshewemi, Doaa A. Ghareeb, Zakia A. Olama, Selenium nanoparticles coated bacterial polysaccharide with potent antimicrobial and anti-lung cancer activities, 2023, 13, 2045-2322, 10.1038/s41598-023-48921-9 | |
52. | Shangjie Yao, Rongkun Tu, Yao Jin, Rongqing Zhou, Chongde Wu, Jiufu Qin, Improvement of the viability of Tetragenococcus halophilus under acidic stress by forming the biofilm cell structure based on RNA‐Seq and iTRAQ analyses, 2024, 104, 0022-5142, 3559, 10.1002/jsfa.13240 | |
53. | Nayeli Martha-Lucero, Gustavo Viniegra-González, Luis González-Olivares, Alma Cruz-Guerrero, Biofilm formation by agave epiphytic lactic acid bacteria fed with agave fructans, 2023, 39, 0959-3993, 10.1007/s11274-023-03749-3 | |
54. | Abdelbasset Lakhdar, Mohamed Trigui, Francesco Montemurro, An Overview of Biostimulants’ Effects in Saline Soils, 2023, 13, 2073-4395, 2092, 10.3390/agronomy13082092 | |
55. | Seda Nur Köktürk, Hülya Yardimci, Postbiotics and their therapeutic effects: a review, 2024, 35, 2770-3150, 175, 10.1097/MRM.0000000000000383 | |
56. | Naimisha Chowdhury, Romen Singh Naorem, Dibya Jyoti Hazarika, Gunajit Goswami, Abhisek Dasgupta, Sudipta Sankar Bora, Robin Chandra Boro, Madhumita Barooah, An oxalate decarboxylase-like cupin domain containing protein is involved in imparting acid stress tolerance in Bacillus amyloliquefaciens MBNC, 2024, 40, 0959-3993, 10.1007/s11274-023-03870-3 | |
57. | Saumi Pandey, Vinod K. Kannaujiya, Bacterial extracellular biopolymers: Eco-diversification, biosynthesis, technological development and commercial applications, 2024, 279, 01418130, 135261, 10.1016/j.ijbiomac.2024.135261 | |
58. | Ramesh Sharma, Pinku Chandra Nath, Biswanath Bhunia, Tarun Kanti Bandyopadhyay, 2023, Chapter 23, 978-981-99-6830-5, 441, 10.1007/978-981-99-6831-2_23 | |
59. | S. Chaitanya Kumari, Dastagir Mohammed, K. Anuradha, 2024, 9780443139321, 113, 10.1016/B978-0-443-13932-1.00020-9 | |
60. | Arun Kumar, Manti Kumar Saha, Vipin Kumar, Anupam Bhattacharya, Sagar Barge, Ashis K. Mukherjee, Mohan C. Kalita, Mojibur R. Khan, Heat-killed probiotic Levilactobacillus brevis MKAK9 and its exopolysaccharide promote longevity by modulating aging hallmarks and enhancing immune responses in Caenorhabditis elegans, 2024, 21, 1742-4933, 10.1186/s12979-024-00457-w | |
61. | Özge Kahraman-Ilıkkan, Selin Akad Dinçer, Elif Şeyma Bağdat, Işılsu Çağlar, Enes Dertli, Hümeyra İspirli, Feride İffet Şahin, The wound healing effect and DNA damage repair of exopolysaccharide extracted from Weissella cibaria MED17, 2024, 31, 22126198, 100400, 10.1016/j.bcdf.2023.100400 | |
62. | Yamid A. Pinchao, Liliana Serna-Cock, Oswaldo Osorio Mora, Probiotic capacity of commensal lactic acid bacteria from the intestine of Guinea pigs (Cavia porcellus), 2024, 10, 24058440, e29431, 10.1016/j.heliyon.2024.e29431 | |
63. | Nina Katarina Grilc, Julijana Kristl, Špela Zupančič, Can polymeric nanofibers effectively preserve and deliver live therapeutic bacteria?, 2025, 245, 09277765, 114329, 10.1016/j.colsurfb.2024.114329 | |
64. | Geum-Jae Jeong, Fazlurrahman Khan, Nazia Tabassum, Young-Mog Kim, Alteration of oral microbial biofilms by sweeteners, 2024, 7, 25902075, 100171, 10.1016/j.bioflm.2023.100171 | |
65. | Niamat Ullah, Syed Zia Ul Hasnain, Rabia Baloch, Adnan Amin, Aygun Nasibova, Dragica Selakovic, Gvozden Luka Rosic, Sokhib Islamov, Nasibakhon Naraliyeva, Nidal Jaradat, Afat O Mammadova, Exploring essential oil-based bio-composites: molecular docking and in vitro analysis for oral bacterial biofilm inhibition, 2024, 12, 2296-2646, 10.3389/fchem.2024.1383620 | |
66. | Marco Montemurro, Marzia Beccaccioli, Giuseppe Perri, Carlo Giuseppe Rizzello, Massimo Reverberi, Erica Pontonio, A chestnut-hemp type-II sourdough to improve technological, nutritional, and sensory properties of gluten-free bread, 2023, 404, 01681605, 110322, 10.1016/j.ijfoodmicro.2023.110322 | |
67. | Sri Wahyuni, Asnani Asnani, Andi Khaeruni, Novi Dian Puspita Dewi, Sarinah Sarinah, R. H. Fitri Faradilla, Study on physicochemical characteristics of local colored rice varieties (black, red, brown, and white) fermented with lactic acid bacteria (SBM.4A), 2023, 60, 0022-1155, 3035, 10.1007/s13197-023-05813-0 | |
68. | Sabine Michielsen, Gabriel T Vercelli, Otto X Cordero, Herwig Bachmann, Spatially structured microbial consortia and their role in food fermentations, 2024, 87, 09581669, 103102, 10.1016/j.copbio.2024.103102 | |
69. | Haktan Aktaş, Bülent Çetın, Multidimensional evaluation of techno-functional properties of yoghurt bacteria, 2024, 148, 09586946, 105795, 10.1016/j.idairyj.2023.105795 | |
70. | Jiali Wang, Chengshun Lu, Qiang Xu, Zhong-Yuan Li, Ya-Jian Song, Sa Zhou, Shuxian Zhao, Jiqi Li, Xue-Gang Luo, Tong-Cun Zhang, Genomic Analysis of Lactiplantibacillus Pentosus LTJ12, a Novel Strain with High Alcohol Tolerance Isolated from Chinese Baijiu, 2022, 1556-5068, 10.2139/ssrn.4194729 | |
71. | Shah Saud, Tang Xiaojuan, Shah Fahad, The consequences of fermentation metabolism on the qualitative qualities and biological activity of fermented fruit and vegetable juices, 2024, 21, 25901575, 101209, 10.1016/j.fochx.2024.101209 | |
72. | H. Nakibapher Jones Shangpliang, Jyoti Prakash Tamang, Metagenomics and metagenome-assembled genomes mining of health benefits in jalebi batter, a naturally fermented cereal-based food of India, 2023, 172, 09639969, 113130, 10.1016/j.foodres.2023.113130 | |
73. | Hassimi Abu Hasan, Nurul Farhana Mohd Rahim, Jahira Alias, Jamilah Ahmad, Nor Sakinah Mohd Said, Nur Nadhirah Ramli, Junaidah Buhari, Siti Rozaimah Sheikh Abdullah, Ahmad Razi Othman, Hajjar Hartini Wan Jusoh, Hafizan Juahir, Setyo Budi Kurniawan, A Review on the Roles of Extracellular Polymeric Substances (EPSs) in Wastewater Treatment: Source, Mechanism Study, Bioproducts, Limitations, and Future Challenges, 2024, 16, 2073-4441, 2812, 10.3390/w16192812 | |
74. | Nowshin Tarannum, Tanim Jabid Hossain, Ferdausi Ali, Tuhin Das, Kartik Dhar, Iqbal Hossain Nafiz, Antioxidant, antimicrobial and emulsification properties of exopolysaccharides from lactic acid bacteria of bovine milk: Insights from biochemical and genomic analysis, 2023, 186, 00236438, 115263, 10.1016/j.lwt.2023.115263 | |
75. | Mohamed Amine Gacem, Kamel Krantar, Sawsen Hadef, Badreddine Boudjemaa, 2024, 9780323952514, 107, 10.1016/B978-0-323-95251-4.00003-X | |
76. | Anna Jańczuk, Aneta Brodziak, Jolanta Król, Tomasz Czernecki, Properties of Yoghurt Fortified in Lactoferrin with Effect of Storage Time, 2023, 13, 2076-2615, 1610, 10.3390/ani13101610 | |
77. | Gabriela N. Tenea, Jazmin Hidalgo, Jocelyne Pepinos, Clara Ortega, Genome characterization of Leuconostoc pseudomesenteroides UTNElla29 isolated from Morus nigra (L.) fruits: A promising exopolysaccharides producing strain, 2024, 206, 00236438, 116594, 10.1016/j.lwt.2024.116594 | |
78. | Shengnan Liang, Xinyu Wang, Chun Li, Libo Liu, Biological Activity of Lactic Acid Bacteria Exopolysaccharides and Their Applications in the Food and Pharmaceutical Industries, 2024, 13, 2304-8158, 1621, 10.3390/foods13111621 | |
79. | Zongling Chen, Xingyu Huo, Jiali Wan, Jinming Che, Meiyi Deng, Yingnan Bao, Hailin Yang, Yanjun Tong, Shoushuai Feng, Enhancing acid resistance of Escherichia coli based on directed morphology evolutionary of key transcription factor bolA, 2024, 62, 22124292, 105291, 10.1016/j.fbio.2024.105291 | |
80. | O. A. Svitich, A. V. Poddubikov, N. O. Vartanova, A. Yu. Leonova, E. A. Kurbatova, Biofilm Formation by Lactobacillus Strains of Modern Probiotics and Their Antagonistic Activity against Opportunistic Bacteria, 2024, 177, 0007-4888, 476, 10.1007/s10517-024-06211-y | |
81. | Rajesh Jeewon, Aadil Ahmad Aullybux, Daneshwar Puchooa, Nadeem Nazurally, Abdulwahed Fahad Alrefaei, Ying Zhang, Marine Microbial Polysaccharides: An Untapped Resource for Biotechnological Applications, 2023, 21, 1660-3397, 420, 10.3390/md21070420 | |
82. | Bio-Prospecting Xylose-Utilizing, Exopolysaccharide (EPS)-Producing Bacteria and EPS Quantification through Submerged Fermentation using Xylose as the Major Carbon Source, 2024, 2814-1822, 13, 10.47430/ujmr.2493.003 | |
83. | Siqun Tang, Jilai Gong, Biao Song, Juan Li, Weicheng Cao, Jun Zhao, Remediation of biochar-supported effective microorganisms and microplastics on multiple forms of nitrogenous and phosphorous in eutrophic lake, 2024, 956, 00489697, 177142, 10.1016/j.scitotenv.2024.177142 | |
84. | Polina Mikshina, Maria Kharina, Alya Sungatullina, Tatyana Petrova, Timur Sibgatullin, Elena Nikitina, Influence of flaxseed mucilage on the formation, composition, and properties of exopolysaccharides produced by different strains of lactic acid bacteria, 2024, 281, 01418130, 136092, 10.1016/j.ijbiomac.2024.136092 | |
85. | Se-Young Kwun, Jeong-Ah Yoon, Ga-Yeon Kim, Young-Woo Bae, Eun-Hee Park, Myoung-Dong Kim, Isolation of a Potential Probiotic Levilactobacillus brevis and Evaluation of Its Exopolysaccharide for Antioxidant and α-Glucosidase Inhibitory Activities, 2024, 34, 1017-7825, 167, 10.4014/jmb.2304.04043 | |
86. | Phu-Tho Nguyen, Huu-Thanh Nguyen, Environmental stress for improving the functionality of lactic acid bacteria in malolactic fermentation, 2024, 4, 29501946, 100138, 10.1016/j.microb.2024.100138 | |
87. | Sharon Y. Geerlings, Kees van der Ark, Bart Nijsse, Sjef Boeren, Mark van Loosdrecht, Clara Belzer, Willem M. de Vos, Omics-based analysis of Akkermansia muciniphila cultivation in food-grade media, 2024, 3, 2771-5965, 10.20517/mrr.2024.06 | |
88. | Gökhan Kürşad İncili, Roghayieh Razavi, Ali Adnan Hayaloğlu, Ahmadreza Abedinia, Seyedeh Sahar Mirmoeini, Mehran Moradi, 2025, 9780443135675, 55, 10.1016/B978-0-443-13567-5.00003-4 | |
89. | Akshay Ramani, Subhadip Manik, Tanmay Hazra, Sheweta Barak, Deepak Mudgil, 2023, Chapter 9, 978-981-99-5458-2, 167, 10.1007/978-981-99-5459-9_9 | |
90. | Kevser Karaman, Sibel Turan Sirke, Şeyda Nur Türkay Rifaioglu, Molecular identification of lactic acid bacteria from traditional fermented foods and screening exopolysaccharide production by using food wastes, 2024, 0015-5632, 10.1007/s12223-024-01187-8 | |
91. | Pascal Drouin, Érica Benjamim da Silva, Julien Tremblay, Eric Chevaux, Emmanuelle Apper, Mathieu Castex, Inoculation with Lentilactobacillus buchneri alone or in combination with Lentilactobacillus hilgardii modifies gene expression, fermentation profile, and starch digestibility in high-moisture corn, 2023, 14, 1664-302X, 10.3389/fmicb.2023.1253588 | |
92. | Vishal Ahuja, Shikha Chauhan, Diptarka Dasgupta, Puneet Wadhwa, Tirath Raj, Yung-Hun Yang, Shashi Kant Bhatia, Microbial exopolysaccharide composites with inorganic materials and their biomedical applications: A review, 2024, 7, 26668939, 100482, 10.1016/j.carpta.2024.100482 | |
93. | Angel Angelov, Aneliya Georgieva, Mariana Petkova, Elena Bartkiene, João Miguel Rocha, Manol Ognyanov, Velitchka Gotcheva, On the Molecular Selection of Exopolysaccharide-Producing Lactic Acid Bacteria from Indigenous Fermented Plant-Based Foods and Further Fine Chemical Characterization, 2023, 12, 2304-8158, 3346, 10.3390/foods12183346 | |
94. | Monic Andrew, Gurunathan Jayaraman, Production optimization and antioxidant potential of exopolysaccharide produced by a moderately halophilic bacterium Virgibacillus dokdonensis VITP14 , 2024, 1082-6068, 1, 10.1080/10826068.2024.2370879 | |
95. | Sizhe Qiu, Aidong Yang, Hong Zeng, Christos A. Ouzounis, Flux balance analysis-based metabolic modeling of microbial secondary metabolism: Current status and outlook, 2023, 19, 1553-7358, e1011391, 10.1371/journal.pcbi.1011391 | |
96. | Giorgia Rampanti, Andrea Cantarini, Federica Cardinali, Vesna Milanović, Cristiana Garofalo, Lucia Aquilanti, Andrea Osimani, Technological and Enzymatic Characterization of Autochthonous Lactic Acid Bacteria Isolated from Viili Natural Starters, 2024, 13, 2304-8158, 1115, 10.3390/foods13071115 | |
97. | Thuy-Trang Pham, Thanh-Dung Nguyen, Thi-Tho Nguyen, Minh-Nhut Pham, Phu-Tho Nguyen, To-Uyen Thi Nguyen, Thanh-Tam Ngoc Huynh, Huu-Thanh Nguyen, Rhizosphere bacterial exopolysaccharides: composition, biosynthesis, and their potential applications, 2024, 206, 0302-8933, 10.1007/s00203-024-04113-1 | |
98. | Saba Kavian, Mehdi Zarei, Ali Niazi, Reza Ghasemi-Fasaei, Amir Ghaffar Shahriari, Tibor Janda, Morphophysiological and Biochemical Responses of Zea mays L. under Cadmium and Drought Stresses Integrated with Fungal and Bacterial Inoculation, 2023, 13, 2073-4395, 1675, 10.3390/agronomy13071675 | |
99. | Lebea N. Nthunya, Tshepiso J. Mpala, Anita Etale, Oranso T. Mahlangu, Mahloro Hope Serepa-Dlamini, Eduardo A. Lopez-Maldonado, Heidi Richards, Biofouling control of thermophilic bacteria in membrane distillation, 2024, 320, 19443986, 100627, 10.1016/j.dwt.2024.100627 | |
100. | Qianru Lin, Mingwang Liu, Hao Ni, Yue Hao, Yiqun Yu, Yiran Chen, Qing Wu, Yi Shen, Lei Zhang, Mingsheng Lyu, Shujun Wang, High-degree polymerizate IMOs of dextranase hydrolysates enhance Lactobacillus acid metabolism: Based on growth, and metabolomic and transcriptomic analyses, 2023, 187, 00236438, 115345, 10.1016/j.lwt.2023.115345 | |
101. | Felipe Martins de Souza, Ram K. Gupta, Bacteria for Bioplastics: Progress, Applications, and Challenges, 2024, 9, 2470-1343, 8666, 10.1021/acsomega.3c07372 | |
102. | Jihen Elleuch, Marwa Drira, Imtinen Ghribi, Farah Hadjkacem, Guillaume Pierre, Hamadi Khemakhem, Philippe Michaud, Imen Fendri, Slim Abdelkafi, Lead removal from the aqueous solution by extracellular polymeric substances produced by the marine diatom Navicula salinicola , 2024, 0959-3330, 1, 10.1080/09593330.2024.2338456 | |
103. | Nina Čuljak, Barbara Bellich, Alice Pedroni, Katarina Butorac, Andreja Leboš Pavunc, Jasna Novak, Martina Banić, Jagoda Šušković, Paola Cescutti, Blaženka Kos, Limosilactobacillus fermentum strains MC1 and D12: Functional properties and exopolysaccharides characterization, 2024, 273, 01418130, 133215, 10.1016/j.ijbiomac.2024.133215 | |
104. | Siqun Tang, Jilai Gong, Biao Song, Weicheng Cao, Juan Li, Remediation of biochar-supported effective microorganisms and microplastics on multiple forms of heavy metals in eutrophic lake, 2024, 465, 03043894, 133098, 10.1016/j.jhazmat.2023.133098 | |
105. | Yingxue Yue, Yuqi Wang, Yu Han, Yifan Zhang, Ting Cao, Guicheng Huo, Bailiang Li, Genome Analysis of Bifidobacterium Bifidum E3, Structural Characteristics, and Antioxidant Properties of Exopolysaccharides, 2023, 12, 2304-8158, 2988, 10.3390/foods12162988 | |
106. | Leandro Wagner Figueira, Ana Bessa Muniz, Anelise Cristina Osorio Cesar Doria, Thalita Mayumi Castaldelli Nishime, Konstantin Georgiev Kostov, Cristiane Y. Koga-Ito, Inhibitory effect of helium cold atmospheric plasma on cariogenic biofilms, 2024, 16, 2000-2297, 10.1080/20002297.2024.2397831 | |
107. | Yeong Yeol Kim, Jong-Cheol Kim, Seulbi Kim, Jung Eun Yang, Ho Myeong Kim, Hae Woong Park, Heterotypic stress-induced adaptive evolution enhances freeze-drying tolerance and storage stability of Leuconostoc mesenteroides WiKim33, 2024, 175, 09639969, 113731, 10.1016/j.foodres.2023.113731 | |
108. | E. A. Pozhidaeva, E. S. Popov, N. S. Rodionova, Y. A. Dymovskikh, Y. V. Durova, M. S. Grebennikova, S. Sadullozoda, S. Voinash, Y. Ospanov, Study of regime parameters of the process of cultivation of a consortium of probiotic microorganisms providing increased synthesis of exopolysaccharides, 2024, 126, 2117-4458, 01044, 10.1051/bioconf/202412601044 | |
109. | Mahdi Asghari Ozma, Seyyed Reza Moaddab, Hedayat Hosseini, Ehsaneh Khodadadi, Reza Ghotaslou, Mohammad Asgharzadeh, Amin Abbasi, Fadhil S Kamounah, Leili Aghebati Maleki, Khudaverdi Ganbarov, Hossein Samadi Kafil, A critical review of novel antibiotic resistance prevention approaches with a focus on postbiotics, 2023, 1040-8398, 1, 10.1080/10408398.2023.2214818 | |
110. | Digambar Kavitake, Swati Tiwari, Irshad Ahmad Shah, Palanisamy Bruntha Devi, Cedric Delattre, G. Bhanuprakash Reddy, Prathapkumar Halady Shetty, Antipathogenic potentials of exopolysaccharides produced by lactic acid bacteria and their food and health applications, 2023, 152, 09567135, 109850, 10.1016/j.foodcont.2023.109850 | |
111. | Zakaria A. Mohamed, Rehab O. Elnour, Saad Alamri, Mohamed Hashem, Increased production of extracellular polysaccharides in Arthrospira platensis as a protective response against saxitoxin: Implications to outdoor mass production, 2023, 74, 22119264, 103184, 10.1016/j.algal.2023.103184 | |
112. | Inga Bazukyan, Dimitrina Georgieva-Miteva, Tsvetelina Velikova, Svetoslav G. Dimov, In Silico Probiogenomic Characterization of Lactobacillus delbrueckii subsp. lactis A4 Strain Isolated from an Armenian Honeybee Gut, 2023, 14, 2075-4450, 540, 10.3390/insects14060540 | |
113. | Cecilia Castro-López, Alfonso García-Galaz, Hugo S. García, Aarón F. González-Córdova, Belinda Vallejo-Cordoba, Adrián Hernández-Mendoza, Potential probiotic lactobacilli strains isolated from artisanal Mexican Cocido cheese: evidence-based biosafety and probiotic action-related traits on in vitro tests, 2023, 54, 1517-8382, 2137, 10.1007/s42770-023-01059-2 | |
114. | Rina Su, Xinyuan Cui, Hao Guan, Wencan Ke, Ying Liang, Hu Chen, Neha Sheoran, Mengya Jia, Yiling Yang, Lizhuang Hao, Guojun Zhao, Xusheng Guo, Effect of psychrotrophic Lactiplantibacillus plantarum L75 with exoploysaccharides-producing property on fermentation, bacterial community, and antioxidant activity of oat silage at low temperature, 2024, 318, 03778401, 116150, 10.1016/j.anifeedsci.2024.116150 | |
115. | Sampat Nehra, Raj Kumar Gothwal, Alok Kumar Varshney, Pooran Singh Solanki, Poonam Meena, P.C. Trivedi, P. Ghosh, 2023, 9789815050264, 145, 10.2174/9789815050264123020012 | |
116. | Manoj Kumar Yadav, Ji Hoon Song, Robie Vasquez, Jae Seung Lee, In Ho Kim, Dae-Kyung Kang, Methods for Detection, Extraction, Purification, and Characterization of Exopolysaccharides of Lactic Acid Bacteria—A Systematic Review, 2024, 13, 2304-8158, 3687, 10.3390/foods13223687 | |
117. | Sangram Keshari Samal, Debadas Sahoo, Diptikanta Acharya, Alterations in structural components of extracellular polymeric substance of epilithic bacteria Brevundimonas faecalis BC1 growing on monumental rock under thermal stress , 2024, 0892-7014, 1, 10.1080/08927014.2024.2432970 | |
118. | Tatyana S Tikhomirova, Maxim R Taraskevich, Yuriy A Lepekhin, Marina P Shevelyova, Vitaliy A Nemashkalov, Optimization and scaling up of extracellular polysaccharide production by submerged culture of Ganoderma lucidum on starch-containing medium using response surface methodology and laboratory bioreactors of various designs, 2024, 77, 1472-765X, 10.1093/lambio/ovae115 | |
119. | Himanshi Kain, Ena Gupta, Prashant Sharma, Akanksha Haldiya, Vijay Kumar Srivastava, Ravi Ranjan Kumar Neeraj, Pradeep Sharma, S. L. Kothari, Sandip Patil, Shaowei Dong, Anupam Jyoti, Sanket Kaushik, Rolling down the pilus formation of gram-positive bacteria: underlining the importance of Sortase C as a drug target, 2024, 0892-7014, 1, 10.1080/08927014.2024.2426167 | |
120. | Shagun Sharma, Vandana Jhalora, Shubhita Mathur, Renu Bist, A Comparison of Antibiotics’ Resistance Patterns of E. coli and B. subtilis in their Biofilms and Planktonic Forms, 2025, 25, 18715265, 10.2174/0118715265278809240101073539 | |
121. | Hao Liting, Zhou Hongliang, He Yuanyuan, Fu Bowei, Li Miao, Hao Xiaodi, Optimizing vanadium(V) removal in groundwater: Influence of packing modes in bioreactors with machine learning predictions, 2025, 69, 22147144, 106820, 10.1016/j.jwpe.2024.106820 | |
122. | Chandni Upadhyaya, Hiren Patel, Ishita Patel, Trushit Upadhyaya, Extremophilic Exopolysaccharides: Bioprocess and Novel Applications in 21st Century, 2025, 11, 2311-5637, 16, 10.3390/fermentation11010016 | |
123. | Vladimir V. Martirosyan, Marina N. Kostyuchenko, Mikhail V. Reynov, Olga E. Tyurina, Olesia A. Savkina, Study of Exopolysaccharide Production by Lactic Acid Bacteria used in the Baking Industry and Comparison of Methods for Their Determination, 2024, 2, 2949-6497, 10.37442/fme.2024.4.67 | |
124. | Xiaona He, Yu Cui, Qiaoyu Jia, Yongliang Zhuang, Ying Gu, Xuejing Fan, Yangyue Ding, Response mechanisms of lactic acid bacteria under environmental stress and their application in the food industry, 2025, 64, 22124292, 105938, 10.1016/j.fbio.2025.105938 | |
125. | Helena Mylise Copeland, Susan Maye, George MacLeod, Dermot Brabazon, Christine Loscher, Brian Freeland, Statistical optimisation and analysis of biomass and exopolysaccharide production by Lacticaseibacillus rhamnosus LRH30, 2025, 41, 0959-3993, 10.1007/s11274-025-04273-2 | |
126. | Stylianos Exarhopoulos, Euripides Krystallis, Eleni Rousi, Olga Groztidou, Despoina Georgiou, Eleni P. Kalogianni, Athanasios Goulas, Georgia Dimitreli, Effect of Thermal Treatment and the Addition of Texture Modifiers on the Rheological Properties and the Microflora of Reconstituted Kefir Powder, 2025, 5, 2673-6209, 7, 10.3390/macromol5010007 | |
127. | Arif Nurkanto, Asrul Fanani, Dian Alfian Nurcahyanto, Jendri Mamangkey, Sarah Marissa, Khatarina Meldawati Pasaribu, Fifi Afiati, Ni Wayan Sri Agustini, Riza Zainuddin Ahmad, Muhammad Taufik, Herman Irawan, Yelin Adalina, Mia Kusmiati, Erlindha Gangga, Anti-hypercholesterolemia properties of exopolysaccharide from Lactiplantibacillus plantarum MI01: computational and in-vivo approaches, 2025, 26660164, 101146, 10.1016/j.cscee.2025.101146 | |
128. | Ahmad Almatroudi, Biofilm Resilience: Molecular Mechanisms Driving Antibiotic Resistance in Clinical Contexts, 2025, 14, 2079-7737, 165, 10.3390/biology14020165 | |
129. | Xinyu Yang, Zhijie Yang, Yanbo Wang, Hong Zeng, Bei Wang, Proteomics and metabolomics elucidate the biosynthetic pathway of acid stress-induced exopolysaccharides and its impact on growth phenotypes in Lactiplantibacillus plantarum HMX2, 2025, 476, 03088146, 143431, 10.1016/j.foodchem.2025.143431 | |
130. | Georgia Dimitreli, Stylianos Exarhopoulos, Parthena Apidopoulou, Olga Groztidou, Despoina Georgiou, Eleni P. Kalogianni, Athanasios Goulas, Effect of Final Fermentation pH and Pre-Drying Storage Temperature on Properties of Kefir Powder Produced by Kefir Grains, 2025, 15, 2076-3417, 2509, 10.3390/app15052509 | |
131. | Tchouli Noufeu, Yueqin Li, Ndeye Fatou Toure, Hui Yao, Xiaoqun Zeng, Qiwei Du, Daodong Pan, Overview of Glycometabolism of Lactic Acid Bacteria During Freeze-Drying: Changes, Influencing Factors, and Application Strategies, 2025, 14, 2304-8158, 743, 10.3390/foods14050743 | |
132. | Aidalú Hernández-Martínez, Carlos Jiménez-Pérez, Alma Cruz-Guerrero, John F. Trant, Sergio Alatorre-Santamaría, 2025, Production of Exopolysaccharides Through Fermentation of Secondary Whey with Kefir Grains, 44, 10.3390/blsf2024040044 | |
133. | Hatice Oto, H. Ceren Akal, Gökçe Eminoğlu, Enhancing synbiotic dairy beverages with chemically cross-linked inulin for improved texture and stability, 2025, 0022-1155, 10.1007/s13197-025-06243-w | |
134. | Jiayi Lin, Chi Zhao, Jvliang Dai, Yu Zhang, Fangming Lan, Lixin Luo, Unravelling the response mechanism of extracellular polysaccharides synthesis and salt stress resistance in Tetragenococcus halophilus JY1biofilm formation, 2025, 66, 22124292, 106327, 10.1016/j.fbio.2025.106327 | |
135. | Angela Maria Catania, Alessandra Dalmasso, Patrizia Morra, Emanuele Costa, Maria Teresa Bottero, Pierluigi Aldo Di Ciccio, Effect of gaseous ozone treatment on cells and biofilm of dairy Bacillus spp. isolates, 2025, 16, 1664-302X, 10.3389/fmicb.2025.1538456 | |
136. | Siyuan Liu, Yi Wang, Chun Xu, Suppressive effects of lemon myrtle extract against the colonization and virulence factors of Candida spp., 2025, 67, 13490079, 100657, 10.1016/j.job.2025.100657 | |
137. | Anita Morris, Charles Boeneke, Joan M. King, Comparison of Storage Stability and In Vitro Digestion of Rice Flour-Based Yogurt Alternatives Made with Lactobacillus rhamnosus Lgg to Milk-Based Yogurt, 2025, 14, 2304-8158, 1129, 10.3390/foods14071129 |
Stress exposure | Strains | Effect on EPS production: Inhibition (-) Stimulation (+) | References |
Low pH | L. rhamnosus GG | − | Lebeer et al., 2007 [97] |
L. reuteri | + | Slížová et al., 2015 [98] | |
L. helveticus ATCC 15807 | + | Torino et al., 2005 [99] | |
L. salivarius UCO_979C-2 | + | Sanhueza et al., 2015 [100] | |
High temperature | B. bifidum | + | Nguyen et al., 2014 [31] |
Sodium chloride | L. helveticus ATCC 15807 | − | Torino et al., (2005) [99] |
Leuconostoc mesenteroides/pseudomesenteroides 406 | + | Silvia-Simona GROSU-TUDOR, 2014 [101] | |
L. confusus TISTR 1498 | + | Seesuriyachan, 2012 [80] | |
Carbon dioxide | B. longum JBL05 | + | Ninomiya et al., 2009 [89] |
L. casei | + | Santillan et al., 2015 [90] | |
Oxidation | B. longum | − | Golowczyc et al., 2011 [91] |
B. scardovii; B. adolescentis | + | Qian, Borowski, & Calhoon, 2011 [92] | |
Excessive nitrogen source | L. delbrueckii ssp. bulgaricus | + | García-Garibay & Marshall, 2008 [78] |
Excessive carbon source | L. confusus TISTR 1498 | + | Seesuriyachan et al., 2012 [80] |
L. (delbrueckii bulgaricus, helveticus and casei) | + | Hussein et al., 2015 [81] | |
F. sanfranciscensis LTH2590 | + | Korakli, Pavlovic, & Vogel, 2003 [82] | |
Leuconostoc mesenteroides NRRL B-1299 | + | Dols, Remaud-Simeon, & Monsan, 1997 [83] | |
S. thermophilus W22 and L. delbrueckii subsp. bulgaricus (B3, G12) | + | Yuksekdag & Aslim, 2008 [84] | |
L. Casei CG11 | + | Cerning et al., 1994 [85] | |
L. rhamnosus C83 | + | Gamar, Blondeau, & Simonet, 2003 [86] | |
Co-cultivation | L. kefiranofaciens JCM 6985 | + | Tada et al., 2007 [106] |
L. rhamnosus (ATCC 9595, R0011, and RW-9595M) | + | Bertsch, Roy, & LaPointe, 2019 [107] | |
L. paracasei ATCC 334 | + | Yamasaki-Yashiki, Sawada, Kino-oka, & Katakura, 2016 [108] |
Stress exposure | Strains | Effect on EPS production: Inhibition (-) Stimulation (+) | References |
Low pH | L. rhamnosus GG | − | Lebeer et al., 2007 [97] |
L. reuteri | + | Slížová et al., 2015 [98] | |
L. helveticus ATCC 15807 | + | Torino et al., 2005 [99] | |
L. salivarius UCO_979C-2 | + | Sanhueza et al., 2015 [100] | |
High temperature | B. bifidum | + | Nguyen et al., 2014 [31] |
Sodium chloride | L. helveticus ATCC 15807 | − | Torino et al., (2005) [99] |
Leuconostoc mesenteroides/pseudomesenteroides 406 | + | Silvia-Simona GROSU-TUDOR, 2014 [101] | |
L. confusus TISTR 1498 | + | Seesuriyachan, 2012 [80] | |
Carbon dioxide | B. longum JBL05 | + | Ninomiya et al., 2009 [89] |
L. casei | + | Santillan et al., 2015 [90] | |
Oxidation | B. longum | − | Golowczyc et al., 2011 [91] |
B. scardovii; B. adolescentis | + | Qian, Borowski, & Calhoon, 2011 [92] | |
Excessive nitrogen source | L. delbrueckii ssp. bulgaricus | + | García-Garibay & Marshall, 2008 [78] |
Excessive carbon source | L. confusus TISTR 1498 | + | Seesuriyachan et al., 2012 [80] |
L. (delbrueckii bulgaricus, helveticus and casei) | + | Hussein et al., 2015 [81] | |
F. sanfranciscensis LTH2590 | + | Korakli, Pavlovic, & Vogel, 2003 [82] | |
Leuconostoc mesenteroides NRRL B-1299 | + | Dols, Remaud-Simeon, & Monsan, 1997 [83] | |
S. thermophilus W22 and L. delbrueckii subsp. bulgaricus (B3, G12) | + | Yuksekdag & Aslim, 2008 [84] | |
L. Casei CG11 | + | Cerning et al., 1994 [85] | |
L. rhamnosus C83 | + | Gamar, Blondeau, & Simonet, 2003 [86] | |
Co-cultivation | L. kefiranofaciens JCM 6985 | + | Tada et al., 2007 [106] |
L. rhamnosus (ATCC 9595, R0011, and RW-9595M) | + | Bertsch, Roy, & LaPointe, 2019 [107] | |
L. paracasei ATCC 334 | + | Yamasaki-Yashiki, Sawada, Kino-oka, & Katakura, 2016 [108] |