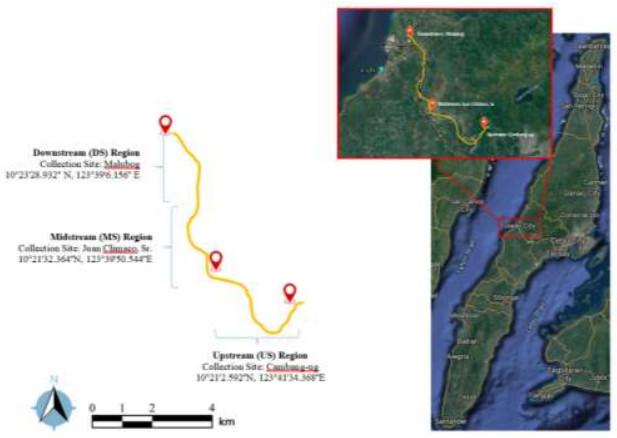
Citation: Joje Mar P. Sanchez, Marchee T. Picardal, Marnan T. Libres, Hedeliza A. Pineda, Ma. Lourdes B. Paloma, Judelynn M. Librinca, Reginald Raymund A. Caturza, Sherry P. Ramayla, Ruby L. Armada, Jay P. Picardal. Characterization of a river at risk: the case of Sapangdaku River in Toledo City, Cebu, Philippines[J]. AIMS Environmental Science, 2020, 7(6): 559-574. doi: 10.3934/environsci.2020035
[1] | Riri Novita Sunarti, Sri Budiarti, Marieska Verawaty, Bayo Alhusaeri Siregar, Poedji Loekitowati Hariani . Diversity of Antibiotic-Resistant Escherichia coli from Rivers in Palembang City, South Sumatra, Indonesia. AIMS Environmental Science, 2022, 9(5): 721-734. doi: 10.3934/environsci.2022041 |
[2] | Romie D. Laranjo, Maria Rio A. Naguit, Farida C. Jamolod, Kristine Gladys E. Jambre, Norma I. Cabornay, Victor B. Bernido, Maricon Denber S. Gahisan . Evaluation of the physicochemical parameters on the water quality of the major rivers of Zamboanga del Norte, Philippines. AIMS Environmental Science, 2023, 10(3): 382-397. doi: 10.3934/environsci.2023022 |
[3] | Muhammad Andang Novianta, Syafrudin, Budi Warsito, Siti Rachmawati . Monitoring river water quality through predictive modeling using artificial neural networks backpropagation. AIMS Environmental Science, 2024, 11(4): 649-664. doi: 10.3934/environsci.2024032 |
[4] | Seiran Haghgoo, Jamil Amanollahi, Barzan Bahrami Kamangar, Shahryar Sorooshian . Decision models enhancing environmental flow sustainability: A strategic approach to water resource management. AIMS Environmental Science, 2024, 11(6): 900-917. doi: 10.3934/environsci.2024045 |
[5] | Adrian Schmid-Breton . Transboundary flood risk management in the Rhine river basin. AIMS Environmental Science, 2016, 3(4): 871-888. doi: 10.3934/environsci.2016.4.871 |
[6] | Périne Doyen, Etienne Morhain, François Rodius . Modulation of metallothionein, pi-GST and Se-GPx mRNA expression in the freshwater bivalve Dreissena polymorpha transplanted into polluted areas. AIMS Environmental Science, 2015, 2(2): 333-344. doi: 10.3934/environsci.2015.2.333 |
[7] | Djordje Vilimanovic, Gangadhar Andaluri, Robert Hannah, Rominder Suri, A. Ronald MacGillivray . Occurrence and aquatic toxicity of contaminants of emerging concern (CECs) in tributaries of an urbanized section of the Delaware River Watershed. AIMS Environmental Science, 2020, 7(4): 302-319. doi: 10.3934/environsci.2020019 |
[8] | Rokhana Dwi Bekti, Kris Suryowati, Maria Oktafiana Dedu, Eka Sulistyaningsih, Erma Susanti . Machine learning and topological kriging for river water quality data interpolation. AIMS Environmental Science, 2025, 12(1): 120-136. doi: 10.3934/environsci.2025006 |
[9] | Kamonrat Suphawan, Kuntalee Chaisee . Gaussian process regression for predicting water quality index: A case study on Ping River basin, Thailand. AIMS Environmental Science, 2021, 8(3): 268-282. doi: 10.3934/environsci.2021018 |
[10] | G Mathias Kondolf, Pilar Lopez-Llompart . National-local land-use conflicts in floodways of the Mississippi River system. AIMS Environmental Science, 2018, 5(1): 47-63. doi: 10.3934/environsci.2018.1.47 |
Water is fundamental for life to exist and sustain on Earth [1]. This essential substance constitutes 75% of the planet's surface in the form of bodies of water [2]. These bodies of water house one-fourth of all identified living organisms, a species number estimated to be around 2.2 million [3]. Of this number, many organisms thrive in freshwater forms like rivers and lakes. Rivers offer 2% of the fresh surface water resources [4,5] to both terrestrial animals and humans for consumption and industrial use, respectively [6,7]. However, rivers are vulnerable targets of industrial and domestic pollution and eutrophication, thereby leading to the destruction of aquatic ecosystems and contamination of consumable water in several parts of the globe, including those river systems found in the Philippines.
In the Philippines, river systems are affected by agricultural (45%), domestic (31%), and industrial (24%) development [8]. World Bank noted that the quantity and quality of water, including those in river systems, are greatly affected, especially in these four regions at risk, namely, Metro Manila, Central Luzon, Southern Tagalog, and Central Visayas [9]. EMB further identified 14 rivers (e.g., Meycauayan River, Calapan River, and Guadalupe River) in the aforementioned regions, which are under the "Sagip Ilog Program" (Save the River Program) of the Department of Environment and Natural Resources [DENR] [8]. Such a list is based on poor water quality, requires monitoring, and those that should undergo a rehabilitation program. Part of the monitoring and assessment of river quality is the continuous physicochemical and microbial analyses by concerned agencies.
Analytical river studies in Luzon like those implemented in the Pasig River in Metro Manila [1] and Balatuin River in Laguna [10] studied untreated wastewater discharges from industrial and domestic sources. The result of both studies seemed to concur that these rivers are highly polluted. In Cebu, EMB found out that some major rivers have poor water quality. Four major rivers have been monitored by DENR since 2014 [8]. Of these four rivers, Luyang River in the municipality of Carmen is in relatively "good condition" while the other three rivers namely Butuanon River (Mandaue City), Guadalupe River (Cebu City), and Sapangdaku River (Toledo City) have shown signs of pollution. While several researchers have already studied Butuanon and Guadalupe rivers [12,13], there are only a few published studies about the condition of Sapangdaku River such that of Lo and Sakamoto [14].
Sapangdaku River, also called Hinulawan River, is situated in estimated terrain elevation of 5 meters above sea level running a long stretch of 9 kilometers across 10 barangays in Toledo City [15]. Riverine communities (i.e., 2010 Philippine Statistics Authority census reported ~ 41,000 people are residing in the immediate vicinity of the river), and a copper ore mine have been operating within the vicinity that could affect the water quality of the Sapangdaku River. Physicochemical, metal and microbial analyses are deemed important to determine the current condition of the river. The results of the physicochemical and microbial assessment provide the baseline data for the Sapangdaku River and give information on how activities of the people may have affected the quality of the river; thus, the conduct of the study.
The study carried out a descriptive quantitative assessment on the Sapangdaku River in Toledo City, southern Cebu, Philippines. Three sampling stations were established along the stretch of the river, namely the upstream [US] in Brgy. Cambang-ug (10°21'2.592''N, 123°41'34.368''E), midstream [MS] in Brgy. Juan Climaco, Sr. (10°21'32.364''N, 123°39'50.544''E), and downstream [DS] in Brgy. Malubog (10°23'28.932'' N, 123°39'6.156'' E) as illustrated in Figure 1. According to the DENR River Basin Control Office Sapangdaku River Basin has a drainage area of 147 km2 and an estimated 88 million cubic meter (MCM) annual run-off (RCBO, 2015). The catchment is classified under Type Ⅲ weather in the Corona climate classification and experiences dry season from November to April and wet season for the other months of the year [15]. Biga Pit, a huge mine tailing deposit facility, is part of the Sapangdaku catchment.
The copper mine operates near the US station, where few residential houses are found and large trucks collect sand and gravel in the water. Grasses and trees thrive and cows and goats graze in the riverside section of the MS station, where more communities live than the US portion of the river. Sand and gravel quarrying and domestic activities such as washing clothes are evident in the middle area of the river. Commercial establishments and national highway are found in the DS station, where most communities live among the three stations. More domestic and industrial affluents are found in this low riverine portion due to the presence of these establishments and communities.
Water sampling methods are based on the guidelines formulated by the American Public Health Association [APHA], American Water Works Association [AWWA], and Water Environment Federation [WEF] [16]. Briefly, five samples were collected in each station. These samples were collected beneath the surface of undisturbed water by opening the container below the surface and collecting water at the surface, and then labeled with unique sample number. All the samples were taken during the cool dry season, collected from 9 to 11 o'clock in the morning, and sampled by the same team under the guidance of a water lab in-charge. After collection, all water samples were immediately transported to Technolab Analytical Group, Inc. in Casuntingan, Mandaue City.
Physicochemical parameters such as temperature, streamflow, dissolved oxygen [DO], chemical oxygen demand [COD], biological oxygen demand [BOD], and total dissolved solids [TDS] were measured on-site using 2150: conductivity meter, float method, 5400-O: azide modification, 5220 B: open reflux, 5210 B: 5-Day test, and 2540: dried at 180 ℃, respectively. Other physicochemical parameters such as pH, conductivity, turbidity, and total suspended solids [TSS] were tested immediately at Technolab Analytical Group, Inc. using 4500-H+ B: electrometric method, 2510: conductivity meter, 2130 B: nephelometric method, and 2540 D: dried at 103–105 ℃, respectively.
Metal and microbiological analyses were also tested in the laboratory. Mercury [Hg] was quantified using 3112 B: cold vapor atomic absorption spectrophotometric [AAS] method. Levels of copper [Cu] and lead [Pb] were determined using 3030 E: nitric acid digestion and 3111 B: direct air-acetylene flame AAS method. Hg, Cu and Pb are analyzed because these metal contaminants are hazardous or toxic to the environment, which may have affected the riverine system over time. Total and fecal coliform counts were determined using 9221: multiple tube fermentation techniques.
All physicochemical parameters except streamflow are based on the guidelines by APHA, AWWA, and WEF [16]. Streamflow is based on Environmental Protection Agency's calculations [17].
All water quality parameters were compared to DENR (Order No. 2016-08) standards, except conductivity [18], turbidity [19], and streamflow [20]. Physicochemical, metal and microbial parameters were analyzed through trends and inferential statistics. The three sampling stations were analyzed due to the anthropogenic activities that occur in certain parts of the river, such as the copper mine in US and the commercial establishments in DS.
Preliminary tests showed that all parameters are homogeneous and normal (Shapiro-Wilk test) with the exception of temperature, and some of site pH, conductivity and streamflow values. The homogeneous and normal parameters were analyzed using analysis of variance and Pearson r correlation while those that are not homogeneous and normal were tested using the non-parametric counterparts. Principal component analysis was conducted to characterize the loadings of all physicochemical and biological parameters for each of the Principal Components (PCs) obtained having eigenvectors higher than one [21]. The component has significant loading on a variable when the loading is greater than 0.7.
All data were stored in Microsoft Excel and the tests were conducted at α = 0.05 using the Statistical Package for the Social Sciences (SPSS).
The results of the physicochemical analysis of the Sapangdaku River are shown in Table 1 below.
Parameter | Units | Standard* | DS | MS | US |
Physicochemical Parameters | |||||
pH | 6.5–9.0 | 7.81 | 7.84 | 7.89 | |
Temperature | ℃ | 25–31 | 23.90 | 23.8 | 22.9 |
Conductivity | mS/cm | < 1a | 0.53 | 1.85 | 0.54 |
Turbidity | NTU | < 25b | 23 | 35 | 22 |
Stream flow | m/s | 0.1–0.69c | 7.45 | 1.86 | 12.89 |
DO | mg/L | > 5 | 8.1 | 7.7 | 8.1 |
BOD | mg/L | 7 | 2.7 | 1.3 | 1.2 |
COD | mg/L | 100 | 29 | 25 | 19 |
TSS | mg/L | 80 | 15 | 20 | 233 |
TDS | mg/L | 100 | 346 | 1,232 | 360 |
Metals | |||||
Hg | mg/L | 0.002 | < 0.0001 | < 0.0001 | < 0.0001 |
Cu | mg/L | 0.02 | < 0.01 | 0.152 | 0.339 |
Pb | mg/L | 0.05 | < 0.01 | < 0.01 | < 0.01 |
Microbial Parameters | |||||
Total coliform | MPN/100mL | 10,000 | 2.8x107 | 7.0x103 | 1.1x103 |
Fecal coliform | MPN/100mL | 400 | 3.90x106 | 2.2x103 | 1.4x103 |
Notes:
*Based on DENR Order No. 2016-08 unless indicated. aEPA (2001); bMPCA (2008); cNIWA (2016). |
Water analysis of the Sapangdaku River showed that pH, DO, BOD, and COD are within the permissible range for freshwater source C waters based on DENR standards. The conductivity, turbidity, and TDS of waters at MS, as well as the TSS at the US, have values outside the maximum permissible values of 1.0 mS/cm, 25 NTU, 100 mg/L, and 80 mg/L, respectively. Temperature is low in all sites while streamflow and TDS are higher than what is acceptable.
Metal concentrations of Hg and Pb in the Sapangdaku River are within the acceptable limit. However, MS and US concentrations of Cu are greater than the limit. Total coliform counts in MS and US are within the permissible range, but DS counts go beyond such range. Fecal coliform counts in all sites are greater than the 400 MPN/100mL standards.
The trends and spatial differences in physicochemical, metal and microbial characteristics across three sites are graphed in Figure 2 below.
Assessment of Sapangdaku River registered an increasing pH from the DS to the US, but pH temperature values across study sites show no significant difference, thus are comparable. TSS increases while temperature, BOD, and COD decrease from DS to the US, and their values significantly vary across sites. Moreover, DO fluctuates but shows no significant spatial difference.
Conductivity, turbidity, TDS, and streamflow have fluctuating trend and their F-values indicate significant differences, with MS values being the highest for the three former and lowest for the latter. Furthermore, no spatial difference is observed for Hg and Pb concentrations. Cu concentration increases while total and fecal coliform counts decrease from DS to the US; all three parameters have significant differences across sites.
Results of the correlational analysis among the physicochemical, metal, and microbial parameters of the Sapangdaku River are tabulated as follows.
Parameters | Site | r-value | p-value |
conductivity and BOD | DS | 0.973* | .005 |
stream flow and TSS | DS | 0.935* | .020 |
total coliform and TDS | DS | 0.936* | .019 |
Cu and temperature | DS | -0.897* | .039 |
pH and temperature | MS | 0.922* | .026 |
pH vs TSS | MS | -0.938* | .018 |
total coliform and conductivity | MS | -0.954* | .012 |
fecal coliform and pH | MS | -0.948* | .014 |
fecal coliform and TSS | MS | 0.883* | .047 |
BOD and TDS | US | 0.903* | .036 |
Cu and temperature | US | -0.945* | .015 |
All other parameters | > .05 | ||
Note: *Significant at α = 0.05 |
Correlational analysis of physicochemical parameters showed that among the associations in the DS, only conductivity & BOD, and streamflow & TSS are significantly correlated with one another, which means that conductivity increases as BOD increases. In the MS, pH has a significant relationship with temperature and TSS. BOD and TDS are significantly correlated in the US.
Cu is significantly associated with temperature in the DS and US. Total coliform has a significant correlation with TDS and conductivity in DS and MS, respectively. Fecal coliform has a significant relationship with pH and TSS in the MS. All other parameters do not correlate with other parameters in certain sites of the river.
The results of the principal component analysis of the water quality parameters of Sapangdaku River is reflected in Table 3 as principal components and rotated principal components. The parameters pH, mercury and lead were dropped upon listwise deletion of PCA in SPSS.
Parameters | PC1 | PC2 | RPC1 | RPC2 |
DO | 0.403 | -0.401 | ||
BOD | 0.760* | 0.812* | ||
COD | 0.983* | 0.975* | ||
TSS | 0.395 | -0.859* | ||
TDS | -0.991* | 0.993* | ||
Temperature | 0.688 | 0.654 | ||
Conductivity | -0.919* | 0.925* | ||
Turbidity | -0.976* | 0.987* | ||
Stream flow | 0.811* | -0.871* | ||
Copper | -0.994* | -0.991* | ||
Total coliform | 0.810* | 0.871* | ||
Fecal coliform | 0.810* | 0.871* | ||
Eigenvalues | 5.511 | 4.725 | ||
%Cumulative variance | 36.742 | 68.239 | ||
Note: *Significant variables. |
The eigenvalues of the two principal components explain 68.239% of the variance in the dataset of the water quality parameters. The first principal component explains 36.742% of the total variance. PC1 contains negative loadings on TSS and copper, as well as positive loadings on BOD, COD, total coliform and fecal coliform. The second principal component explains 31.497% of the total variance. PC2 contains one negative loading on streamflow and positive loadings on TDS, conductivity and turbidity.
The relationship between components and variables in two components shows the representativeness and influence of the water quality parameters relative to the components and the distance from the origin. Based on Figure 3, four distinct groups are formed. Two groups have negative correlation with PC1 and PC2; these are solids (copper and TSS), and streamflow, respectively. The remaining two groups show positive correlation; these are chemico-biological aspects (COD, BOD, total coliform and fecal coliform) in PC1, and water clarity (TDS, turbidity and conductivity).
Rivers are vulnerable targets of industrial and domestic pollution and eutrophication from several commercial and industrial activities, including mining activities. Metal mining such as for Cu, Zn, and Pb, has become an international concern because of its significant environmental effect and ecological degradation that can seriously impact to the health of human beings and other organisms [22]. Metals may spill into the surrounding area of the mine pit that can alter both terrestrial and aquatic environments and flow to riverine systems that can be carried to other nearby areas [14,23]. This spillage has occurred in Sapangdaku River in the Philippines, where stored contaminated water from a mining pit has been dispersed in the riverine system in 1999 [14]. Due to this, the copper mine has strengthened their observance to global environmental standards and compliance to the country's environmental protection and enhancement [24]. The copper mine, along with several domestic communities and commercial establishments located near the river, have affected the riverine system through time. This present study assesses the current condition of Sapangdaku River and provides information how people and their activities have affected the river.
The water pH of the Sapangdaku River is described as very slightly basic. This weak alkalinity is primarily due to the natural composition of carbonate-rich soils in Cebu Island, Philippines. Carbonate compounds such as limestone increase the pH of freshwater sources and contribute to the alkaline nature of Cebu rivers [25,26]. There is no significant spatial difference in pH across the three sites, implying that there is no or very little chemical interference caused by domestic and industrial effluents to the river [27]. In the midstream, pH has a significant positive correlation with temperature but a negative correlation with TSS. This association could be since when temperature increases, the dissolution of carbonates and hydroxides in water also increases [28]. As carbonates and hydroxides are dissolved, less suspended solids are found. The relationship between pH, temperature, and TSS affects the habitability of aquatic life [29].
Sapangdaku River has cooler waters than normal Class C freshwater sources. This could be affected by the rainy weather during the sampling of water. There is a significant difference in the temperature of the three experimental sites, which could be due to the geographical location and sampling time [20]. Water temperature varies depending on local conditions, such as the upstream has cooler waters than the downstream due to altitude [30].
The conductivity of the downstream and upstream of the Sapangdaku River is within the acceptable range while TDS is within that range across all sites. The midstream registered the highest conductivity and TDS values. These higher values can be due to the limestone deposits naturally occurring in Cebu, as well as the low rate of calculated streamflow in the site. Due to limestone deposits, calcium and magnesium ions are dissociated in the water [31]. Low stream flow rate and cooler condition of the site increases conductivity and TDS, which could lead to a greater risk of eutrophication and hardness of the water in the river [32].
Like conductivity, the turbidity of the downstream and upstream of the river is within the acceptable range for warm waters. The turbidity in the midstream site is very high compared to the MPCA range, which could be associated with the quarrying activity in the upstream. The sand and gravel particles from the activity are carried from the upstream, wherein flow drastically decreases from there, thereby having more particle settlements in the midstream. Human activities like quarrying generate very increased levels of colloidal rock particles in the water [27,33]. There has been observed a significant difference in turbidity measures in the sampling sites, which could be due to the extent of use of soil resources in the river (i.e., through quarrying) and the significant difference in the flow of water in the river. The drastic increase of turbidity in the midstream signifies an environmental concern, which could impair water quality, thereby decreasing the habitability of aquatic and human life and increasing the risk of flooding [34].
The flow rate of water of the Sapangdaku River is higher than the acceptable range for rivers and streams. Observed quarrying activity, which causes erosion, have caused the high flow rate in the upstream and downstream, as well as higher TSS in the upstream area of the river, which devastates the aquatic life in the headstream and low stream [35,36]. The abundance of pollution-indicator organisms such as dragonflies and striders in the river suggests the possible polluted condition brought about by quarrying and household activities [37]. Significant differences among flow rates in the three sites could be due to the extent of human use of river soil resources, erosion, and depth.
The DO concentrations of the Sapangdaku River are within the permissible range and are not significantly different across the three sites. This means that the river has DO concentration enough for the survival of the organisms living underwater [38], and any site of the river can offer more diverse and stable ecosystems [39]. Due to these high levels of DO, the BOD and COD levels across all sites are within the DENR standard. BOD and COD results indicate that the river water has little organic pollution; this means that no large amounts of organic matter are found in the river. With this little amount of organic matter, microorganisms could not find substrates to undergo aerobic processes, therefore, oxygen levels are still controllable [40]. BOD and COD in the downstream part registered the highest concentrations, which may be attributed to the contribution of domestic wastes and industrial effluents from communities surrounding the lower part of the river [41]. BOD is significantly correlated with conductivity in the downstream; the BOD-conductivity relationship can be explained by the domestic and industrial effluents that may have carried dissolved salts in them [42]. BOD and TDS are significantly associated with the upstream, which can be attributed to the ions coming from limestone deposits and soluble metals in the mountain and mining area [43].
The Hg and Pb concentrations in all sites of the river are very low that such values are within the DENR standard. Hg and Pb at low levels suggest that the water is free from possible metal toxicity and bioaccumulation through trophic chains [44,45]. Previous Sapangdaku River study by Lok & Sakamoto [14] found high amounts of Pb but in the pipe tailings sediments and not necessarily from the river. The same river study also found out that Cu has the highest concentration among other metals as found in sediments. In the present study, Cu is found in high concentrations in the midstream and upstream beyond the permissible range. This high concentration can be attributed to the fact that a copper mine operates near the area. Cu leached from the surroundings through erosion because the landmass in the area is generally rich in copper [14]. Thus, in the present, Cu is found in large concentrations. High amounts of the metal lead to Cu toxicity and poisoning that negatively affects both aquatic animals and humans alike [46].
Total coliform counts in the midstream and upstream are acceptable, while the downstream count is unacceptable. The total counts in the downstream mean that there are large numbers of coliforms in the water that there is a high probability that other pathogenic bacteria or organisms may be present that could cause diseases [47]. Such counts may be linked to more domestic and industrial effluents found in the downstream site. Total coliform is associated with TDS in the downstream, indicating that domestic wastes may have carried soluble solids along with bacteria and other pathogens [48]. Total coliform is inversely related to conductivity in the midstream, suggesting that coliform count is still acceptable even though conductivity increases due to low flow rate and cooler conditions of the site [32].
Fecal coliform counts in all sites are beyond the standard. This means that there are large numbers of bacteria coming from the feces of people and animals. All river sites have this large number of fecal bacteria because households are living along with each side of the river. Households dump human and animal feces, decaying food wastes, and animal carcasses contributing to the high counts [12]. Of the three sites, the downstream registered the highest fecal coliform counts. More communities are found in the downstream where there are more people and have more garbage to dump. The fecal coliform count is inversely correlated with pH and directly correlated with TSS in the midstream. Seo et al. [49] obtained the same result and attributed the former to the occurrence of carbon dioxide while the latter to the inflow of non-point pollutants such as feces.
Results of the principal component analysis showed that water quality parameters that are important in understanding the current condition of Sapangdaku River. In this study, PCA was used to determine the most important factors, which contributed to the overall variability of physico-chemical and biological parameters across the sampling area. Under the first principal component, positive loading for BOD, COD, total coliform and fecal coliform may imply presence of organic pollutants from domestic sewage, animal feces and decaying animal carcasses found in the vicinity. Studies reported that "organic factor" from municipal and industrial effluents may result to positive loadings of COD and BOD [21,50]. In this present paper, however, the BOD and COD levels are within permissible values. while total coliform and fecal coliform counts are beyond the acceptable standard. Though there is evidence that the river is polluted to some extent due to the coliform counts, the acceptable BOD and COD values indicate that the riverine system can still offer habitat for life to exist. Meanwhile, positive loadings for turbidity may imply that soil erosion may have recently occurred in the area prior to data collection, and this is associated to the presence of dissolved solids [21,51,52].
The condition of the river is affected by the metal copper and suspended solids, streamflow, and water clarity. Copper and suspended solid concentrations are significantly increasing from DS to US, implying that these solids may come from soil erosion activities such as quarrying and mining. Streamflow in the middle portion of the river has the lowest rate due to the observed quarrying activity. Suspended and dissolved solid particles originating from the high flow rate (upper portion) which settled in the midstream increases turbidity and conductivity in the area. These parameters describe how soil erosion activities have affected Sapangdaku River.
Sapangdaku River in Toledo City is very weakly basic and has cooler waters than a typical Class C freshwater source. Significant fluctuations in conductivity, turbidity, streamflow, and TDS are observed across the upstream, midstream, and downstream sites of the river. DO, BOD, and COD concentrations show that the Sapangdaku River exhibits little organic pollution that keeps the level of usable oxygen at optimum for aquatic ecosystems to prosper. There are negligible amounts of Hg and Pb but high concentrations of Cu that may pose metal poisoning to aquatic life and humans. Total coliform counts are low in the midstream and upstream, while fecal coliform counts are high in all sites.
Anthropogenic activities such as mining and large-scale quarrying have affected the Sapangdaku River quality. Higher conductivity, higher turbidity, slower flow rate, and cooler conditions in the midstream, as well as the presence of more pathogenic bacteria and Cu across sites, indicate poor water quality. These conditions could be improved through educating the communities, decreasing means of erosion, and establishing strict policies and ordinances on dumping garbage and the use of river resources in the area.
Though there is poor quality, the DO, BOD, and COD levels of the river show promising results that aquatic life can still thrive in the Sapangdaku River. Future researchers could use the study findings as to baseline data for further assessments, especially on the river ecosystem. They could also use the results for interventions that the government, private sector, and communities will formulate and implement for the Sapangdaku River and other southern Cebu rivers.
The authors wish to extend their gratitude to the local government of Toledo City, especially the barangay captains and barangay health workers of Cambang-ug, Don Juan Climaco Sr., and Dumlog who helped in the conduct of the study.
The authors declare no conflict of interest.
[1] | Gorme JB, Maniquiz MC, Song P, et al. (2010) The water quality of the Pasig River in the City of Manila, Philippines: Current status, management and future recovery. Environ Eng Res 15: 173–179. |
[2] | Catchilar GC (2008) Fundamentals of Environmental Science. Mandaluyong City: National Book Store. |
[3] | Mora C, Tittensor DP, Adl S, et al. (2011) How many species are there on Earth and in the ocean? PLoS Biol 9: e1001127. |
[4] | Shiklomanov L (1993) World freshwater resources. Water in crisis: A guide to the fresh water resources. New York: Oxford University Press, 13–24. |
[5] | Gleick PH (1996) Water resources. Encyclopedia of climate and weather. New York: Oxford University Press, 2: 817–823. |
[6] | Lansing JS, Lansing P, Erazo J (1998) The value of a river. J Pol Ecol 5: 1–22. |
[7] | Jordaan JM (2009) The uses of river water and impacts. Fresh Surface Water 3: 1–10. |
[8] | Environmental Management Bureau (2014) National water quality report 2006–2013. Quezon City: Department of Environment and Natural Resources-EMB. |
[9] | World Bank (2003) Philippines – environment monitor 2003. Available from: http://documents1.worldbank.org/curated/en/144581468776089600/pdf/282970PH0Environment0monitor.pdf |
[10] | Dyer SD, Peng C, McAvoy DC, et al. (2003) The influence of untreated wastewater to aquatic communities in the Balatuin River, the Philippines. Chemosphere 52: 43–53. |
[11] | Environmental Management Bureau (2016) Annual Report for CY 2016. Quezon City: Department of Environment and Natural Resources-EMB. |
[12] | Picardal JP, Bendoy A, Calumba JR, et al. (2012) Impacts of waste disposal practices and water utilization of riverside dwellers on physico-chemical and microbiological properties of Butuanon River, Central Visayas, Philippines. CNU J High Educ Sp.: 78–100. |
[13] | Oquiñena-Paler, MKM, Ancog, R (2014) Copper, lead and zinc concentration in water, sediments and catfish (Clarias macrocephalus gunther) from Butuanon River, Metro Cebu, Philippines. IOSR J Environ Sci Toxicol Food Tech 8: 49–56. |
[14] | Lo JM, Sakamoto H. Heavy metals distribution in the surface sediments from central west coast of Cebu, Philippines. J Sediment Sco Jap 62: 31–41. |
[15] | Paringit, EC, Otadoy, RS (2017) LiDAR surveys and Flood mapping of Sapangdaku River. Quezon City: University of the Philippines Training Center for Applied Geodesy and Photogrammetry. |
[16] | American Public Health Association, American Water Works Association, & Water Environment Association (2005) Standard methods for the examination of water and wastewater (21st Edition). Washington, DC: APHA-AWWA-WEF. |
[17] | Environmental Protection Agency (2012) 5.1 Stream flow. Available from: https://archive.epa.gov/water/archive/web/html/vms51.html |
[18] | Environmental Protection Agency (2012) 5.9 Conductivity. Available from: https://archive.epa.gov/water/archive/web/html/vms59.html |
[19] | Minnesota Pollution Control Agency (2008) Turbidity: description, impact on water quality, sources, measures- A general overview. Available from: https://www.pca.state.mn.us/sites/default/files/wq-iw3-21.pdf |
[20] | National Institute of Water and Atmospheric Research (2016) Streamflow. Available from: https://niwa.co.nz/ |
[21] | Ling TY, Soo CL, Liew JJ, et al. (2017) Application of multivariate statistical analysis in evaluation of surface river water quality of a tropical river. J Chem 2017. |
[22] | Byrne P, Wood PJ, Reid I (2012) The impairment of river systems by metal mine contamination: A review including remediation options. Crit Rev Environ Sci Technol 42: 2017–2077. |
[23] | Haddaway NR, Cooke SJ, Lesser P, et al. (2019) Evidence of the impacts of metal mining and the effectiveness of mining mitigation measures on social–ecological systems in Arctic and boreal regions: a systematic map protocol. Environ Evid 8. |
[24] | Atlas Mining (2013) Safeguarding the natural balance of the environment[Internet] |
[25] | Maglangit FF, Galapate RP, Bensig, EO (2014) Physicochemical-assessment of the water quality of Buhisan River, Cebu, Philippines. Int J Res Environ Sci Tech 4: 83–87 |
[26] | Maglangit FF, Galapate RP, Bensig, EO (2014) Physicochemical-assessment of the water quality of Bulacao River, Cebu, Philippines. J Biodiv Environ Sci 5: 518–525. |
[27] | Kheira R, Boualem R (2015) Impact of quarry sand exploitation on surface water flow quality—case of El Harrach stream channel, Algeria. Desalin Water Treat 57: 21189–21200. |
[28] | Li H, Shi A, Li M, et al. (2013) Effect of pH, temperature, dissolved oxygen, and flow rate of overlying water on heavy metals release from storm sewer sediments. J Chem Article ID 434012. |
[29] | Nyanti L, Soo C, Danial-Nakhaie M, et al. (2018) Effects of water temperature and pH on total suspended solids tolerance of Malaysian native and exotic fish species. AACL Bioflux 11: 565–573. |
[30] | Regional Aquatics Monitoring Program (2018) Water quality indicators: Temperature and dissolved oxygen. Available from: http://www.ramp-alberta.org/river/water+sediment+quality/chemical/temperature+and+dissolved+oxygen.aspx#:~:text=Water%20temperature%20is%20one%20of,decreases%20as%20water%20temperature%20increases. |
[31] | State Water Resources Control Board (2004) The clean water team guidance compendium for watershed monitoring and assessment. Available from: https://www.waterboards.ca.gov/water_issues/programs/swamp/cwt_guidance.html |
[32] | Halliday, SJ, Skeffington, RA, Bowes, MJ, et al. (2008) The water quality of the River Enbourne, UK: Observation from high-frequency in a rural, lowland river system. Water 6: 150–180. |
[33] | Green JA, Pavlish JA, Merritt RG, et al. (2005) Hydraulic impacts of quarries and gravel pits. Legislative Commission on Minnesota Resources. Available from: https://files.dnr.state.mn.us/publications/waters/hdraulic-impacts-of-quarries.pdf |
[34] | Wood MS (2014) Estimating suspended sediment in rivers using acoustic Doppler meters. US Geological Survey Fact Sheet. US Geological Survey |
[35] | Simon TP (1999) Assessing the sustainability and biological integrity of water resources using fish communities. New York: CRC Press |
[36] | Armstrong DS, Parker GW, Richards TA (2004) Evaluation of streamflow requirements for habitat protection by comparison to streamflow characteristics at index streamflow-gaging stations in Southern New England. US Geological Survey |
[37] | Maine Department of Environmental Protection (2016) Dragonfly & damselfly larvae (Odonata). Available from: https://www.maine.gov/dep/water/monitoring/biomonitoring/sampling/bugs/dragonsanddamsels.html |
[38] | Mesner N, Geiger J (2005) Dissolved Oxygen. Utah State University Extension. Available from: https://extension.usu.edu/waterquality/files-ou/whats-in-your-water/do/NR_WQ_2005-16dissolvedoxygen.pdf |
[39] | Vancouver Water Resources Education Center (2020) Water quality: Temperature, pH and dissolved oxygen. Available from: https://www.cityofvancouver.us/sites/default/files/fileattachments/public_works/page/18517/water_quality_tempph_do.pdf |
[40] | Samudro G, Mangkoedihardjo S (2010) Review on BOD, COD and BOD/COD ratio: A triangle zone for toxic, biodegradable and stable levels. Int J Acad Res 2: 235–239 |
[41] | Jordão, CP, Pereira, MG, Matos, AT, et al. (2005) Influence of domestic and industrial waste discharges on water quality at Minas Gerais State, Brazil. J Braz Chem Soc 16: 241–250 |
[42] | Ogunfowokon AO, Okoh EK, Adenuga AA, et al. (2005) An assessment of the impact of point source pollution from a university sewage treatment oxidation pond on a receiving stream- A preliminary study. J App Sci 5: 36–43 |
[43] | Raisbeck MF, Riker SL, Tate CM, et al. (2008) Water Quality for Wyoming Livestock & Wildlife: A Review of the Literature pertaining to Health Effects of Inorganic Contaminants. University of Wyoming Department of Veterinary Sciences |
[44] | Rodrigues ACM, Jesus FT, Fernandes MAF, et al. (2013) Mercury toxicity to freshwater organisms: Extrapolation using species sensitivity distribution. Bull Environ Contam Toxicol, 91: 191–196 |
[45] | Paul S, Mandal A, Bhattacharjee P, et al. (2019) Evaluation of water quality and toxicity after exposure of lead nitrate in fresh water fish, major source of water pollution. Egypt J Aquat Res, 45: 345–351 |
[46] | Woody CA, O'Neal SL (2012) Effects of copper on fish and aquatic resources. The Nature Conservancy. Available from: https://www.conservationgateway.org/ConservationByGeography/NorthAmerica/UnitedStates/alaska/sw/cpa/Documents/W2013ECopperF062012.pdf |
[47] | Oram B (2020) Drinking water and other waters bacterial testing and screening. Water Research Center. Available from: https://water-research.net/index.php/water-testing/bacteria-testing/coliform-bacteria |
[48] | World Health Organization (2003) Guidelines for drinking-water quality. Geneva: WHO |
[49] | Seo M, Lee H, Kim Y (2019) Relationship between coliform bacteria and water quality factors at Weir Stations in the Nakdong River, South Korea. Water 11: 1171 |
[50] | Simeonov V, Stratis JA, Samaraetal C (2003) Assessment of the surface water quality in Northern Greece. Water Res 37: 4119–4124 |
[51] | Bilotta GS, Brazier RE (2008) Understanding the influenceof suspended solids on water quality and aquatic biota. Water Res 42: 2849–2861 |
[52] | Phung D, Huang C, Rutherford S (2015) Temporal and spatial assessment of river surface water quality using multivariate statistical techniques: A study in Can Tho City, a Mekong Deltaarea, Vietnam. Environ Monito Assess 187: 5. |
1. | Stephen Balaka Opiyo, Godwin Opinde, Sammy Letema, Spatio-seasonal variations in water quality status of Migori River in Kenya and associated household health risk implications: an application of a multidimensional water quality index approach, 2022, 1571-5124, 1, 10.1080/15715124.2022.2138409 |
Parameter | Units | Standard* | DS | MS | US |
Physicochemical Parameters | |||||
pH | 6.5–9.0 | 7.81 | 7.84 | 7.89 | |
Temperature | ℃ | 25–31 | 23.90 | 23.8 | 22.9 |
Conductivity | mS/cm | < 1a | 0.53 | 1.85 | 0.54 |
Turbidity | NTU | < 25b | 23 | 35 | 22 |
Stream flow | m/s | 0.1–0.69c | 7.45 | 1.86 | 12.89 |
DO | mg/L | > 5 | 8.1 | 7.7 | 8.1 |
BOD | mg/L | 7 | 2.7 | 1.3 | 1.2 |
COD | mg/L | 100 | 29 | 25 | 19 |
TSS | mg/L | 80 | 15 | 20 | 233 |
TDS | mg/L | 100 | 346 | 1,232 | 360 |
Metals | |||||
Hg | mg/L | 0.002 | < 0.0001 | < 0.0001 | < 0.0001 |
Cu | mg/L | 0.02 | < 0.01 | 0.152 | 0.339 |
Pb | mg/L | 0.05 | < 0.01 | < 0.01 | < 0.01 |
Microbial Parameters | |||||
Total coliform | MPN/100mL | 10,000 | 2.8x107 | 7.0x103 | 1.1x103 |
Fecal coliform | MPN/100mL | 400 | 3.90x106 | 2.2x103 | 1.4x103 |
Notes:
*Based on DENR Order No. 2016-08 unless indicated. aEPA (2001); bMPCA (2008); cNIWA (2016). |
Parameters | Site | r-value | p-value |
conductivity and BOD | DS | 0.973* | .005 |
stream flow and TSS | DS | 0.935* | .020 |
total coliform and TDS | DS | 0.936* | .019 |
Cu and temperature | DS | -0.897* | .039 |
pH and temperature | MS | 0.922* | .026 |
pH vs TSS | MS | -0.938* | .018 |
total coliform and conductivity | MS | -0.954* | .012 |
fecal coliform and pH | MS | -0.948* | .014 |
fecal coliform and TSS | MS | 0.883* | .047 |
BOD and TDS | US | 0.903* | .036 |
Cu and temperature | US | -0.945* | .015 |
All other parameters | > .05 | ||
Note: *Significant at α = 0.05 |
Parameters | PC1 | PC2 | RPC1 | RPC2 |
DO | 0.403 | -0.401 | ||
BOD | 0.760* | 0.812* | ||
COD | 0.983* | 0.975* | ||
TSS | 0.395 | -0.859* | ||
TDS | -0.991* | 0.993* | ||
Temperature | 0.688 | 0.654 | ||
Conductivity | -0.919* | 0.925* | ||
Turbidity | -0.976* | 0.987* | ||
Stream flow | 0.811* | -0.871* | ||
Copper | -0.994* | -0.991* | ||
Total coliform | 0.810* | 0.871* | ||
Fecal coliform | 0.810* | 0.871* | ||
Eigenvalues | 5.511 | 4.725 | ||
%Cumulative variance | 36.742 | 68.239 | ||
Note: *Significant variables. |
Parameter | Units | Standard* | DS | MS | US |
Physicochemical Parameters | |||||
pH | 6.5–9.0 | 7.81 | 7.84 | 7.89 | |
Temperature | ℃ | 25–31 | 23.90 | 23.8 | 22.9 |
Conductivity | mS/cm | < 1a | 0.53 | 1.85 | 0.54 |
Turbidity | NTU | < 25b | 23 | 35 | 22 |
Stream flow | m/s | 0.1–0.69c | 7.45 | 1.86 | 12.89 |
DO | mg/L | > 5 | 8.1 | 7.7 | 8.1 |
BOD | mg/L | 7 | 2.7 | 1.3 | 1.2 |
COD | mg/L | 100 | 29 | 25 | 19 |
TSS | mg/L | 80 | 15 | 20 | 233 |
TDS | mg/L | 100 | 346 | 1,232 | 360 |
Metals | |||||
Hg | mg/L | 0.002 | < 0.0001 | < 0.0001 | < 0.0001 |
Cu | mg/L | 0.02 | < 0.01 | 0.152 | 0.339 |
Pb | mg/L | 0.05 | < 0.01 | < 0.01 | < 0.01 |
Microbial Parameters | |||||
Total coliform | MPN/100mL | 10,000 | 2.8x107 | 7.0x103 | 1.1x103 |
Fecal coliform | MPN/100mL | 400 | 3.90x106 | 2.2x103 | 1.4x103 |
Notes:
*Based on DENR Order No. 2016-08 unless indicated. aEPA (2001); bMPCA (2008); cNIWA (2016). |
Parameters | Site | r-value | p-value |
conductivity and BOD | DS | 0.973* | .005 |
stream flow and TSS | DS | 0.935* | .020 |
total coliform and TDS | DS | 0.936* | .019 |
Cu and temperature | DS | -0.897* | .039 |
pH and temperature | MS | 0.922* | .026 |
pH vs TSS | MS | -0.938* | .018 |
total coliform and conductivity | MS | -0.954* | .012 |
fecal coliform and pH | MS | -0.948* | .014 |
fecal coliform and TSS | MS | 0.883* | .047 |
BOD and TDS | US | 0.903* | .036 |
Cu and temperature | US | -0.945* | .015 |
All other parameters | > .05 | ||
Note: *Significant at α = 0.05 |
Parameters | PC1 | PC2 | RPC1 | RPC2 |
DO | 0.403 | -0.401 | ||
BOD | 0.760* | 0.812* | ||
COD | 0.983* | 0.975* | ||
TSS | 0.395 | -0.859* | ||
TDS | -0.991* | 0.993* | ||
Temperature | 0.688 | 0.654 | ||
Conductivity | -0.919* | 0.925* | ||
Turbidity | -0.976* | 0.987* | ||
Stream flow | 0.811* | -0.871* | ||
Copper | -0.994* | -0.991* | ||
Total coliform | 0.810* | 0.871* | ||
Fecal coliform | 0.810* | 0.871* | ||
Eigenvalues | 5.511 | 4.725 | ||
%Cumulative variance | 36.742 | 68.239 | ||
Note: *Significant variables. |