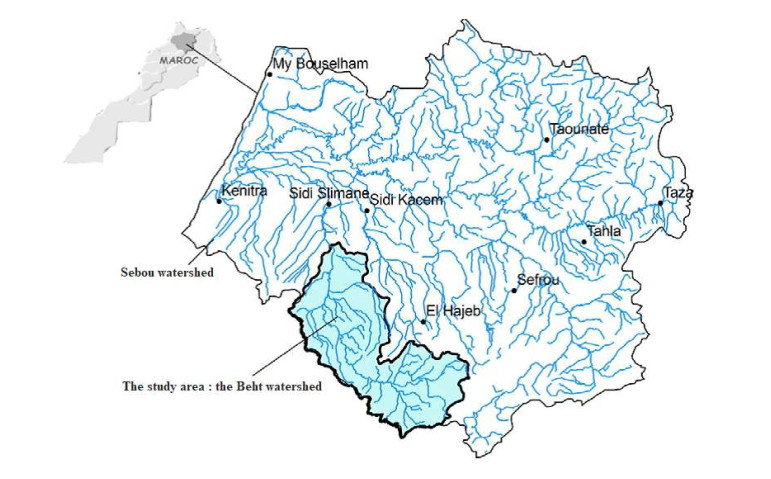
In recent years studies of nanomaterials have been explored in the field of microbiology due to the increasing evidence of antibiotic resistance. Nanomaterials could be inorganic or organic, and they may be synthesized from natural products from plant or animal origin. The therapeutic applications of nano-materials are wide, from diagnosis of disease to targeted delivery of drugs. Broad-spectrum antiviral and antimicrobial activities of nanoparticles are also well evident. The ratio of nanoparticles surface area to their volume is high and that allows them to be an advantageous vehicle of drugs in many respects. Effective uses of various materials for the synthesis of nanoparticles impart much specificity in them to meet the requirements of specific therapeutic strategies. The potential therapeutic use of nanoparticles and their mechanisms of action against infections from bacteria, fungi and viruses were the focus of this review. Further, their potential advantages, drawbacks, limitations and side effects are also included here. Researchers are characterizing the exposure pathways of nano-medicines that may cause serious toxicity to the subjects or the environment. Indeed, societal ethical issues in using nano-medicines pose a serious question to scientists beyond anything.
Citation: Ashok Chakraborty, Anil Diwan, Jayant Tatake. Prospect of nanomaterials as antimicrobial and antiviral regimen[J]. AIMS Microbiology, 2023, 9(3): 444-466. doi: 10.3934/microbiol.2023024
[1] | Emitt C. Witt III . Use of lidar point cloud data to support estimation of residual trace metals stored in mine chat piles in the Old Lead Belt of southeastern, Missouri. AIMS Environmental Science, 2016, 3(3): 509-524. doi: 10.3934/environsci.2016.3.509 |
[2] | Susmita Mukherjee, Sharanya Paul, Shreya Bhattacharjee, Somava Nath, Upasana Sharma, Sonali Paul . Bioleaching of critical metals using microalgae. AIMS Environmental Science, 2023, 10(2): 226-244. doi: 10.3934/environsci.2023013 |
[3] | Joje Mar P. Sanchez, Marchee T. Picardal, Marnan T. Libres, Hedeliza A. Pineda, Ma. Lourdes B. Paloma, Judelynn M. Librinca, Reginald Raymund A. Caturza, Sherry P. Ramayla, Ruby L. Armada, Jay P. Picardal . Characterization of a river at risk: the case of Sapangdaku River in Toledo City, Cebu, Philippines. AIMS Environmental Science, 2020, 7(6): 559-574. doi: 10.3934/environsci.2020035 |
[4] | Sandrine Chifflet, Marc Tedetti, Hana Zouch, Rania Fourati, Hatem Zaghden, Boubaker Elleuch, Marianne Quéméneur, Fatma Karray, Sami Sayadi . Dynamics of trace metals in a shallow coastal ecosystem: insights from the Gulf of Gabès (southern Mediterranean Sea). AIMS Environmental Science, 2019, 6(4): 277-297. doi: 10.3934/environsci.2019.4.277 |
[5] | Jerry R. Miller, John P. Gannon, Kyle Corcoran . Concentrations, mobility, and potential ecological risks of selected metals within compost amended, reclaimed coal mine soils, tropical South Sumatra, Indonesia. AIMS Environmental Science, 2019, 6(4): 298-325. doi: 10.3934/environsci.2019.4.298 |
[6] | Irene Mwakesi, Raphael Wahome, Daniel Ichang'i . Mining impact on communities’ livelihoods: A case study of Taita Taveta County, Kenya. AIMS Environmental Science, 2020, 7(3): 286-301. doi: 10.3934/environsci.2020018 |
[7] | Jerry R. Miller . Potential ecological impacts of trace metals on aquatic biota within the Upper Little Tennessee River Basin, North Carolina. AIMS Environmental Science, 2016, 3(3): 305-325. doi: 10.3934/environsci.2016.3.305 |
[8] | Paloma Alcorlo, Irene Lozano, Angel Baltanás . Heavy metals effects on life traits of juveniles of Procambarus clarkii. AIMS Environmental Science, 2019, 6(3): 147-166. doi: 10.3934/environsci.2019.3.147 |
[9] | Jan Zloch, Dana Adamcová, Ondřej Šindelář, Markéta Šourková, Magdalena Daria Vaverková . Testing of phytotoxicity of mining waste to determine the direction of future development. AIMS Environmental Science, 2020, 7(4): 324-334. doi: 10.3934/environsci.2020021 |
[10] | Marta Rodrigues, André B. Fortunato . Assessment of a three-dimensional baroclinic circulation model of the Tagus estuary (Portugal). AIMS Environmental Science, 2017, 4(6): 763-787. doi: 10.3934/environsci.2017.6.763 |
In recent years studies of nanomaterials have been explored in the field of microbiology due to the increasing evidence of antibiotic resistance. Nanomaterials could be inorganic or organic, and they may be synthesized from natural products from plant or animal origin. The therapeutic applications of nano-materials are wide, from diagnosis of disease to targeted delivery of drugs. Broad-spectrum antiviral and antimicrobial activities of nanoparticles are also well evident. The ratio of nanoparticles surface area to their volume is high and that allows them to be an advantageous vehicle of drugs in many respects. Effective uses of various materials for the synthesis of nanoparticles impart much specificity in them to meet the requirements of specific therapeutic strategies. The potential therapeutic use of nanoparticles and their mechanisms of action against infections from bacteria, fungi and viruses were the focus of this review. Further, their potential advantages, drawbacks, limitations and side effects are also included here. Researchers are characterizing the exposure pathways of nano-medicines that may cause serious toxicity to the subjects or the environment. Indeed, societal ethical issues in using nano-medicines pose a serious question to scientists beyond anything.
The interest of evaluating the impacts of mining and its wastes on the environment did not arise until the 1990s. This interest required, in several countries, the implementation of mining activities regulations to limit the risks of pollution and to preserve natural ecosystems. Like in other countries, the mining sector in Morocco has found itself confronted with this environmental issue. The mining regulations (law 33-13) aim to set a range of new conditions for mining, taking into account the environment parameter during all phases of exploitation and post-mine. However, the problem of old mines exploited and abandoned without rehabilitation still remains [1,2].
Mining generates negative impacts on the environment as a result of direct or indirect effects during activities and/or after the closure of the mine [3,4]. Freshwater basins are the most affected element by such activities due to the water usage for the ore treatment and mine water discharges [4]. Thus, mines are increasingly threatening the water resources on which the whole ecosystem depends. Water is then considered as a "victim of mining" [5]. Metals are omnipresent in surface and ground waters, and their concentrations are generally very low, hence comes the name "metallic trace elements (MTE)". Mineral deposits are concentrations of metallic or other mineral commodities in the Earth's crust that result from a variety of complex geologic processes. The natural weathering and erosion of a mineral deposit at the Earth's surface disperse their constituents into the waters, soils, and sediments of the surrounding environment [6,7]. Thereby, the exploitation of a deposit rich in metals generates a change in terms of the quality of the water in contact with the mining works [8]. Around the world, several previous studies have been devoted to the problem of the abandoned mines' impact on the environment [2,9,10,11,12,13,14,15,16,17]
The present work falls within the general perspective of understanding the pollutants behavior and the metallic trace elements mobility in the mining environment, through the diagnosis of the current contamination situation and its evolution over time. The aim of the work is to ensure the protection of the environment against the nuisance caused by mining activity in the study area. The haut Beht mine is located at the Beht watershed which is part of the Sebou watershed (sub-basin) (Figure 1). The Sebou River drains one of the main watersheds of Morocco in terms of water resources. However, it is subjected to a strong pollution [18,19,20,21]. The waters of Sebou have been experiencing a significant deterioration in their quality for several years, due to domestic and industrial discharges [22,23,24,25]. The Sebou watershed contains 13.3% of the country's industrial units, 7% of which are located in the Fez region, 3.2% in the Meknes region, and 3.1% in the Kenitra region [18]. In several previous studies, water pollution in the sebou basin and its sub-basin Beht has been treated, among these studies we can cite [18,19,20,21,22,23,24]. for the Sebou and [25,26,27,28]. for The Beht, but to our knowledge there is no study that has been dedicated to the evaluation of the mine water discharges quality and their impact on the environment like this work.
The purpose of this work is to evaluate the physicochemical quality of the Haut Beht mine water discharges and their metallic trace elements (MTE) load (As, Pb, Cd, Zn, and Cu) through monitoring of four stations during two analysis campaigns in 2014 and 2015. The mine water which is considered in other studies as natural mine discharges, because when the continuous pumping of the water from the mine stop in the abandoned mines, its mine water infiltrates and becomes more charged in the mine, achieving by gravity the underground waters and contaminating it by heavy metals. The mine water discharges are originally from the mine, and they are different from those coming from the mine tailing dam which is treated in several studies as the only mine' discharge. The evaluation of the mine water load in trace metal element and other analyses in this work are elaborated in the world for cases of sustainable management of mine water discharges and the reduction of their environmental impacts, or in the context of the performance monitoring of a treatment station of mine water discharges, or for post-mine monitoring and impact assessment (Table 2).
The study area is in the Beht watershed (sub-basin of the sebou watershed: The watershed of the river Beht is located northwest of Morocco and occupies the southwestern part of the Sebou basin), which is bounded on the north by the Gharb plain and the Meknes plateau, on the south by the Oum-Erbia basin, on the west by the Bouregreg watershed and on the east by the Middle Atlas, which occupies the southwestern part of the Sebou watershed in northwestern Morocco [29]. The administrative territory of the Beht watershed overlaps contains five provinces and twenty-six Rural Communes (Figure 1). The study area has a mountain character, and does not contain a generalized groundwater table. The water resources of the localized water tables are used in the form of springs or by wells in the alluvium by the local populations for the irrigation of small plots or the supply of drinking water. In addition, there are alluvial aquifers along the Beht river where small perched aquifers with low flow rates are found. The HBM mine is located on the boundary of Beht river, The main affluent of the Sebou river, and its two affluents 1 and 2 (Figures 1 and 3). The Sebou watershed covers an area of 40, 000 km2 located between the meridians 3°50' and 6°40' W and the parallels 33°and 35° N. This watershed, which includes 1/3 of Morocco's surface water resources, is drained by the Sebou river that originates in the Middle Atlas and travels about 500 km before reaching the Atlantic Ocean near Kenitra [30]. The watershed of Sebou is the richest in terms of water resources in Morocco, with water supplies of the basin amounting to more than 5 billion m3/year, and it is one of the regions with the most important potential of irrigable and irrigated lands and industries at the national level. All these activities affect the quality of waters in this basin and cause more or less significant changes to the living communities [22,24,31].
From a geological point of view, the mine studied is located in the north-eastern part of the massif of central Morocco, which is a northern zone of the western Meseta [32,33]. This zone consists essentially of a Neoproterozoic substratum (acid or intermediate lava and low flush granites) [34,35], a Paleozoic cover (Cambrian to Permian age) and sedimentary and volcanic Meso-Cenozoic cover [2,32,33,35,36]. The main Paleozoic formations of the mine are: micro-conglomeratic schist, Ordovician quartzite (Ashgill), and Black Silurian graptolite shale (Gothlandian) [37,38]; yellow tentaculite schist is associated with sandstone banks and Devonian seedy limestone formations [38] (Figure 2). The mine is located in a dislocation zone of the Smaala-Oulmes (NE-SW) major fault, which ends at the NE with several branches of the same direction and shows NNE-SSW branches [38,39,40,41]. The system of this fault is truncated further to the north by the Tafoudeit accident overlapping the Namur formations and the Visean lands [37,38,42].
The mineralogical study in different areas of the massif of central Morocco made it possible to distinguish different types of ores, as breccia pyrite brecciated ore, massive ore with pyrrhotite, pyrite milky ore, and ribbon ore with pyrrhotite and chalcopyrite. The mineral paragenesis is dominated in some areas by pyrrhotite (more than 90%) with pyrite, chalcopyrite, magnetite, and glaucodot (Co, Fe) which are all associated. On the other hand, sphalerite, galena, and arsenopyrite are present in accessory quantities [43,44,45,46,47,48]. The regional geological framework has given mineral richness to the Sebou watershed, which includes four deposits (active and abandoned) [2,49,50].
The study area does not contain a generalized groundwater table. The water resources of the localized water tables are used in the form of springs or by wells in the alluvium by the local populations for the irrigation of small plots or the supply of drinking water. In addition, there are alluvial aquifers along the Beht river where small perched aquifers with low flow rates are found. Taking into account the predominance of schistose and marl formations that form the watershed of the Beht river, it seems certain that the Groundwater availability in the Beht Basin remains very low. The existence of some auriferous rocks, with low water productivity and very limited extension, is mainly related to secondary modifications affecting the initially impermeable formations, to which are added appropriate structural forms (faults) [51].
From a climatic point of view, the mine is located in the Beht watershed, the region's climate is a Mediterranean type with oceanic influence and becomes continental inland [25]. It is manifestedby rainy winds coming from the west and decreases in precipitation away from the sea and in protected valleys like those of Beht or high Sebou before increasing rapidly on the slopes of the Rif. These influences of altitude, latitude, and exposure are combined to form a local microclimate where cold, frost, snow, and winter rains can oppose summer heat and thunderstorms [53,54]. The rains are poorly distributed throughout the year and very irregular from one year to the next. Average annual temperatures in the watershed range between 15 ℃ and 19 ℃ depending on altitude and continentality summer temperatures are high, the hottest months are July and August with average highs of 34 to 36 ℃ and the coldest months are December, January and February. The average of the minima is 3 to 7 ℃ [29]. The annual average rainfall in the basin is 600 mm on average with a maximum of 1000 mm on the heights further northward and a minimum of 300 mm [53].
The soil cover of the Beht watershed is essentially characterized by soils whose chemistry is dominated by the presence of varying amount of alkaline earth (calcium and magnesium) because of the limestone backbone of the area [55]. The lands of the Beht watershed are limestone clay-loam in nature, the upper horizons of which are relatively rich of organic matter. They are formed on the recent and sub-current alluvial deposits of the Beht river. The porosity is medium, and the compactness is quite high. Structural stability of water is precarious, and under the effect of excess water (irrigation, rain), the upper horizons become crusty (beating soil) [56].
In the context of the study of the impact of mining activity on the environment at the Sebou watershed that we chose the haut beht mine (HBM) among the four (active and abandoned) mines that include the Sebou watershed [2,49,50], and we proceeded to a physico-chemical characterization of its mine's water discharges and an assessment of their load in metallic trace elements and their potential and punctual impact on the environment, through two sampling and analysis campaigns, in 2014 and 2015 at the four discharge points of the mine's water.
The sampling stations were chosen to assess the physico-chemical quality and the trace metal load of the mine's water discharges. At the four selected stations, four water discharge samples were taken during two sampling and analysis campaigns carried out in 2014 and 2015 (Figure 3).
● Station 1: (Ex 1): the water discharge of the station 1 is located upstream of all other discharge points on the Beht river.
● Station 2: (Ex2): the water discharge of the station 2 is located downstream the station 1 (Ex1) on the Beht river.
● Station 3: (Ex3): the water discharge of the station 3 is located upstream of the discharge point of Ex4 on the same affluent of the Beht river, affluent 1.
● Station 4: (Ex4): the water discharge of the station 3 is located downstream the discharge point of Ex3 on the same affluent of the Beht river affluent 1.
The mine water discharges samples were taken in 2014 and 2015 at the four sampling stations. Sterilized polyethylene vials were used for sampling, and each sample was taken to avoid the degassing of the sample, then to do this each bottle is filled gently while minimizing the effects of turbulence. Hermetically sealed, sample vials are stored at 4 ℃ for rapid transport to the analytical laboratory in order to avoid changes in chemical composition due to degassing and photo-lytic or microbial reactions. Water discharges samples were acidified by the addition of 4% nitric acid to avoid changes in MTE concentration.
Following standardized methods (Table 1), 19 variables were monitored physico-chemically, including two in situ (pH, temperature).
TESTS | REFERENCE METHODS | TESTS | REFERENCE METHODS |
Accredited tests in chemistry | Accredited tests in chemistry | ||
pH | NF T 90-008/NMISO10523 | As | FD T 90-119 |
T ℃ | NF EN 25667 (ISO 5667)/NM 03.7.008 | As | NF EN ISO 11885 |
Sulfates | NF T 90-040/NM ISO 9280 | Al | NF EN ISO 11885 |
Pb | FD T 90-112 | Al | FD T 90-119 |
Pb | FD T 90-119 | Zn | FD T 90-112 |
Pb | NF EN ISO 11885 | Zn | NF EN ISO 11885 |
Cd | NFENISO5961 | Cu | FD T 90-112 |
Cd | NF EN ISO 11885 | Cu | NF EN ISO 11885 |
Fe | FD T 90-112 | Cr | NF EN1233 |
Fe | NF EN ISO 11885 | Cr | NF EN ISO 11885 |
Mn | NF EN ISO 11885 | Sb | NF EN ISO 11885 |
Mn | FD T 90-112 | Ni | FD T 90-119 |
Co | NM 03-7-022 | Ni | NF EN ISO 11885 |
Co | NF EN ISO 11885 | Se | NF EN ISO 11885 |
Sn | NF EN ISO 11885 | Se | Se FD T 90-119 |
Ag | NF EN ISO 11885 |
The heavy metal analyzes were performed by ICP-MS (inductively coupled plasma mass spectrometry), which is a highly sensitive technique with detection limits up to part per trillion (ppt) (ng/l), for monitoring the evolution of the metal charge of water discharges in five particular elements: Lead (Pb), Zinc (Zn), Copper (Cu), Cadmium (Cd) and Arsenic (As).
The two sampling and analysis campaigns of water discharges (C1: 2014 campaign and C2: 2015 campaign) were carried out by a state-approved laboratory. The samples were taken following the AFNOR standard NF EN 25667 (ISO 5667) (table 1).
The exploitation of deposits rich in sulfides [43,44,45,46,57] expose rocks to the action of air and water which together have a strong oxidizing power. Oxidation of pyrite, and other associated primary minerals in sulfide deposits, results in mine water characterized by significant amounts of sulfates and dissolved heavy metals. [58]. The heavy metals chosen in this study are linked to sulfides because the arsenopyrite is the main source of arsenic (As), the Chalcopyrite contains most of the copper, the Zn and Cd are in sphalerite [8,59], and since the native lead (Pb) is rare, and due to its chalcophile nature, it is associated with sulfide deposits [8,47,48].
All of the analysis' results of the eight samples of the mine water discharges collected during the 2014 and 2015 campaigns were compared with the general limit values for discharges into surface or underground water. The analysis of the results obtained made it possible to identify the pH variability, which is a feature key of acid mine drainage (AMD), as well as the elements in excess and the elements relating to the metallic load of water discharges, in particular lead (Pb); Zinc (Zn); Copper (Cu); Cadmium (Cd) and Arsenic (As).
The pH varies between 7.6 and 8.35 during C1 and between 7.6 and 8.2 during C2 (Figure 4). The upstream/downstream spatial distribution of the sampling stations shows a low variability of the pH values recorded during the C1 and C2 campaigns.
The mine water discharges have a neutral to slightly alkaline pH (7 < pH < 8.5), which indicates an absence of the acid character of the mine water, which is the main character of acid mine drainage (AMD) [60], in all the samples analyzed in C1 and C2. The variation in the pH contents of the mine water discharges between C1 and C2 is linked to several factors, including the depth of the mine, because as the water circulates deeper in the mine, its overall mineralization increases due to the geochemical background changes resulting from the advancement of mining operations. As well as the residence time of mine water in the mine and the contact time of mine water with the rocks (leaching of the bottom walls) because when the transit time of this water is sufficient, it occurs exchange of bases between the cations of the clays from the bottom of the mine and the cations contained in the water, which causes further changes in pH concentrations [61]. This seasonal variation in the mine water pH concentration can also be related to the rainfall conditions of the sampling period, because during a heavy rainy event the mine water records a supply of meteoric origin water. This water is very weakly mineralized with a rather acidic tendency (pH of rainwater between 6 and 6.5), which causes the drop of the mine water pH concentrations [62].
This neutral to basic character of the mine water can be explained by the fact that the AMD phenomenon is not encountered in all sulfide mineral mining operations, especially when mineral phases in the bedrock are able to neutralize the acidity produced [45,57]. With the presence of carbonate, the acidity produced is neutralized by the dissolution of carbonates witch greatly slow the solubilization rate of contaminating metallic trace elements. It is a neutral mining drainage (NMD) [63]. The capacity of the sulfides to produce acid is determined by the relative content of the acid-generating mineral phases and the acid-consuming phases; if the acid consuming such as calcite and bauxite are present, the resulting water can be neutral pH containing high concentrations of sulfate and metals [64]. In the geological context of the studied area, the presence of carbonate minerals such as calcite, promotes a natural neutralization in situ of the acidity of the mine water discharges by producing a NMD [65,66].
The sulfate contents vary during the C1 between 131 mg/L recorded at the station Ex 3, and 1413 mg/L recorded at the station Ex 4, and between 131 mg/L at the station Ex 1 and 1310 mg/L at the Ex 4 station during the C2 (Figure 5). The station Ex3 shows the two lowest sulfate concentrations noted in both C1 and C2 campaigns with a slight increase in C2. However, the station Ex 4 shows the two highest sulfate concentrations recorded during both C1 and C2 campaigns, with a significant decrease in C2.
All sulfate concentrations recorded at the mine water discharges during the C2 are lower than those recorded during the C1, except on the station Ex 3. The sulfate values recorded at the totality of the mine water discharges sampling stations exceed the limit value of the industrial discharges set at 500 mg/L, except on the station Ex 3, which represents lower concentration to the standards during the two analysis campaigns (Figure 5).
The high concentration of sulfate in the mine water discharges is mainly related to the exploitation of sulfide ore [66] due to the oxidation of the mineral sulfides by producing heavy metals and sulfate [67].
The variation in the sulfate contents between C1 and C2 at the Ex1 and Ex2 stations is linked to the progress of the underground mining works, because the geochemical background changes and induces the modification of the mineralization of the mine water discharges. The increase of the depth of the exploited levels induces the decrease of the sulfate contents because the high sulfate contents characterize the water circulating through shallow mining works [61,68,69,70]. The factor of the contact time of the mine water discharges with sulfide rocks at the mine (residence time in the mine) can also be a determining factor for the sulfate concentrations in the mine water discharges, because sulfates come from the oxidation of pyrites at the contact of water and air [61,71].
Furthermore, the sulfate concentration recorded in the station Ex 3, which does not exceed the industrial waste limit values during the C1 and C2 analysis campaigns, is probably related to the dilution phenomenon, since the final discharges sampled in this station are composed of the mixture of mine water used for mining and a large part of the dewatering of the deposit which has a significant water wealth. The discharges rate at this station accounts for more than the half of the mine's water discharges.
Aluminum concentrations show a very low variation during the C2. However, during the C1, the values recorded at the four measurement stations are less than 1.5 mg/L, except on the station Ex 2, which has a value of 14.1 mg/L, which exceed the limit value of the industrial discharges in the surface and underground waters setting the threshold value at 10 mg/L (Figure 6).
Aluminum is the second most abundant metal in the earth after iron [72]. The origin of aluminum in the mine water discharges can be linked to the geochemical alteration of rocks in contact with water and oxygen during mining it corresponds to total hydrolysis, which results in the release and drainage-driven elimination of minerals constituents. It should be noted that iron and aluminum oxyhydroxides are insolubilized. This alteration leads to the formation of newly formed clays, which can induce an increase in the turbidity of the mine water discharges circulating through the mine. Thus, the turbidity of the mine water discharges can be a determining factor of the high aluminum content recorded at point Ex2 during C1, because the increase of the turbidity induces an increase of the aluminum concentration in the mine water [73]. The high aluminum content at point Ex2 during C1 can also be linked to the pH of the mine waters, because the solubility of aluminum is low in waters with a pH close to neutrality between 7 and 7, 5, while the precipitation of aluminum requires a pH greater than 5 [73,74].
Also, the aluminum can be related to the natural erosion phenomena of the mountain, because it comes mainly from the mechanical training of alumino-siliceous minerals, which are easily mobilized, present in amorphous gels (allophanes type) and/or watershed clays [75].
The iron concentrations recorded at the mine water discharges during the C2 are lower than those recorded during the C1, except at the station Ex 1 (Figure 7). Like aluminum, the iron concentrations recorded during the C1 and C2 analysis campaigns are below the limit recommended by the limit values of industrial discharges in surface or underground waters fixed at 5 mg/L, except at the station Ex 2, which represents a value of 25.5 mg/L during the C1, far exceeding the limit value.
During the mining of a massive rock containing sulfide minerals, the pumping of the mine's dewatering in the surrounding lands an area that was previously saturated, which put the sulfide minerals in contact with the oxygen and the water of percolation. This induces the alteration of all readily oxidizable minerals, through the formation of sulfates and hydroxides, carbonates and other oxygenates characteristic of what is called the oxidation zone of mineral deposits. The Pyrite, which is the most widespread sulfide in the earth's crust, oxidizes to ferrous sulfate which, in the presence of free oxygen, is transformed into ferric sulfate [75]. This could explain the high concentration of Iron at the station Ex 2 because the deposit 2 is rich in pyrite [19,57,65,66,76].
The Manganese concentration recorded values indicate an irregular contamination. Indeed, they vary, at the 4 stations, between a minimum value of 0.003 mg/L recorded in the station Ex 3 during the C2 and a maximum value of 8.78 mg/L noted in the station Ex 2 during the C1, thus exceeding the limit value fixed at 1 mg/L. Seasonal variability is recorded at the station Ex 1, which has a concentration of 0.075 mg/L during the C1 and a concentration of 7.35 mg/L during the C2 (Figure 8).
Like iron and aluminum, the high concentration of Manganese at the station Ex 2 during the C1 and at the station Ex 1 during the C2 can be related to the oxidation of pyrite [19,57,76], following the percolation of the mine's water through the mining cavities of sulfide lands [75].
The remarkable decrease in the concentration of aluminum, iron and manganese at the station Ex 2, between the C1 and C2 campaigns is due to the change of the geochemical background, this is being the case because when the depth of the mine increases the water circulates deeper in the mine, and its overall mineralization changes [61,70].
The arsenic values recorded at the totality of the mine water discharges sampling stations do not exceed the limit values of industrial discharges in surface or underground waters set at 50 μg/l, except in the Ex 2 station which had a concentration above the standard during the C1. During the C1, they show significant variability from downstream to upstream of the mine, which varies between 83μg/l and 2.5μg/l at the Ex 2 and the Ex 1 stations respectively (Figure 9).
However during the C2, the variation of the arsenic contents is less important with values much lower than those recorded in the C1, and which vary between 25μg/l at the station Ex 3 and 3, 6μg/l at the Ex 1 and Ex 4 stations.
The arsenic ultimate source in the mine waste is the primary arsenopyrite in the ore concentrates. Arsenic is commonly associated with metallic mineral deposits. While arsenic is naturally mobilized from these deposits, mining and beneficiation of the deposits can significantly amplify arsenic mobilization. Once the ore has been excavated, processed, and discarded in waste rock piles and tailing, percolating rainwater can facilitate oxidation and dissolution of arsenic from the mine wastes and mine excavations. Dissolved arsenic can then be discharged into the environment with potentially toxic consequences for the downstream biota. Mining and tailings disposal promotes oxidative arsenic mobilization by increasing permeability and increasing contact between arsenic sulfides and oxygenated water. Hence, anthropogenic interference accelerates the natural processes of arsenic mobilization and dispersal into streams.
Arsenic is initially present in sulfide compounds such as arsenopyrite (FeAsS), orpiment (As2S3) or realgar (AsS) [77,78,79,80,81]. The arsenic contents in the waters vary according to the lithology crossed, the climate and the anthropic contribution [79]. And since it comes in different chemical forms (like many other elements), its speciation depends on the pH and the redox potential [82,83].
The visible decrease in the concentration of arsenic at point Ex 2 during C2, is probably linked to the decrease in the concentrations of Fe, Al and Mn in the mine water discharges of this point, because the oxyhydroxides of these elements constitute alongside clays and organic matter a significant fraction of the arsenic trapping during the weathering which plays an important role in controlling the concentration of dissolved arsenic [84,85,86,87,88,89].
The levels of lead, cadmium, copper and zinc recorded at the totality of the mine water discharges sampling stations, have a small spatial and temporal variability (Figure 10). They remain very low and do not exceed the limit values for industrial discharges set at 1000 μg/l for lead, 200 μg/l for cadmium, 3000 μg/l for copper and 5000 μg/l for zinc.
In waters, lead (Pb) has a strong affinity for sedimentary particles including clays, oxy-hydroxides of iron and manganese, sulfides and organic matter [90]. It can also be associated with carbonates when the medium is poor in organic matter and in oxy-hydroxides of Fe or Mn [91]. While zinc (Zn) is in ionic form, it is complexed by organic ligands (fulvic and humic acids) or even associated with inorganic colloids. In waters with pH below 8, the concentrations of zinc in the cationic form (Zn2 +) are greater, while the neutral species ZnCO3 is predominant in waters with pH above 8 [92]. Furthermore, the zinc is complexed with sulfates in waters with an acid pH and can precipitate in the form of sulfated salts under extreme acid conditions [93,94]. For the Cadmium (Cd) in waters and pH value below 8, it is present in the dissolved state as Cd2 + form, preferentially complexed by humic substances [95]. And in an anoxic environment, it precipitates with S2- even at very low concentrations [24].
In waters the copper (Cu) is mainly found in divalent form Cu (II), while the monovalent form Cu (I) is present only at extremely low concentrations since it reacts to form metallic copper and Cu (II) ions. Cu (I) can be produced under reducing conditions and the majority of the compounds formed are insoluble [96,97].
The Copper in complexed form is associated with inorganic and organic ligands. This complexation of Cu by organic ligands strongly conditions its bioavailability, because the organically complexed copper is very stable [98]. The metallic cations (Pb, Zn, Cu and Cd) generally show low concentrations in the waters of mining lakes when the pH is alkaline [99]. This is the case of the HBM mine water discharges, which is located in limestone soils [38,55] where acidic waters are quickly neutralized by carbonates, and where most metals become insoluble and precipitate [100].
In morocco, currently there are active mines producing a variety of mineralization processes, and there have been many closed and abandoned mines since the 1970s. Recently, some studies have been interested in the environmental impact of abandoned mines and sites as well as the recovery of their waste. Let us cite those of [101] and [102], on the Kettara mine and [103] on the Sidi Bou Othmane mine in the Jebilet; we can also refer to those carried out in Haute-Moulouya [104,105,106,107]., in central Morocco on the Tighza mine [108], on the Mohammedia salt mine [109] and on the Jerrada mine [110]. The studies on zones near the mining centers of Aouli and Mibladan in Haut Moulouya have shown a negative impact of mining activities, especially the contamination of surface water, sediments, soil and plants [63,106,111,112], as well as pollution by heavy metals of surface water of Moulouya river [100,113].
The evaluation of the physico-chemical quality and the MTE load of the mine water discharges is an inseparable part of the evaluation of the impact of mining on the environment, because it can be indicative of the origin of any metal detected in excess in surface water, groundwater or sediments surrounding mines. However in most of the environmental impact studies the mine water discharges are considered as natural waters discharges from the mine, however the drainage of this water through the mine cavities can induce their load in MTE., which can have an impact on the environment once this mine water is discharged or infiltrated into the underground water.
Average MTE concentrations (µg/L) | Industrial site | Study area | Exploited substance | Reference | |||||
Pb | Cd | As | Cu | Zn | |||||
Mine water discharges | 23, 17 | 1, 4 | 23, 87 | 53, 81 | 131, 67 | HBM | Sebou watershed | n. d | This Work |
Mine lakes | 27, 5 | n. d | 92, 825 | n. d | n. d | Zeïda Mine | HauteMoulouya | Pb | [111] |
drainage water | n. d | n. d | n. d | 58000 | 45000 | Kettara Mine | Kettara River Jebilet | Fe-S | [102,116,117] |
Mine lakes | 31, 186 | n. d | 111, 202 | n. d | n. d | Zeïda Mine | Haute Moulouya | Pb | [63] |
Leachate of mine tailing | 306, 875 | 9, 125 | 763 | 100686 | 8597 | Kettara Mine | Kettara RiverJebilet | Fe-S | [115] |
n. d.: Undetermined value |
In Morocco, in the majority of the impact of mining on the environment evaluation studies, the physico-chemical characterization and the evaluation of the contamination in MTE are focused on the evaluation of the water quality and sediments surrounding mining sites. In such studies, the designation of mine discharges is attributed to all liquid or solid mine discharges (spoil tip; dumps; ore processing residues; water from quarry lakes and infiltration at the foot of the tailings dam). As an example of the studies characterizing the quality of solid discharges from the mine or the ore processing plant, we can cite the work on the Tourtit and Ichoumellal mines [2], the Zeïda, Mibladen and Aouli mines [106,113], the Sidi Bou Othmane mine [103], the Kettara mine [101,102,114,115].
Since we are interested in the HBM mine water discharges, in our study the concentrations of metallic trace elements evaluated in this study were compared with some national studies (Table 2), which are concerned with the evaluation of metallic trace elements in mining areas and their concentrations in mine liquid discharges [63,102,111,115,116,117]:
The origin of the waters and their course define their hydro-chemical character and, hence the difference in MTE contents in mine liquid discharges compared to the levels in the HBM mine water discharges.
In the case of the Zeïda mine, water is sampled at the level of the mining lakes, which is located at the foot of the spoil tip and the tailings dam. The arsenic and lead concentrations are higher than the levels recorded in HBM's mine water discharges. These discharges have a common character with the waters of the mining lakes of Zeïda which is the neutral pH to slightly alkaline influencing the solubility of the MTE due to the fact that the metallic trace element (Pb, Zn, Cd, Cu, ...) show high concentrations in acid mining lakes, while the MTE (As and Se) are generally at high concentrations in alkaline mining lakes [63,99].
For the drainage water from the kettara mine, samples were taken from various pits collecting runoff water of the tailings pond and runoff water of the waste rock [102,116,117]. These drainage waters have much higher copper and zinc contents than those recorded in the HBM mine water.
The leachate of the kettara mine tailings are obtained by mixing 150 g of tailings with 300 ml of distilled water (Ratio 1/2), with permanent agitation, and after a week, the filtration was carried out, then the measurements of the concentrations of MTE in the leachate water, these analyses show that the As and Pb contents are higher at the top of the pile of mining residues, which can be explained by the acidic pH on the surface of the pile of mining residues in direct contact with the oxygen. The values recorded in As, Pb, Cu, Cd and Zn at the leachate of the tailings of the kettara mine are much higher than those recorded at the HBM's water discharges.
The MTE concentrations in the HBM water discharges are the lowest compared to other liquid discharges from other mines, something which can be linked to several factors, including: the geochemical background of the mine, the waters contact time with the rocks, the nature of the residues that the water percolates through and finally the pH of the water which plays an important role in the degree of passage of the MTE in the aqueous phase.
The concentrations of metallic trace elements evaluated in this study were compared with some international studies (Table 3), which are concerned with the evaluation of metallic trace elements in mining areas and their concentrations in mine water discharges [118,119,120].
Average MTE concentrations (µg/L) | Industrial site | Study area | Exploited substance | Reference | ||||||
Pb | Cd | As | Cu | Zn | ||||||
Morocco | Ex1 (C1) | 61 | 1, 1 | 2, 5 | 4, 2 | 190 | HBM | Sebou watershed | n.d | This work |
Ex1 (C2) | 5, 6 | 0, 5 | 3, 6 | 2 | 115 | |||||
Ex2 (C1) | 72 | 8, 3 | 83 | 406 | 528 | |||||
Ex2 (C2) | 1, 7 | 0, 2 | 9, 3 | 1, 4 | 2, 2 | |||||
Ex3 (C1) | 28 | 0, 3 | 48 | 3, 3 | 60 | |||||
Ex3 (C2) | 1, 7 | 0, 2 | 25 | 1, 4 | 2, 2 | |||||
Ex4 (C1) | 9 | 0, 4 | 16 | 8, 8 | 143 | |||||
Ex4 (C2) | 6, 4 | 0, 2 | 3, 6 | 3, 4 | 13 | |||||
Average | 23, 17 | 1, 4 | 23, 87 | 53, 81 | 131, 67 | |||||
France | 29 | n. d. | 2, 14 | 5 | 9, 28 | Escarro mine | Languedoc RoussillonTêt watershed | CaF2 | [119] | |
11, 12 | 2, 7 | n. d. | n. d. | 4, 21 | Malines mine | Saint-Laurent-le-MinierHérault watershed | Pb, ZnS, PbS, Zn | [118] | ||
England | 10, 2 | 0, 84 | n. d. | 0, 56 | 160, 2 | Soughs mine | DerbyshireBugsworth watershed | Pb | [118] | |
Norway | n. d. | 12800 | 280 | 574000 | 5640000 | Killingdal mine | Sør-TrøndelagGaula watershed | Cu Zn S | [120] | |
n. d.: Undetermined value |
The average concentration of lead (Pb) recorded in the HBM water discharges is lower than the one noted in the mine water discharges of the abandoned Escarro mine in France, which was exploited between 1960 and 1991, according to the environmental impact study of the Escaro's mine developed in 1983 [118]. In terms of lead, the average concentration in the HBM mine's water is in second class after that of the Escaro mine, then in third class that of the Malines mine in France, and in the last class that of the Soughs mine in England.
For the Cadmium (Cd), the average concentration recorded in the mine water discharges of the HBM is in third class after that of the Killingdal mine in Norway, and that of the Malines mine in France, and in the last class that of the Soughs mine in England. In terms of Arsenic (As), the average concentration in the HBM water discharges is in second class after that of the Killingdal mine in Norway, and in the last class that of the Escarro mine in France.
The average concentration of copper (Cu) in the HBM water discharges is in second class after that of the Killingdal mine in Norway, then in third class that of the Escarro mine in France, then in the last class that of the Soughs mine in England. And for the Zinc (Zn), the average concentration recorded in the HBM water discharges is in third class after that of the Killingdal mine in Norway, and that of the Soughs mine in England. In fourth class that of the Escarro mine in France then that of the Malines mine in France.
It should be noted that the physicochemical characterizations of mine waters compared to the HBM are developed as part of studies aimed at the sustainable management of mine water discharges and the reduction of their environmental impacts. For the rest of the mines, it is in the context of an environmental impact study for the continuation of exploitation for the Escarro mine, and in the context of the performance monitoring of the treatment station of mine water discharges for the Malines mine, and post-mine monitoring and impact assessment for the Soughs and the Killingdal mines. The differences among the average concentrations of MTE contained in the mines waters show the importance of the lithology of the host rock, rather than the mineralogy of the ore, for the quality of the mine water [120].
The oxidation of sulfide minerals to release heavy metals, sulfate and acid is the fundamental reaction characterizing acid mine drainage [60,121,122,123]. However, the quality of mines waters can be adversely affected by other parameters such as the kinetic factor because of the relatively slow rate of dissolution and oxidation of sulfide minerals compared to the rapid flux of limestone groundwater through the mine conduits [38,55]. And the solubility of heavy metals that is suppressed by the high alkalinity of water, as well as other parameters that may negatively influence the global quality of mine water discharges such as the salinity, the traces of explosives based on nitrogen oxidized to nitrates and the organic parameters [120].
The mine water discharges of the HBM are classified as one of the least charged mine water in terms of MTE compared to other mines (Table 3).
The physicochemical characterization of the mine water and the evaluation of their load in metallic trace elements (MTE) showed a small variability in time and space between the four stations sampled, and absenteeism of the acidic nature of mine's water. The majority of the analyzed mine waters presents important concentrations of sulfate. During the C1 at the point Ex2 theirs is contamination of Iron, Aluminum, Manganese and Arsenic. However, the concentrations of Pb, Cd, Zn and Cu elements remain conform and very low compared to the limit of standards.
The monitoring of the overtake elements made it possible to identify the degree of contamination of the mine's water discharges, and to note an improvement in time in the mine water discharges quality. This variation in element concentrations between the C1 and C2 campaigns is due to the change of the geochemical background, because with the progress of the mining operations, the geochemical background changes, influencing the hydro-chemical composition of the percolated mine water through the walls of the mine before its rise to the surface of the ground, forming the final discharges.
The mine water discharges of the HBM are classified as one of the least charged mine water in terms of MTE concentration compared to other national liquid mine discharges and to other mine waters worldwide.
The evaluation of the quality of the discharges of mine water elaborated in this study for the first time, to our knowledge, at the level of the beht basin, sub-basin of Sebou, allowed to identify the quality of the discharges of mine water and their load in MTE in order to evaluate the impact of these discharges on the environment. Thus the evaluation of the mining contribution in the modification of the quality of the environment surrounding the Haut beht mine.
The mine water remains a reservoir of contamination able to drop in the aqueous phase other elements by the phenomenon of leaching and dredging in the wet phase. Therefore it presents a potential toxicological risk in the absence of specific means of sustainable management aimed at safeguarding the surrounding environment of the mine and mitigating the potential impact of the mine water discharges into the environment.
Despite the absence of contamination of the HBM water discharges, their pH-dependent nature draws attention to the possibility of an MTE loading of the sediments and the waters surrounding the mine. The potential impacts of the mine's water discharges on the quality of groundwater, surface water, and sediments will be the subject of other ongoing works.
The diagnosis elaborated through the analysis and the quantification of the different parameters and their impacts, allowed to draw up a first report on the variability of the mine's waters discharges quality and their potential impacts on the environment.
Thus, the monitoring of environmental indicators may be essential for all industrial activity to limit their potential impacts on the environment.
This research did not receive any specific grant from funding agencies in the public, commercial, or not-for-profit sectors.
The authors declares that there is no conflict of interests regarding the publication of this paper.
[1] |
Sethi S, Murphy TF (2001) Bacterial infection in chronic obstructive pulmonary disease in 2000: a state-of-the-art review. Clin Microbiol Rev 14: 336-363. https://doi.org/10.1128/CMR.14.2.336-363.2001 ![]() |
[2] |
Durand ML, Calderwood SB, Weber DJ, et al. (1993) Acute bacterial meningitis in adults-A review of 493 episodes. N Engl J Med 328: 21-28. https://doi.org/10.1056/NEJM199301073280104 ![]() |
[3] |
Lara HH, Ayala-Nu~nez NV, Ixtepan-Turrent L, et al. (2010) Mode of antiviral action of silver nanoparticles against HIV-1. J Nanobiotechno 8: 1-10. https://doi.org/10.1186/1477-3155-8-1 ![]() |
[4] | Singh SR, Krishnamurthy NB, Mathew BB (2014) A review on recent diseases caused by microbes. J Appl Environ Microbiol 2: 106-115. |
[5] |
Laxminarayan R, Duse A, Wattal C, et al. (2013) Antibiotic resistance—the need for global solutions. Lancet Infect Dis 13: 1057-1098. https://doi.org/10.1016/S1473-3099(13)70318-9 ![]() |
[6] |
Friedman H, Newton C, Klein TW (2003) Microbial infections, immunomodulation, and drugs of abuse. Clin Microbiol Rev 16: 209-219. https://doi.org/10.1128/CMR.16.2.209-219.2003 ![]() |
[7] | Atolani O, Baker MT, Adeyemi OS, et al. (2020) Covid-19: critical discussion on the applications and implications of chemicals in sanitizers and disinfectants. EXCLI J 19: 785-799. |
[8] |
Gurunathan S, Han JW, Kwon DN, et al. (2014) Enhanced antibacterial and antibiofilm activities of silver nanoparticles against Gram-negative and Gram-positive bacteria. Nanoscale Res Lett 9: 1-17. https://doi.org/10.1186/1556-276X-9-373 ![]() |
[9] | Yien L, Zin NM, Sarwar A, et al. (2012) Antifungal activity of chitosan nanoparticles and correlation with their physical properties. Int J Biomater 632698. https://doi.org/10.1155/2012/632698 |
[10] |
Sobhani Z, Samani SM, Montaseri H, et al. (2017) Nanoparticles of chitosan loaded ciprofloxacin: fabrication and antimicrobial activity. Adv Pharmaceut Bull 7: 427. https://doi.org/10.15171/apb.2017.051 ![]() |
[11] |
Rai A, Prabhune A, Perry CC (2010) Antibiotic mediated synthesis of gold nanoparticles with potent antimicrobial activity and their application in antimicrobial coatings. J Mater Chem 20: 6789-6798. https://doi.org/10.1039/c0jm00817f ![]() |
[12] |
Alshammari F, Alshammari B, Moin A, et al. (2021) Ceftriaxone mediated synthesized gold nanoparticles: A nano-therapeutic tool to target bacterial resistance. Pharmaceutics 13: 1896. https://doi.org/10.3390/pharmaceutics13111896 ![]() |
[13] |
Cepas V, López Y, Gabasa Y, et al. (2019) Inhibition of bacterial and fungal biofilm formation by 675 extracts from microalgae and cyanobacteria. Antibiotics (Basel) 8: 77. https://doi.org/10.3390/antibiotics8020077 ![]() |
[14] |
Cremonini E, Zonaro E, Donini M, et al. (2016) Biogenic selenium nanoparticles: characterization, antimicrobial activity and effects on human dendritic cells and fibroblasts. Microb Biotechnol 9: 758-771. https://doi.org/10.1111/1751-7915.12374 ![]() |
[15] | Haghighi F, Roudba Mohammadir S, Mohammadi P, et al. (2013) Antifungal acitivity of TiO2 nanoparticles and EDTA on Candida abicans Biofilms. Infect Epidemiol Med 1: 133-138. |
[16] |
Singh P, Singh D, Sa P, et al. (2021) Insights from nanotechnology in COVID-19: prevention, detection, therapy and immunomodulation. Nanomedicine (Lond) 16: 1219-1235. https://doi.org/10.2217/nnm-2021-0004 ![]() |
[17] |
Singh CK, Sodhi KK (2023) The emerging significance of nanomedicine-based approaches to fighting COVID-19 variants of concern: A perspective on the nanotechnology's role in COVID-19 diagnosis and treatment. Front Nanotechnol 4: 1084033. https://doi.org/10.3389/fnano.2022.1084033 ![]() |
[18] |
Yang D (2021) Application of nanotechnology in the COVID-19 pandemic. Int J Nanomedicine 16: 623-649. https://doi.org/10.2147/IJN.S296383 ![]() |
[19] |
Diwan A, Chakraborty A, Vijetha Chiniga V, et al. (2022) Dual effects of NV-CoV-2 biomimetic polymer: An antiviral regimen against COVID-19. PLOS One 17: e0278963. https://doi.org/10.1371/journal.pone.0278963 ![]() |
[20] |
Chakraborty A, Diwan A, Arora V, et al. (2022) Mechanism of antiviral activities of nanoviricide's platform technology based biopolymer (NV-CoV-2). AIMS Public Health 9: 415-422. https://doi.org/10.3934/publichealth.2022028 ![]() |
[21] |
Mubeen B, Ansar AN, Rasool R, et al. (2021) Nanotechnology as a novel approach in combating microbes providing an alternative to antibiotics. Antibiotics (Basel) 10: 1473. https://doi.org/10.3390/antibiotics10121473 ![]() |
[22] |
Hung YP, Chen YF, Tsai PJ, et al. (2021) Advances in the application of nanomaterials as treatments for bacterial infectious diseases. Pharmaceutics 13: 1913. https://doi.org/10.3390/pharmaceutics13111913 ![]() |
[23] | Ozdal M, Gurkok S (2022) Recent advances in nanoparticles as antibacterial agent. ADMET DMPK 10: 115-129. https://doi.org/10.5599/admet.1172 |
[24] |
Hussain FS, Abro NQ, Ahmed N, et al. (2022) Nano antivirals: A comprehensive review. Front Nanotechnol 4: 1064615. https://doi.org/10.3389/fnano.2022.1064615 ![]() |
[25] | Nami S, Aghebati-Maleki A, Aghebati-Maleki L (2021) Current applications and prospects of nanoparticles for antifungal drug delivery. EXCLI J 20: 562-584. |
[26] |
Khan I, Saeed K, Khan I (2017) Nanoparticles: properties, applications and toxicities. Arab J Chem 12: 908-931. https://doi.org/10.1016/j.arabjc.2017.05.011 ![]() |
[27] | Song X, Bayati P, Gupta M, et al. (2021) Fracture of magnesium matrix nanocomposites-a review. Int J Lightweight Mater Manufact 4: 67-98. https://doi.org/10.1016/j.ijlmm.2020.07.002 |
[28] |
Vert M, Doi Y, Hellwich KH, et al. (2012) Terminology for biorelated polymers and applications (IUPAC Recommendations 2012). Pure Appl Chem 84: 377-410. https://doi.org/10.1515/amma-2016-0032 ![]() |
[29] |
Von Nussbaum F, Brands M, Hinzen B, et al. (2006) Antibacterial natural products in medicinal chemistry—Exodus or revival?. Angew Chem Int Ed 45: 5072-5129. https://doi.org/10.1002/anie.200600350 ![]() |
[30] |
Akhtar M, Swamy MK, Umar A, et al. (2015) Biosynthesis and characterization of silver nanoparticles from methanol leaf extract of Cassia didymobotyra and assessment of their antioxidant and antibacterial activities. J Nanosci Nanotechnol 15: 9818-9823. https://doi.org/10.1166/jnn.2015.10966 ![]() |
[31] |
Fröhlich E, Salar-Behzadi S (2014) Toxicological assessment of inhaled nanoparticles: Role of in vivo, ex vivo, in vitro, and in silico studies. Int J Mol Sci 15: 4795-4822. https://doi.org/10.3390/ijms15034795 ![]() |
[32] |
Qiu Y, Xu D, Sui G, et al. (2020) Gentamicin decorated phosphatidylcholine-chitosan nanoparticles against biofilms and intracellular bacteria. Int J Biol Macromol 156: 640-647. https://doi.org/10.1016/j.ijbiomac.2020.04.090 ![]() |
[33] |
Evangelista TF, Andrade GR, Nascimento KN, et al. (2020) Supramolecular polyelectrolyte complexes based on cyclodextrin-grafted chitosan and carrageenan for controlled drug release. Carbohydr Polym 245: 116592. https://doi.org/10.1016/j.carbpol.2020.116592 ![]() |
[34] |
Walvekar P, Gannimani R, Salih M, et al. (2019) Self-assembled oleylamine grafted hyaluronic acid polymersomes for delivery of vancomycin against methicillin resistant Staphylococcus aureus (MRSA). Colloids Surf B Biointerfaces 182: 110388. https://doi.org/10.1016/j.colsurfb.2019.110388 ![]() |
[35] |
Ejaz S, Ihsan A, Noor T, et al. (2020) Mannose functionalized chitosan nanosystems for enhanced antimicrobial activity against multidrug resistant pathogens. Polym Test 91: 106814. https://doi.org/10.1016/j.polymertesting.2020.106814 ![]() |
[36] |
Ucak S, Sudagidan M, Borsa BA, et al. (2020) Inhibitory effects of aptamer targeted teicoplanin encapsulated PLGA nanoparticles for Staphylococcus aureus strains. World J Microbiol Biotechnol 36: 69. https://doi.org/10.1007/s11274-020-02845-y ![]() |
[37] |
Vrouvaki I, Koutra E, Kornaros M, et al. (2020) Polymeric nanoparticles of pistacia lentiscus var. chia essential oil for cutaneous applications. Pharmaceutics 12: 353. https://doi.org/10.3390/pharmaceutics12040353 ![]() |
[38] |
Gherasim O, Grumezescu AM, Grumezescu V, et al. (2020) Bioactive surfaces of polylactide and silver nanoparticles for the prevention of microbial contamination. Materials 13: 768. https://doi.org/10.3390/ma13030768 ![]() |
[39] |
Grumezescu AM, Stoica AE, Dima-Balcescu MS, et al. (2019) Electrospun polyethylene terephthalate nanofibers loaded with silver nanoparticles: novel approach in anti-infective therapy. J Clin Med 8: 1039. https://doi.org/10.3390/jcm8071039 ![]() |
[40] | Khandelwal N, Kaur G, Kumara N, et al. (2014) Application of silver nanoparticles in viral inhibition: A new hope for antivirals. Dig J Nanomater Biostruct 9: 175-186. https://nanogo.co.uk/wp-content/uploads/2021/12/application-of-nanosilver.pdf |
[41] |
Alamdaran M, Movahedi B, Mohabatkar H, et al. (2018) In-vitro study of the novel nanocarrier of chitosan-based nanoparticles conjugated HIV-1 P24 protein-derived peptides. J Mol Liq 265: 243-250. https://doi.org/10.1016/j.molliq.2018.05.137 ![]() |
[42] |
Belgamwar AV, Khan SA, Yeole PG (2019) Intranasal dolutegravir sodium loaded nanoparticles of hydroxypropyl-beta-cyclodextrin for brain delivery in Neuro-AIDS. J Drug Deliv Sci Technol 52: 1008-1020. https://doi.org/10.1016/j.jddst.2019.06.014 ![]() |
[43] | Costa AF, Araujo DE, Cabral MS, et al. (2018) Development, characterization, and in vitro-in vivo evaluation of polymeric nanoparticles containing miconazole and farnesol for treatment of vulvovaginal candidiasis. Med Mycol 7: 52-62. https://doi.org/10.1093/mmy/myx155 |
[44] | Reddy YC (2018) Formulation and evaluation of chitosan nanoparticles for improved efficacy of itraconazole antifungal drug. Asian J Pharm Clin Res 11: 147-152. https://doi.org/10.22159/ajpcr.2018.v11s4.31723 |
[45] |
Sombra FM, Richter AR, De Araújo AR, et al. (2020) Development of amphotericin B-loaded propionate Sterculia striata polysaccharide nanocarrier. Int J Biol Macromol 146: 1133-1141. https://doi.org/10.1016/j.ijbiomac.2019.10.053 ![]() |
[46] |
Real D, Hoffmann S, Leonardi D, et al. (2018) Chitosan-based nanodelivery systems applied to the development of novel triclabendazole formulations. PLOS One 13: e0207625. https://doi.org/10.1371/journal.pone.0207625 ![]() |
[47] |
Durak S, Arasoglu T, Ates SC, et al. (2020) Enhanced antibacterial and antiparasitic activity of multifunctional polymeric nanoparticles. Nanotechnology 31: 175705. https://doi.org/10.1088/1361-6528/ab6ab9 ![]() |
[48] |
Binder U, Aigner M, Risslegger B, et al. (2019) Minimal Inhibitory concentration (mic)-phenomena in candida albicans and their impact on the diagnosis of antifungal resistance. J Fungi (Basel) 5: 83. https://doi.org/10.3390/jof5030083 ![]() |
[49] |
Kesharwani P, Fatima M, Singh V, et al. (2022) Itraconazole and difluorinated-curcumin containing chitosan nanoparticle loaded hydrogel for amelioration of onychomycosis. Biomimetics (Basel) 7: 206. https://doi.org/10.3390/biomimetics7040206 ![]() |
[50] |
Butani D, Yewale C, Misra A (2016) Topical amphotericin B solid lipid nanoparticles: Design and development. Colloids Surf B 139: 17-24. https://doi.org/10.1016/j.colsurfb.2015.07.032 ![]() |
[51] |
Souto E, Wissing S, Barbosa C, et al. (2004) Development of a controlled release formulation based on SLN and NLC for topical clotrimazole delivery. Int J Pharm 278: 71-7. https://doi.org/10.1016/j.ijpharm.2004.02.032 ![]() |
[52] |
Cassano R, Ferrarelli T, Mauro MV, et al. (2016) Preparation, characterization and in vitro activities evaluation of solid lipid nanoparticles based on PEG-40 stearate for antifungal drugs vaginal delivery. Drug Deliv 23: 1037-46. https://doi.org/10.3109/10717544.2014.932862 ![]() |
[53] |
Sanna V, Gavini E, Cossu M, et al. (2007) Solid lipid nanoparticles (SLN) as carriers for the topical delivery of econazole nitrate: In-vitro characterization, ex-vivo and in-vivo studies. J Pharm Pharmacol 59: 1057-64. https://doi.org/10.1211/jpp.59.8.0002 ![]() |
[54] |
Bhalekar MR, Pokharkar V, Madgulkar A, et al. (2009) Preparation and evaluation of miconazole nitrate-loaded solid lipid nanoparticles for topical delivery. AAPS PharmSciTech 10: 289-96. https://doi.org/10.1208/s12249-009-9199-0 ![]() |
[55] |
Jain S, Jain S, Khare P, et al. (2010) Design and development of solid lipid nanoparticles for topical delivery of an anti-fungal agent. Drug Deliv 17: 443-451. https://doi.org/10.3109/10717544.2010.483252 ![]() |
[56] |
Kenechukwu FC, Attama AA, Ibezim EC (2017) Novel solidified reverse micellar solution-based mucoadhesive nano lipid gels encapsulating miconazole nitrate-loaded nanoparticles for improved treatment of oropharyngeal candidiasis. J Microencapsul 34: 592-609. https://doi.org/10.1080/02652048.2017.1370029 ![]() |
[57] |
Mahato R, Tai W, Cheng K (2011) Prodrugs for improving tumor targetability and efficiency. Adv Drug Deliv Rev 63: 659-70. https://doi.org/10.1016/j.addr.2011.02.002 ![]() |
[58] |
Kumar R, Sinha VR (2016) Solid lipid nanoparticle: An efficient carrier for improved ocular permeation of voriconazole. Drug Dev Ind Pharm 42: 1956-67. https://doi.org/10.1080/03639045.2016.1185437 ![]() |
[59] |
Füredi P, Pápay ZE, Kovács K, et al. (2017) Development and characterization of the voriconazole loaded lipid-based nanoparticles. J Pharm Biomed Anal 132: 184-9. https://doi.org/10.1016/j.jpba.2016.09.047 ![]() |
[60] |
El-Housiny S, Shams Eldeen MA, El-Attar YA, et al. (2018) Fluconazole-loaded solid lipid nanoparticles topical gel for treatment of pityriasis versicolor: formulation and clinical study. Drug Deliv 25: 78-90. https://doi.org/10.1080/10717544.2017.1413444 ![]() |
[61] |
Anurak L, Chansiri G, Peankit D, et al. (2011) Griseofulvin solid lipid nanoparticles based on microemulsion technique. Adv Mater Res 197–198: 47-50. https://doi.org/10.4028/www.scientific.net/AMR.197-198.47 ![]() |
[62] |
Ahmad A, Wei Y, Syed F, et al. (2016) Amphotericin B-conjugated biogenic silver nanoparticles as an innovative strategy for fungal infections. Microb Pathol 99: 271-81. https://doi.org/10.1016/j.micpath.2016.08.031 ![]() |
[63] |
Tutaj K, Szlazak R, Szalapata K, et al. (2016) Amphotericin B-silver hybrid nanoparticles: Synthesis, properties and antifungal activity. Nanomedicine 12: 1095-103. https://doi.org/10.1016/j.nano.2015.12.378 ![]() |
[64] |
Hussain MA, Ahmed D, Anwar A, et al. (2019) Combination therapy of clinically approved antifungal drugs is enhanced by conjugation with silver nanoparticles. Int Microbiol 22: 239-46. https://doi.org/10.1007/s10123-018-00043-3 ![]() |
[65] |
Mussin JE, Roldán MV, Rojas F, et al. (2019) Antifungal activity of silver nanoparticles in combination with ketoconazole against Malassezia furfur. AMB Express 9: 131. https://doi.org/10.1186/s13568-019-0857-7 ![]() |
[66] |
Souza AC, Nascimento AL, de Vasconcelos NM, et al. (2015) Activity and in vivo tracking of Amphotericin B loaded PLGA nanoparticles. Eur J Med Chem 95: 267-276. https://doi.org/10.1016/j.ejmech.2015.03.022 ![]() |
[67] |
Vásquez Marcano RGDJ, Tominaga TT, Khalil NM, et al. (2018) Chitosan functionalized poly (ϵ-caprolactone) nanoparticles for amphotericin B delivery. Carbohydr Polym 202: 345-354. https://doi.org/10.1016/j.carbpol.2018.08.142 ![]() |
[68] |
Chhonker YS, Prasad YD, Chandasana H, et al. (2015) Amphotericin-B entrapped lecithin/chitosan nanoparticles for prolonged ocular application. Int J Biol Macromol 72: 1451-8. https://doi.org/10.1016/j.ijbiomac.2014.10.014 ![]() |
[69] |
Bhattacharjee S, Joshi R, Chughtai AA, et al. (2021) Graphene-and nanoparticle-embedded antimicrobial and biocompatible cotton/silk fabrics for protective clothing. ACS Appl Bio Mater 4: 6175-6185. https://doi.org/10.1021/acsabm.1c00508. 10.1021/acsabm.1c00508 ![]() |
[70] |
Kharaghani D, Dutta D, Gitigard P, et al. (2019) Development of antibacterial contact lenses containing metallic nanoparticles. Polym Test 79: 106034. https://doi.org/10.1016/j.polymertesting.2019.106034 ![]() |
[71] |
Kalita S, Kandimalla R, Bhowal AC, et al. (2018) Functionalization of β-lactam antibiotic on lysozyme capped gold nanoclusters retrogress MRSA and its persisters following awakening. Sci Rep 8: 1-13. https://doi.org/10.1038/s41598-018-22736-5 ![]() |
[72] |
Konop M, Czuwara J, Kłodzińska E, et al. (2020) Evaluation of keratin biomaterial containing silver nanoparticles as a potential wound dressing in full-thickness skin wound model in diabetic mice. J Tissue Eng Regen Med 14: 334-346. https://doi.org/10.1002/term.2998 ![]() |
[73] |
Gupta A, Briffa SM, Swingler S, et al. (2020) Synthesis of silver nanoparticles using curcumin-cyclodextrins loaded into bacterial cellulose-based hydrogels for wound dressing applications. Biomacromolecules 21: 1802-1811. https://doi.org/10.1021/acs.biomac.9b01724 ![]() |
[74] |
Urbán P, Liptrott NJ, Bremer S (2019) Overview of the blood compatibility of nanomedicines: A trend analysis of in vitro and in vivo studies. Wiley Interdiscip Rev Nanomed Nanobiotechnol 11: e1546. https://doi.org/10.1002/wnan.1546 ![]() |
[75] |
de la Harpe KM, Kondiah PPD, Choonara YE, et al. (2019) The hemocompatibility of nanoparticles: A review of cell-nanoparticle interactions and hemostasis. Cells 8: 1209. https://doi.org/10.3390/cells8101209 ![]() |
[76] |
Guo S, Shi Y, Liang Y, et al. (2021) Relationship and improvement strategies between drug nanocarrier characteristics and hemocompatibility: What can we learn from the literature. Asian J Pharm Sci 16: 551-576. https://doi.org/10.1016/j.ajps.2020.12.002 ![]() |
[77] |
Mikušová V, Mikuš P (2021) Advances in chitosan-based nanoparticles for drug delivery. Int J Mol Sci 22: 9652. https://doi.org/10.3390/ijms22179652 ![]() |
[78] |
Pathak N, Singh P, Singh PK, et al. (2022) Biopolymeric nanoparticles based effective delivery of bioactive compounds toward the sustainable development of anticancerous therapeutics. Front Nutr 9: 963413. https://doi.org/10.3389/fnut.2022.963413 ![]() |
[79] |
Chen HT, Neerman MF, Parrish AR, et al. (2004) Cytotoxicity, hemolysis, and acute in vivo toxicity of dendrimers based on melamine, candidate vehicles for drug delivery. J Am Chem Soc 126: 10044-10048. https://doi.org/10.1021/ja048548j ![]() |
[80] |
Jia YP, Ma BY, Wei XW, et al. (2017) The in vitro and in vivo toxicity of gold nanoparticles. Chin Chem Lett 28: 691-702. https://doi.org/10.1016/j.cclet.2017.01.021 ![]() |
[81] |
Chetyrkina MR, Fedorov FS, Nasibulin AG (2022) In vitro toxicity of carbon nanotubes: a systematic review. RSC Adv 25: 16235-16256. https://doi.org/10.1039/D2RA02519A ![]() |
[82] |
Mahmoudi M, Hofmann H, Rothen-Rutishauser B, et al. (2012) Petri-Assessing the in vitro and in vivo Toxicity of Superparamagnetic Iron Oxide Nanoparticles. Chem Rev 112: 2323-2338. https://doi.org/10.1021/cr2002596 ![]() |
[83] |
Chen L, Liu J, Zhang Y, et al. (2018) The toxicity of silica nanoparticles to the immune system. Nanomedicine 13: 1939-1962. https://doi.org/10.2217/nnm-2018-0076 ![]() |
[84] |
Hadipour Moghaddam SP, Mohammadpour R, Ghandehari H (2019) In vitro and in vivo evaluation of degradation, toxicity, biodistribution, and clearance of silica nanoparticles as a function of size, porosity, density, and composition. J Control Release 311–312: 1-15. https://doi.org/10.1016/j.jconrel.2019.08.028 ![]() |
[85] |
Zhang XF, Shen W, Gurunathan S (2016) Silver nanoparticle-mediated cellular responses in various cell lines: An in vitro model. Int J Mol Sci 17: 1603. https://doi.org/10.3390/ijms17101603 ![]() |
[86] | Yuan YG, Zhang S, Hwang JY, et al. (2018) Silver nanoparticles potentiates cytotoxicity and apoptotic potential of camptothecin in human cervical cancer cells. Oxid Med Cell Longev 18: 6121328. https://doi.org/10.1155/2018/6121328 |
[87] |
Rim KT, Song SW, Kim HY (2013) Oxidative DNA damage from nanoparticle exposure and its application to workers' health: a literature review. Saf Health Work 4: 177-186. https://doi.org/10.1016/j.shaw.2013.07.006 ![]() |
[88] |
Wen H, Dan M, Yang Y, et al. (2017) Acute toxicity and genotoxicity of silver nanoparticle in rats. PLOS One 2: e0185554. https://doi.org/10.1371/journal.pone.0185554 ![]() |
[89] |
Hassanen EI, Khalaf AA, Tohamy AF, et al. (2019) Toxicopathological and immunological studies on different concentrations of chitosan-coated silver nanoparticles in rats. Int J Nanomedicine 14: 4723-4739. https://doi.org/10.2147/IJN.S207644 ![]() |
[90] |
Panyam J, Labhasetwar V (2003) Biodegradable nanoparticles for drug and gene delivery to cells and tissue. Adv Drug Deliv Rev 55: 329-347. https://doi.org/10.1016/S0169-409X(02)00228-4 ![]() |
[91] |
Almofti MR, Ichikawa T, Yamashita K, et al. (2003) Silver ion induces a cyclosporine a-insensitive permeability transition in rat liver mitochondria and release of apoptogenic cytochrome C. J Biochem 134: 43-49. https://doi.org/10.1093/jb/mvg111 ![]() |
[92] | Iancu SD, Albu C, Chiriac L, et al. (2020) Assessment of gold-coated iron oxide nanoparticles as negative T2 contrast agent in small animal MRI studies. Int J Nanomedicine 4811–4824. https://doi.org/10.2147/IJN.S253184 |
[93] |
Akhlaghi N, Najafpour-Darzi G (2021) Manganese ferrite (MnFe2O4) Nanoparticles: From synthesis to application-A review. J Ind Eng Chem 103: 292-304. https://doi.org/10.1016/j.jiec.2021.07.043 ![]() |
[94] |
Ganapathe LS, Mohamed Md A, Yunus RM (2020) Magnetite (Fe3O4) Nanoparticles in biomedical application: from synthesis to surface functionalisation. Magnetochemistry 6: 68. https://doi.org/10.3390/magnetochemistry6040068 ![]() |
[95] |
Liu Z, Liu Y, Liu S, et al. (2021) The effects of TiO2 nanotubes on the biocompatibility of 3D printed Cu-bearing TC4 alloy. Mater Des 207: 109831. https://doi.org/10.1016/j.matdes.2021.109831 ![]() |
[96] |
Andrade RGD, Ferreira D, Veloso SRS, et al. (2022) Synthesis and cytotoxicity assessment of citrate-coated calcium and manganese ferrite nanoparticles for magnetic hyperthermia. Pharmaceutics 14: 2694. https://doi.org/10.3390/pharmaceutics14122694 ![]() |
[97] |
Rudramurthy GR, Swamy MK, Sinniah UR, et al. (2016) Nanoparticles: alternatives against drug-resistant pathogenic microbes. Molecules 21: 836. https://doi.org/10.3390/molecules21070836 ![]() |
[98] |
Shiri S, Abbasi N, Alizadeh K, et al. (2019) Novel and green synthesis of a nanopolymer and its use as a drug delivery system of silibinin and silymarin extracts in the olfactory ensheathing cells of rats in normal and high-glucose conditions. RSC Adv 9: 38912-38927. https://doi.org/10.1039/C9RA05608D ![]() |
[99] |
Sharma S, Sudhakara P, Singh J, et al. (2021) Critical review of biodegradable and bioactive polymer composites for bone tissue engineering and drug delivery applications. Polymers (Basel) 13: 2623. https://doi.org/10.3390/polym13162623 ![]() |
[100] |
Makadia HK, Siegel SJ (2011) Poly Lactic-co-Glycolic Acid (PLGA) as biodegradable controlled drug delivery carrier. Polymers (Basel) 3: 1377-1397. https://doi.org/10.3390/polym3031377 ![]() |
[101] | Félix Lanao RP, Jonker AM, Wolke JG, et al. (2013) Physicochemical properties and applications of poly(lactic-co-glycolic acid) for use in bone regeneration. Tissue Eng Part B Rev 19: 380-390. https://doi.org/10.1089/ten.teb.2012.0443 |
[102] |
da Silva D, Kaduri M, Poley M, et al. (2018) Biocompatibility, biodegradation and excretion of polylactic acid (PLA) in medical implants and theranostic systems. Chem Eng J 340: 9-14. https://doi.org/10.1016/j.cej.2018.01.010 ![]() |
[103] |
Ramos DP, Sarjinsky S, Alizadehgiashi M, et al. (2019) Polyelectrolyte vs polyampholyte behavior of composite chitosan/ gelatin films. ACS Omega 4: 8795-8803. http://pubs.acs.org/journal/acsodf ![]() |
[104] |
Olasehinde TA, Olaniran AO (2022) Neurotoxicity of polycyclic aromatic hydrocarbons: a systematic mapping and review of neuropathological mechanisms. Toxics 10: 417. https://doi.org/10.3390/toxics10080417 ![]() |
[105] |
Liu Y, Hardie J, Zhang X, et al. (2017) Effects of engineered nanoparticles on the innate immune system. Semin Immunol 34: 25-32. https://doi.org/10.1016/j.smim.2017.09.011 ![]() |
[106] |
Corbo C, Molinaro R, Parodi A, et al. (2016) The impact of nanoparticle protein corona on cytotoxicity, immunotoxicity and target drug delivery. Nanomedicine (Lond) 11: 81-100. https://doi.org/10.2217/nnm.15.188 ![]() |
[107] |
Suk JS, Xu Q, Kim N, et al. (2016) PEGylation as a strategy for improving nanoparticle-based drug and gene delivery. Adv Drug Deliv Rev 99: 28-51. https://doi.org/10.1016/j.addr.2015.09.012 ![]() |
[108] |
Tan X, Fang Y, Ren Y, et al. (2019) D-α-tocopherol polyethylene glycol 1000 succinate-modified liposomes with an siRNA corona confer enhanced cellular uptake and targeted delivery of doxorubicin via tumor priming. Int J Nanomedicine 14: 1255-1268. https://doi.org/10.2147/IJN.S191858 ![]() |
[109] |
Neophytou CM, Mesaritis A, Gregoriou G, et al. (2019) d-a-Tocopheryl Polyethylene Glycol 1000 Succinate and a small-molecule Survivin suppressant synergistically induce apoptosis in SKBR3 breast cancer cells. Sci Rep 9: 14375. https://doi.org/10.1038/s41598-019-50884-9 ![]() |
[110] |
Dang Y, Guan J (2020) Nanoparticle-based drug delivery systems for cancer therapy. Smart Mater Med 1: 10-19. https://doi.org/10.1016/j.smaim.2020.04.001 ![]() |
[111] |
Adepu S, Ramakrishna S (2021) Controlled drug delivery systems: current status and future directions. Molecules 26: 5905. https://doi.org/10.3390/molecules26195905 ![]() |
[112] |
Yu B, Tai HC, Xue W, et al. (2010) Receptor-targeted nanocarriers for therapeutic delivery to cancer. Mol Membr Biol 27: 286-298. https://doi.org/10.3109/09687688.2010.521200 ![]() |
[113] |
Yu H, Yang Z, Li F, et al. (2020) Cell-mediated targeting drugs delivery systems. Drug Deliv 27: 1425-1437. https://doi.org/10.1080/10717544.2020.1831103 ![]() |
[114] |
Zhao Z, Ukidve A, Kim J, et al. (2020) Targeting strategies for tissue-specific drug delivery. Cell 181: 2020. https://doi.org/10.1016/j.cell.2020.02.001 ![]() |
[115] |
Manzari MT, Shamay Y, Kiguchi H, et al. (2021) Targeted drug delivery strategies for precision medicines. Nat Rev Mater 6: 351-370. https://doi.org/10.1038/s41578-020-00269-6 ![]() |
[116] |
Mitchell MJ, Billingsley MM, Haley RM, et al. (2021) Engineering precision nanoparticles for drug delivery. Nat Rev Drug Discov 20: 101-124. https://doi.org/10.1038/s41573-020-0090-8 ![]() |
[117] |
Xie J, Lee S, Chen X (2010) Nanoparticle-based theranostic agents. Adv Drug Deliv Rev 62: 1064-1079. https://doi.org/10.1016/j.addr.2010.07.009 ![]() |
[118] |
Chatterjee DK, Diagaradjane P, Krishnan S (2011) Nanoparticle-mediated Hyperthermia in Cancer Therapy. Ther Deliv 2: 1001-1014. https://doi.org/10.4155/tde.11.72 ![]() |
[119] |
Jacque D, Martínez Maestro L, del Rosal B, et al. (2014) Nanoparticles for photothermal therapies. Nanoscale 6: 9494-9530. https://doi.org/10.1039/C4NR00708E ![]() |
[120] |
Zhou Y, Quan G, Wu Q, et al. (2018) Mesoporous silica nanoparticles for drug and gene delivery. Acta Pharmaceutica Sinica B 8: 165-177. https://doi.org/10.1016/j.apsb.2018.01.007 ![]() |
[121] |
Li Z, Barnes JC, Bosoy A, et al. (2012) Mesoporous silica nanoparticles in biomedical applications. Chem Soc Rev 41: 2590-2605. https://doi.org/10.1039/c1cs15246g ![]() |
[122] |
De Jong WH, Borm PJ (2008) Drug delivery and nanoparticles:applications and hazards. Int J Nanomedicine 3: 133-149. https://doi.org/10.2147/IJN.S596 ![]() |
[123] |
Patra JK, Das G, Fraceto LF, et al. (2018) Nano based drug delivery systems: recent developments and future prospects. J Nanobiotechnol 16: 71. https://doi.org/10.1186/s12951-018-0392-8 ![]() |
[124] |
Masarudin MJ, Cutts SM, Evison BJ, et al. (2015) Factors determining the stability, size distribution, and cellular accumulation of small, monodisperse chitosan nanoparticles as candidate vectors for anticancer drug delivery: application to the passive encapsulation of [(14)C]-doxorubicin. Nanotechnol Sci Appl 8: 67-80. https://doi.org/10.2147/NSA.S91785 ![]() |
[125] |
Ajdary M, Moosavi MA, Rahmati M, et al. (2018) Health concerns of various nanoparticles: a review of their in vitro and in vivo toxicity. Nanomaterials (Basel) 8: 634. https://doi.org/10.3390/nano8090634 ![]() |
[126] |
Ray P, Haideri N, Haque I, et al. (2021) The impact of nanoparticles on the immune system: a gray zone of nanomedicine. J Immunological Sci 5: 19-33. https://doi.org/10.29245/2578-3009/2021/1.1206 ![]() |
[127] |
Abdal Dayem A, Hossain MK, Lee SB, et al. (2017) The role of reactive oxygen species (ros) in the biological activities of metallic nanoparticles. Int J Mol Sci 18: 120. https://doi.org/10.3390/ijms18010120 ![]() |
[128] |
Ray PC, Yu H, Fu PP (2009) Toxicity and environmental risks of nanomaterials: challenges and future needs. J Environ Sci Health C Environ Carcinog Ecotoxicol Rev 27: 1-35. https://doi.org/10.1080/10590500802708267 ![]() |
[129] |
Egbuna C, Parmar VK, Jeevanandam J, et al. (2021) Toxicity of nanoparticles in biomedical application: nanotoxicology. J Toxicol 2021:9954443: 1-21. https://doi.org/10.1155/2021/9954443 ![]() |
[130] |
Huang YW, Cambre M, Lee HJ (2017) The toxicity of nanoparticles depends on multiple molecular and physicochemical mechanisms. Int J Mol Sci 18: 2702. https://doi.org/10.3390/ijms18122702 ![]() |
[131] |
You DJ, Bonner JC (2020) Susceptibility factors in chronic lung inflammatory responses to engineered nanomaterials. Int J Mol Sci 21: 7310. https://doi.org/10.3390/ijms21197310 ![]() |
[132] |
Singh A, Kukreti R, Saso L, et al. (2019) Oxidative stress: A key modulator in neurodegenerative diseases. Molecules 24: 1583. https://doi.org/10.3390/molecules24081583 ![]() |
[133] |
Gänger S, Schindowski K (2018) Tailoring formulations for intranasal nose-to-brain delivery: a review on architecture, physico-chemical characteristics and mucociliary clearance of the nasal olfactory mucosa. Pharmaceutics 10: 116. https://doi.org/10.3390/pharmaceutics10030116 ![]() |
[134] |
Guo C, Xia Y, Niu P, et al. (2015) Silica nanoparticles induce oxidative stress, inflammation, and endothelial dysfunction in vitro via activation of the MAPK/Nrf2 pathway and nuclear factor-κB signaling. Int J Nanomedicine 10: 1463-1477. https://doi.org/10.2147/IJN.S76114 ![]() |
[135] |
Uskoković V (2021) Nanomedicine for the poor: a lost cause or an idea whose time has yet to come?. Nanomedicine (Lond) 16: 1203-1218. https://doi.org/10.2217/nnm-2021-0024 ![]() |
[136] | Shubhika Kwatra (2013) Nanotechnology and medicine–The upside and the downside. Int. J Drug Dev Res 5: 1-10. http://www.ijddr.in |
[137] |
Diwan A, Tatake J, Chakraborty A (2022) Therapeutic uses of TheraCour™ polymeric nanomicelles against cancer, infectious diseases and more. Nanomaterials for Cancer Detection Using Imaging Techniques and Their Clinical Applications.Springer Nature 473-506. https://doi.org/10.1007/978-3-031-09636-5_17 ![]() |
[138] |
Chakraborty A, Diwan A, Barton R, et al. (2022) A new antiviral regimen against sars-cov-2 based on nanoviricide's biopolymer (NV-CoV-2). Front Nanotechnol 4: 891605. https://doi.org/10.3389/fnano.2022.891605 ![]() |
[139] | Barton RW, Tatake JG, Diwan AR (2011) Nanoviricides-A novel approach to antiviral therapeutics. Bionanotechnology II.CRC Press 141-154. |
[140] |
Chakraborty A, Diwan A (2020) NL-63: A better surrogate virus for studying SARS-CoV-2. Integr Mol Med 7: 1-9. https://doi.org/10.15761/IMM.1000408 ![]() |
[141] | NanoViricides, Inc., Pan-coronavirus COVID-19 Drug Candidates Are Highly Effective in Pre-clinical Animal Studies in Support of FDA Pre IND Application (2021). Available from: https://www.bloomberg.com/press-releases/2021-03-09/nanoviricides-inc-pan-coronavirus-covid-19-drug-candidates-are-highly-effective-in-pre-clinical-animal-studies-i. |
[142] | Barton RW, Tatake JG, Diwan AR (2016) Nanoviricides: Targeted Anti-Viral Nanomaterials. Handbook of Clinical Nanomedicine, Nanoparticles, Imaging, Therapy, and Clinical Applications.Jenny Stanford Publishing 1039-1046. |
[143] | NanoViricides is Developing Drugs Against SARS-CoV-2 with an Integrated Approach to Combat COVID-19, as Reported at The LD 500 Virtual Conference (2020). Available from: https://www.accesswire.com/604794/NanoViricides-is-Developing-Drugs-Against-SARS-CoV-2-with-an-Integrated-Approach-to-Combat-COVID-19-as-Reported-at-The-LD-500-Virtual-Conference. |
[144] | Pal M, Berhanu G, Desalegn C, et al. (2020) Severe acute respiratory syndrome coronavirus-2 (sars-cov-2): an update. Cureus 12: e7423. https://doi.org/10.7759/cureus.7423 |
[145] |
Chatterjee S, Bhattacharya M, Nag S, et al. (2023) A detailed overview of sars-cov-2 omicron: its sub-variants, mutations and pathophysiology, clinical characteristics, immunological landscape, immune escape, and therapies. Viruses 15: 167. https://doi.org/10.3390/v15010167 ![]() |
[146] |
Beigel JM, Tomashek KM, Dodd LE, et al. (2020) Remdesivir for the treatment of Covid-19 final report. N Engl J Med 83: 1813-1826. https://doi.org/10.1056/NEJMoa2007764 ![]() |
[147] | ClinicalTrials.gov, multicenter, retrospective study of the effects of remdesivir in the treatment of severe Covid-19 infections, 2021. Available from: https://clinicaltrials.gov/ct2/show/NCT04365725 |
[148] | Chakraborty A, Diwan A, Arora V, et al. (2022) Polymer protects the drug and improves its pharmacokinetics. EC Pharmacol Toxicol 10.2: 108-118. https://ecronicon.org/assets/ecpt/pdf/ECPT-10-00707.pdf |
[149] |
Osorno LL, Brandley AN, Maldonado DE, et al. (2021) Review of contemporary self-assembled systems for the controlled delivery of therapeutics in medicine. Nanomaterials (Basel) 11: 278. https://doi.org/10.3390/nano11020278 ![]() |
[150] |
Yadav S, Sharma AK, Kumar P (2020) Nanoscale self-assembly for therapeutic delivery. Front Bioeng Biotechnol 8: 127. https://doi.org/10.3389/fbioe.2020.00127 ![]() |
[151] |
Li H, Labean TH, Leong KW (2011) Nucleic acid-based nanoengineering: novel structures for biomedical applications. Interface Focus 1: 702-24. https://doi.org/10.1098/rsfs.2011.0040 ![]() |
[152] |
Marshall ML, Wagstaff KM (2020) Internalized functional DNA aptamers as alternative cancer therapies. Front Pharmacol 11: 1115. https://doi.org/10.3389/fphar.2020.01115 ![]() |
[153] |
Ni X, Castanares M, Mukherjee A, et al. (2011) Nucleic acid aptamers: clinical applications and promising new horizons. Curr Med Chem 18: 4206-14. https://doi.org/10.2174/092986711797189600 ![]() |
[154] |
Li J, Mo L, Lu CH, et al. (2016) Functional nucleic acid-based hydrogels for bioanalytical and biomedical applications. Chem Soc Rev 45: 1410-31. https://doi.org/10.1039/C5CS00586H ![]() |
[155] |
Abune L, Wang Y (2021) Affinity hydrogels for protein delivery. Trends Pharmacol Sci 42: 300-312. https://doi.org/10.1016/j.tips.2021.01.005 ![]() |
1. | Shinji Matsumoto, Taiki Katayama, Tetsuo Yasutaka, Shingo Tomiyama, Saburou Yamagata, Component separation and origin estimation of mining-influenced water based on fluoride ions and water isotopes in underground legacy mine, Central Japan, 2024, 54, 22145818, 101856, 10.1016/j.ejrh.2024.101856 |
TESTS | REFERENCE METHODS | TESTS | REFERENCE METHODS |
Accredited tests in chemistry | Accredited tests in chemistry | ||
pH | NF T 90-008/NMISO10523 | As | FD T 90-119 |
T ℃ | NF EN 25667 (ISO 5667)/NM 03.7.008 | As | NF EN ISO 11885 |
Sulfates | NF T 90-040/NM ISO 9280 | Al | NF EN ISO 11885 |
Pb | FD T 90-112 | Al | FD T 90-119 |
Pb | FD T 90-119 | Zn | FD T 90-112 |
Pb | NF EN ISO 11885 | Zn | NF EN ISO 11885 |
Cd | NFENISO5961 | Cu | FD T 90-112 |
Cd | NF EN ISO 11885 | Cu | NF EN ISO 11885 |
Fe | FD T 90-112 | Cr | NF EN1233 |
Fe | NF EN ISO 11885 | Cr | NF EN ISO 11885 |
Mn | NF EN ISO 11885 | Sb | NF EN ISO 11885 |
Mn | FD T 90-112 | Ni | FD T 90-119 |
Co | NM 03-7-022 | Ni | NF EN ISO 11885 |
Co | NF EN ISO 11885 | Se | NF EN ISO 11885 |
Sn | NF EN ISO 11885 | Se | Se FD T 90-119 |
Ag | NF EN ISO 11885 |
Average MTE concentrations (µg/L) | Industrial site | Study area | Exploited substance | Reference | |||||
Pb | Cd | As | Cu | Zn | |||||
Mine water discharges | 23, 17 | 1, 4 | 23, 87 | 53, 81 | 131, 67 | HBM | Sebou watershed | n. d | This Work |
Mine lakes | 27, 5 | n. d | 92, 825 | n. d | n. d | Zeïda Mine | HauteMoulouya | Pb | [111] |
drainage water | n. d | n. d | n. d | 58000 | 45000 | Kettara Mine | Kettara River Jebilet | Fe-S | [102,116,117] |
Mine lakes | 31, 186 | n. d | 111, 202 | n. d | n. d | Zeïda Mine | Haute Moulouya | Pb | [63] |
Leachate of mine tailing | 306, 875 | 9, 125 | 763 | 100686 | 8597 | Kettara Mine | Kettara RiverJebilet | Fe-S | [115] |
n. d.: Undetermined value |
Average MTE concentrations (µg/L) | Industrial site | Study area | Exploited substance | Reference | ||||||
Pb | Cd | As | Cu | Zn | ||||||
Morocco | Ex1 (C1) | 61 | 1, 1 | 2, 5 | 4, 2 | 190 | HBM | Sebou watershed | n.d | This work |
Ex1 (C2) | 5, 6 | 0, 5 | 3, 6 | 2 | 115 | |||||
Ex2 (C1) | 72 | 8, 3 | 83 | 406 | 528 | |||||
Ex2 (C2) | 1, 7 | 0, 2 | 9, 3 | 1, 4 | 2, 2 | |||||
Ex3 (C1) | 28 | 0, 3 | 48 | 3, 3 | 60 | |||||
Ex3 (C2) | 1, 7 | 0, 2 | 25 | 1, 4 | 2, 2 | |||||
Ex4 (C1) | 9 | 0, 4 | 16 | 8, 8 | 143 | |||||
Ex4 (C2) | 6, 4 | 0, 2 | 3, 6 | 3, 4 | 13 | |||||
Average | 23, 17 | 1, 4 | 23, 87 | 53, 81 | 131, 67 | |||||
France | 29 | n. d. | 2, 14 | 5 | 9, 28 | Escarro mine | Languedoc RoussillonTêt watershed | CaF2 | [119] | |
11, 12 | 2, 7 | n. d. | n. d. | 4, 21 | Malines mine | Saint-Laurent-le-MinierHérault watershed | Pb, ZnS, PbS, Zn | [118] | ||
England | 10, 2 | 0, 84 | n. d. | 0, 56 | 160, 2 | Soughs mine | DerbyshireBugsworth watershed | Pb | [118] | |
Norway | n. d. | 12800 | 280 | 574000 | 5640000 | Killingdal mine | Sør-TrøndelagGaula watershed | Cu Zn S | [120] | |
n. d.: Undetermined value |
TESTS | REFERENCE METHODS | TESTS | REFERENCE METHODS |
Accredited tests in chemistry | Accredited tests in chemistry | ||
pH | NF T 90-008/NMISO10523 | As | FD T 90-119 |
T ℃ | NF EN 25667 (ISO 5667)/NM 03.7.008 | As | NF EN ISO 11885 |
Sulfates | NF T 90-040/NM ISO 9280 | Al | NF EN ISO 11885 |
Pb | FD T 90-112 | Al | FD T 90-119 |
Pb | FD T 90-119 | Zn | FD T 90-112 |
Pb | NF EN ISO 11885 | Zn | NF EN ISO 11885 |
Cd | NFENISO5961 | Cu | FD T 90-112 |
Cd | NF EN ISO 11885 | Cu | NF EN ISO 11885 |
Fe | FD T 90-112 | Cr | NF EN1233 |
Fe | NF EN ISO 11885 | Cr | NF EN ISO 11885 |
Mn | NF EN ISO 11885 | Sb | NF EN ISO 11885 |
Mn | FD T 90-112 | Ni | FD T 90-119 |
Co | NM 03-7-022 | Ni | NF EN ISO 11885 |
Co | NF EN ISO 11885 | Se | NF EN ISO 11885 |
Sn | NF EN ISO 11885 | Se | Se FD T 90-119 |
Ag | NF EN ISO 11885 |
Average MTE concentrations (µg/L) | Industrial site | Study area | Exploited substance | Reference | |||||
Pb | Cd | As | Cu | Zn | |||||
Mine water discharges | 23, 17 | 1, 4 | 23, 87 | 53, 81 | 131, 67 | HBM | Sebou watershed | n. d | This Work |
Mine lakes | 27, 5 | n. d | 92, 825 | n. d | n. d | Zeïda Mine | HauteMoulouya | Pb | [111] |
drainage water | n. d | n. d | n. d | 58000 | 45000 | Kettara Mine | Kettara River Jebilet | Fe-S | [102,116,117] |
Mine lakes | 31, 186 | n. d | 111, 202 | n. d | n. d | Zeïda Mine | Haute Moulouya | Pb | [63] |
Leachate of mine tailing | 306, 875 | 9, 125 | 763 | 100686 | 8597 | Kettara Mine | Kettara RiverJebilet | Fe-S | [115] |
n. d.: Undetermined value |
Average MTE concentrations (µg/L) | Industrial site | Study area | Exploited substance | Reference | ||||||
Pb | Cd | As | Cu | Zn | ||||||
Morocco | Ex1 (C1) | 61 | 1, 1 | 2, 5 | 4, 2 | 190 | HBM | Sebou watershed | n.d | This work |
Ex1 (C2) | 5, 6 | 0, 5 | 3, 6 | 2 | 115 | |||||
Ex2 (C1) | 72 | 8, 3 | 83 | 406 | 528 | |||||
Ex2 (C2) | 1, 7 | 0, 2 | 9, 3 | 1, 4 | 2, 2 | |||||
Ex3 (C1) | 28 | 0, 3 | 48 | 3, 3 | 60 | |||||
Ex3 (C2) | 1, 7 | 0, 2 | 25 | 1, 4 | 2, 2 | |||||
Ex4 (C1) | 9 | 0, 4 | 16 | 8, 8 | 143 | |||||
Ex4 (C2) | 6, 4 | 0, 2 | 3, 6 | 3, 4 | 13 | |||||
Average | 23, 17 | 1, 4 | 23, 87 | 53, 81 | 131, 67 | |||||
France | 29 | n. d. | 2, 14 | 5 | 9, 28 | Escarro mine | Languedoc RoussillonTêt watershed | CaF2 | [119] | |
11, 12 | 2, 7 | n. d. | n. d. | 4, 21 | Malines mine | Saint-Laurent-le-MinierHérault watershed | Pb, ZnS, PbS, Zn | [118] | ||
England | 10, 2 | 0, 84 | n. d. | 0, 56 | 160, 2 | Soughs mine | DerbyshireBugsworth watershed | Pb | [118] | |
Norway | n. d. | 12800 | 280 | 574000 | 5640000 | Killingdal mine | Sør-TrøndelagGaula watershed | Cu Zn S | [120] | |
n. d.: Undetermined value |