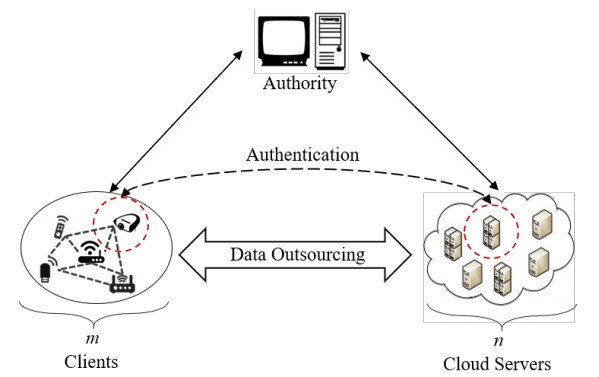
The efficiency of photovoltaic (PV) panels is significantly affected by environmental factors such as solar irradiance, wind speed, humidity, dust accumulation, shading, and surface temperature, with thermal buildup being the primary cause of efficiency degradation. In this review, we examined various cooling techniques to mitigate heat accumulation and enhance PV panel performance. A comprehensive analysis of active, passive, and hybrid cooling strategies is presented, including heat pipe-based cooling, heat sinks, holographic films, nanofluids, phase change materials (PCM), thermoelectric, biomaterial-based, and hybrid cooling systems. The effectiveness of these techniques in reducing surface temperature and improving electrical efficiency was assessed. Notably, heat pipe cooling and hybrid PCM-thermoelectric systems demonstrated the most promising improvements, with some methods achieving temperature reductions exceeding 40 ℃ and efficiency enhancements over 15%. Future research directions include developing advanced nanofluid formulations, optimizing the design of heat pipes and heat sinks, integrating multi-functional coatings, and enhancing the real-world durability of cooling materials for inventing innovative, sustainable, and eco-friendly cooling systems. By providing a structured assessment of emerging PV cooling techniques, this study is a valuable resource for researchers and engineers striving to improve solar energy efficiency, reduce thermal losses, and advance the sustainability of photovoltaic technologies.
Citation: Kaovinath Appalasamy, R Mamat, Sudhakar Kumarasamy. Smart thermal management of photovoltaic systems: Innovative strategies[J]. AIMS Energy, 2025, 13(2): 309-353. doi: 10.3934/energy.2025013
[1] | Jindan Zhang, Urszula Ogiela, David Taniar, Nadia Nedjah . Improved cloud storage auditing scheme with deduplication. Mathematical Biosciences and Engineering, 2023, 20(5): 7905-7921. doi: 10.3934/mbe.2023342 |
[2] | Mikail Mohammed Salim, Jungho Kang, Yi Pan, Jong Hyuk Park . A Lightweight authentication scheme for IoT against Rogue Base Station Attacks. Mathematical Biosciences and Engineering, 2022, 19(11): 11735-11755. doi: 10.3934/mbe.2022546 |
[3] | Kittur Philemon Kibiwott , Yanan Zhao , Julius Kogo, Fengli Zhang . Verifiable fully outsourced attribute-based signcryption system for IoT eHealth big data in cloud computing. Mathematical Biosciences and Engineering, 2019, 16(5): 3561-3594. doi: 10.3934/mbe.2019178 |
[4] | Ridha Ouni, Kashif Saleem . Secure smart home architecture for ambient-assisted living using a multimedia Internet of Things based system in smart cities. Mathematical Biosciences and Engineering, 2024, 21(3): 3473-3497. doi: 10.3934/mbe.2024153 |
[5] | Han-Yu Lin, Tung-Tso Tsai, Hong-Ru Wu, Miao-Si Ku . Secure access control using updateable attribute keys. Mathematical Biosciences and Engineering, 2022, 19(11): 11367-11379. doi: 10.3934/mbe.2022529 |
[6] | Yongjun Ren, Yan Leng, Yaping Cheng, Jin Wang . Secure data storage based on blockchain and coding in edge computing. Mathematical Biosciences and Engineering, 2019, 16(4): 1874-1892. doi: 10.3934/mbe.2019091 |
[7] | Lihong Guo, Jian Wang, Haitao Wu, Najla Al-Nabhan . XML security protection scheme based on Kerberos authentication and polynomials authorization. Mathematical Biosciences and Engineering, 2020, 17(5): 4609-4630. doi: 10.3934/mbe.2020254 |
[8] | Yifeng Yin, Zhaobo Wang, Wanyi Zhou, Yong Gan, Yanhua Zhang . Group key agreement protocol for edge computing in industrial internet. Mathematical Biosciences and Engineering, 2022, 19(12): 12730-12743. doi: 10.3934/mbe.2022594 |
[9] | Te-Wei Chiang, Dai-Lun Chiang, Tzer-Shyong Chen, Frank Yeong-Sung Lin, Victor R. L. Shen, Min-Chien Wang . Novel Lagrange interpolation polynomials for dynamic access control in a healthcare cloud system. Mathematical Biosciences and Engineering, 2022, 19(9): 9200-9219. doi: 10.3934/mbe.2022427 |
[10] | Shitharth Selvarajan, Hariprasath Manoharan, Celestine Iwendi, Taher Al-Shehari, Muna Al-Razgan, Taha Alfakih . SCBC: Smart city monitoring with blockchain using Internet of Things for and neuro fuzzy procedures. Mathematical Biosciences and Engineering, 2023, 20(12): 20828-20851. doi: 10.3934/mbe.2023922 |
The efficiency of photovoltaic (PV) panels is significantly affected by environmental factors such as solar irradiance, wind speed, humidity, dust accumulation, shading, and surface temperature, with thermal buildup being the primary cause of efficiency degradation. In this review, we examined various cooling techniques to mitigate heat accumulation and enhance PV panel performance. A comprehensive analysis of active, passive, and hybrid cooling strategies is presented, including heat pipe-based cooling, heat sinks, holographic films, nanofluids, phase change materials (PCM), thermoelectric, biomaterial-based, and hybrid cooling systems. The effectiveness of these techniques in reducing surface temperature and improving electrical efficiency was assessed. Notably, heat pipe cooling and hybrid PCM-thermoelectric systems demonstrated the most promising improvements, with some methods achieving temperature reductions exceeding 40 ℃ and efficiency enhancements over 15%. Future research directions include developing advanced nanofluid formulations, optimizing the design of heat pipes and heat sinks, integrating multi-functional coatings, and enhancing the real-world durability of cooling materials for inventing innovative, sustainable, and eco-friendly cooling systems. By providing a structured assessment of emerging PV cooling techniques, this study is a valuable resource for researchers and engineers striving to improve solar energy efficiency, reduce thermal losses, and advance the sustainability of photovoltaic technologies.
Internet of Things is a promising technology that may promote human society towards intelligence. However, the constrained local storage space of the devices will be an obstacle for the further development of IoT. Cloud computing is a new emerging technology in recent years. By accessing the wired or wireless network, clients can enjoy the high-quality cloud services. At present, the resources of storage and computing are the main services that cloud computing can provide for consumers [1,2,3]. The resource of clients is constrained due to the IoT devices in the system are lightweight. Therefore, the resource-constrained clients of IoT can outsource their local data to cloud servers for long-term data storage. Moreover, clients can enjoy the unlimited storage resource and computing capability anytime and anywhere via the Internet [4,5]. As the cloud server can provide virtual resources for clients, they no longer need to worry about the local hardware investments [6]. Clients only need to pay the fees of cloud usage as a manner of pay-as-you-use. Hence, with the assist of cloud computing, the technology of IoT may have the further development space.
In the practical cloud usage, the adversaries may impersonate legitimate clients to cheat cloud server, which brings a great security threats to the data stored in cloud servers [7,8]. On the other hand, cloud servers can be accessed by any users through the Internet, which is easy to cause malicious users to attack the server [9,10]. To ensure the storage security and avoid server attack, the authentication protocol should be provided to ensure the security of the system [11,12,13,14,15]. In 2009, Li et al. proposed an identity-based authentication protocol based on identity-based encryption and signature schemes [16]. Due to the superior feature of identity-based cryptography, The scheme of Li et al. is more efficient and very suitable for lightweight clients. To realize more strong security, Choudhury et al. proposed a strong user authentication framework for cloud computing that can strongly verify the legitimacy of users before the participation in the system [17]. Moreover, The scheme of Choudhury et al. can provide the properties of identity management, mutual authentication, session key establishment between cloud users and servers. In 2015, Liu et al. proposed a privacy-preserving authentication protocol based on shared authority [18]. The scheme of Liu et al. can also provide data sharing based on proxy re-encryption. In addition, various security features can realize except authentication in the scheme of Liu et al., such as data anonymity, user privacy, and forward security.
However, the previous schemes only focused on the research of authentication security in cloud computing but ignored the storage security. Moreover, there is no in-depth study on the issue of mutual authentication. Despite some researches have devoted themselves to the research of mutual authentication in this respect, the traditional interactive authentication protocol will bring much communication cost to clients and servers. Hence, a secure mutual authentication with less interacting should be designed. In addition, the storage checking should also be considered in the design of the authentication scheme in cloud-assisted IoT.
In this paper, a secure mutual authentication and storage checking scheme is proposed for cloud-assisted IoT. The main contributions of the proposed scheme are shown as follows.
(1) Chameleon hash signature is used to design the authentication mechanism, which improves the security of authentication. Moreover, the proposed scheme is extended to support mutual authentication between cloud servers and clients that ensures the storage security for clients, and avoid the participation of malicious entities in the system.
(2) By using the encryption of certificate in the authentication of server, the proposed scheme effectively prevents the leakage of sensitive information. Moreover, the proposed scheme can resist the tamper attack and the replay attack.
(3) The proposed scheme also supports storage checking for the stored data in cloud servers. Moreover, the storage checking tasks are delegated to the entity of Authority, which improves the fairness of the storage checking and highly reduces the computational overhead at the client side.
The authentication protocol can ensure the security of communication and storage security in cloud computing [19,20,21,22]. To enhance the security of mobile users in cloud computing, Tsai et al. proposed an efficient authentication for distributed mobile cloud computing [23]. By using the bilinear pairing and dynamic nonce generation, the proposed scheme in [23] can provide mutual authentication, key exchange, user anonymity and so on. In the scheme of Tsai et al., only the target server needs to interact with the request client, which highly reduces the communication cost between the client and the server. To protect the access policies in cloud computing, Liu et al. proposed a hierarchical attribute based access control scheme that can achieve scalability and authentication in realizing write privilege on the stored data [24]. Note that the scheme of Liu et al. can well enhance the security of access control on stored data. To solve the authentication of IoT devices in cloud computing, Amin et al. proposed an architecture that can implement authentication protocol using smartcard [25]. In addition, AVISPA tool and BAN logic model are used in the design of the proposed scheme in the scheme of Amin et al., which ensures the legitimate users can access the sensitive information from the private cloud with various security threats resistance.
The rest of this paper is organized as follows. Section 2 presents the preliminaries. Section 3 provides the system model and the design goals. Section 4 introduces the proposed scheme in detail. Section 5 describes the analysis of the security and the performance of the proposed scheme. The conclusion of this paper is given in Section 6.
In this section, the preliminaries of this paper are provided, including bilinear pairing and chameleon hash signature. The detailed introduction of them is shown as follows.
Bilinear pairing is proposed by Boneh et al. in 2001 and it is widely used to construct all kinds of cryptographic security protocols [26]. In general, bilinear pairing are constructed on elliptic curve or hyperelliptic curve in finite field. In the construction of bilinear pairing, two multiplicative cyclic groups G0 and G1 with large prime order q are defined. The bilinear pairing can be denoted as ˆe:G0×G0→G1. Then, two points P,Q∈G0 and two integers a,b∈Z∗q are randomly selected. Here, Z∗q is a set that consists integers 1 to q-1 with modulo q. The generator of group G0 is G. The properties of the bilinear pairing are shown as follows.
(1) Bilinear: ˆe(Pa,Qb)=ˆe(P,Q)ab;
(2) Non-degenerate: ˆe(G,G)≠1;
(3) Computable: ˆe(P,Q) can be computed by an existing algorithm.
Chameleon hash signature evolved from the technique of chameleon hashing that is a non-interactive signature algorithm [27]. That is to say, the prover generates the signature without interacting with the verifier in chameleon hash signature. The main process of chameleon hash signature is shown as follows.
First, the prover selects s∈RZ∗q as its secret key. Then, the prover computes the chameleon as C=Gs. To realize the authentication, the prover selects private key a∈RZ∗q and computes the corresponding public key as P=Ga. After that, the prover generates an auxiliary parameter mau=CFind(a,nonce,s)=s−aλ, where CFind is a collision finding algorithm, nonce is authentication challenge and λ=H(P⊕nonce). The information of {C,mau,P,nonce} will be sent to the prover for the further authentication. Upon receiving the authentication information from the prover, the verifier will compute a chameleon hash parameter as CH(mau,P,nonce)=Gmau⋅Pλ. According to checking whether the chameleon hash parameter is equal to C, the verifier can successfully authenticate the prover.
This section provides the system model of the proposed scheme. Moreover, the design goals of the proposed scheme are given, including mutual authentication, tamper-resistance and storage checking.
The system model of the proposed scheme consists of three different kinds of entities, which are Clients, Authority and Cloud Servers. The system model of the proposed scheme is shown as Figure 1. The detailed introduction and security features of each entity are shown as follows.
(1) Clients. In the system, clients are the IoT devices who enjoy the cloud services as a manner of pay-per-use through the Internet [28]. The IoT clients are not fully-trusted in the system. Note that the storage resource of clients is limited. To realize the unconstrained data file storage, clients can outsource the data files to cloud servers for long-term storage [29]. However, clients cannot determine whether the servers are legal. Hence, clients should authenticate the validity of a specific server before the data outsourcing. Moreover, clients can check the data file storage in the cloud servers with the help of Authority. Here, clients are honest, but it is also easy for attackers to impersonate legitimate users to participate in the system.
(2) Authority. Compared to clients and cloud servers, the entity of Authority is a fully-trusted entity. Authority is responsible for generating the required security parameters and certificates for clients and cloud servers in the system. Moreover, Authority can check the data file storage on behalf of clients.
(3) Cloud Servers. The cloud servers are distributed and can be connected each other by the Internet. However, cloud servers are honest-but-curious to the stored data. Moreover, cloud servers are vulnerable to be attacked by adversaries. In addition, cloud servers may destroy or delete the infrequently used data file for financial reasons. Hence, cloud servers need to authenticate the validity of the accessed clients and cooperate with the user's data storage checking at any time during the service providing period.
(1) Mutual Authentication. To ensure the storage security and avoid the participation of illegal entities in the system, the proposed scheme should provide a mechanism of mutual authentication. That is to say, every client can authenticate the validity of any one of the cloud servers in the system and each cloud server can implement the authentication of any one of the clients.
(2) Tamper-Resistance. In order to avoid the influence of external factors on the authentication, the proposed scheme should provide the property of tamper-resistance for the authentication. In other words, any attacker cannot modify the authentication information of the entity that needs to be authenticated in the system.
(3) Storage Checking. The proposed scheme should support the storage checking. To improve the fairness and alleviate the computational overhead of the storage checking, the clients in the system can delegate the storage checking tasks to the entity of Authority.
The proposed scheme consists of two parts, including authentication and storage checking. The authentication can realize mutual authentication, namely, the mutual authentication between clients and cloud servers. With the assist of mutual authentication, the proposed scheme can avoid illegal and attacked entities from participating in the system. The mutual authentication is designed based on the idea of chameleon hashing in [30]. Moreover, the proposed scheme supports storage checking, which highly improves the security of the data file storage. The detailed description of the proposed scheme is shown as follows.
In the system, the total number of the clients and cloud servers is m and n, respectively. Assume that client x is a data owner who wants to enjoy the cloud services provided by server r via the Internet, where 1⩽x⩽m and 1⩽r⩽n. However, client x cannot determine whether server r is an authorized server by the Authority. To avoid the harm of malicious servers to users' rights and interests, in the proposed scheme, the clients in the system can authenticate the server before data outsourcing. At the same time, the cloud servers can also authenticate the validity of the accessed clients. The detailed construction of the proposed scheme is shown as follows.
First, client x selects kx∈RZ∗p as its secret key. Then, client x computes a chameleon parameter as CHAx=Gkx by using for the secret key for the certificate computation, where G is a randomly selected point from group G0. After that, client x sends CHAx to Authority in a secure channel. Upon receiving the chameleon parameter of client x, Authority will compute the certificate for client x as Cx=SKA(CHAx), where Ka is the secret key of Authority that is randomly selected from Z∗p. Note that S is an encryption algorithm and the corresponding public decryption key is pkA. Similar to the certificate computation of client x, Authority can compute a certificate for server r according to the received chameleon parameter of server r. The certificate of server r is denoted as Cr=SKA(CHAr), where CHAr=Gk and kr is the secret key of server r.
In the authentication phase, client x randomly selects a private secret key skx from Z∗p. The corresponding public key can be computed as pkx=Gskx. Then, client x computes a security parameter as SPx=CFind(skx,nonce,kx) for the further authentication. Finally, client x sends SPx and its own certificate Cx to server r for verification. According to the received parameters from client x, server r can authenticate client x by checking whether Verify=(Cx,pkA)=CH(SPx,pkx,nonce) can hold using the public keys of Authority and client x. If the authentication of client x can pass, server r will compute encryption key Kxr=skx⋅pkr by using its secret key and the public key of client x. Finally, server r encrypts its certificate Cr using Krx as ECr=EncKrx(Cr). Here, the encryption of Enc is a symmetric encryption. The encrypted certificate of server r will be sent to client x for the validity checking. When receiving the encrypted certificate of server r, client x can compute the decryption key as Krx=skr⋅pkx. Note that Krx=Kxr. Hence, server r's encrypted certificate ECr can be decrypted by client x as C′r=DecKxr(ECr) by using Kxr, where Dec is the decryption algorithm. According to checking whether C∗r is equal to C′r, client x can determine whether server r is a honest server. Here, C∗r is the certificate of server r stored in the certificate list at Authority side. If C∗r≠C′r, client x can check the correctness of equation Verify(Cr,pkA)=CH(SPr,pkr,nonce).
When the mutual authentication between the client and the server is finished, the corresponding client can outsource its own data files to the valid cloud server. The main philosophy of storage checking in this paper is constructed based on the key techniques in studies [31,32]. Suppose that the data file of client x is F=[f1,f2,⋅⋅⋅,fz] and the data file identifier is IdF. The tag of the data file can be computed as tF=IdF||SSigsks(IdF), where SSig is a signature algorithm for tag generation and sks is the secret key of signature generation. The corresponding public key for signature verification is pks. After that, client x computes authenticator δi=(H1(IdF)⋅ηfi)kx for every data file block, where η is security parameter that is chosen from group G0 and 1⩽i⩽z. Moreover, client x computes ω=ˆe(η,CHAx). Finally, client x uploads the local data file blocks with the authenticators and data file tag to cloud server r.
When client x wants to check the data file storage in server r, a specific data file storage checking request with security parameter ω needs to be generated and sent to Authority. Then, Authority retrieves the corresponding data file tag to local side and checks the correctness of it using pks. If the tag can pass the checking, the entity of Authority recovers the data file identifier from tF. To realize secure storage checking, Authority selects ρ parameters vi(1⩽i⩽ρ) and randomly selects a security parameter ci from Zp for every vi. Finally, Authority sends {i,ci} to server r as storage checking challenge.
Upon receiving the challenge from Authority, the cloud server r computes fprx=∑i∈[1,ρ](ci⋅fi) and tprx=∏i∈[1,ρ]δcii according to the stored data file and the corresponding tag. Then, server r sends fprx and tprx to Authority for the storage checking. The entity of Authority computes μf=∏i∈[1,ρ]ˆe((H(IdF))ci,CHAx) and checking whether μf⋅ωfprx=ˆe(tprx,G) can hold.
In this section, the security and the performance of the proposed scheme are evaluated. The detailed evaluation is shown as follows.
Theorem 1. The proposed authentication can be proved to be correct if all required parameters are generated successfully.
Proof of Theorem 1. In the authentication of client x, we can get the chameleon hash result of SPx and pkx is CH(SPx,pkx)=GSPx⋅pkλx. From the description of the authentication, the security parameter of SPx can be computed as SPx=CFind(skx,kx)=kx−skx⋅λ. We have CH(SPx,pkx)=Gkx−skx⋅λ⋅(Gskx)λ=Gkx. According to the computation of the certificate of client x, the certificate can be recovered by pkA as CHAx=Gkx. In addition, similar to the proof of client x authentication, the correctness of server r authentication can also be proved. Here, the detailed description of the server r authentication is omitted. Hence, the mutual authentication presented in the proposed scheme is correct.
Theorem 2. The encrypted certificates of servers can be successfully decrypted by using the generated keys of clients.
Proof of Theorem 2. If Kxr=Krx is correct, the encrypted certificate of server r can be decrypted by client x. According to the above description, we can get that Kxr=skx⋅pkr=skx⋅(skr⋅G)=(skx⋅G)⋅(skr)=pkx⋅skr. The decryption of Krx=skr⋅pkx. Therefore, the certificate decryption in the proposed scheme is correct.
Theorem 3. The correctness of the proposed storage checking mechanism can be proved.
Proof of Theorem 3. If the storage checking equation can be proved to be correct, the proposed storage checking in this paper is correct. The storage checking equation is solved as follows.
μf⋅ωfprx=∏i∈[1,ρ]ˆe((H(IdF))ci,CHAx)⋅ˆe(η,CHAx)∑i∈[1,ρ](ci⋅fi)=∏i∈[1,ρ]ˆe((H(IdF))ci,Gkx)⋅∏i∈[1,ρ]ˆe(η,Gkx)ci⋅fi=∏i∈[1,ρ]ˆe(((H(IdF)⋅ηfi)kx)ci,G)=ˆe(∏i∈[1,ρ]δcii,G)=ˆe(tprx,G) |
That is to say, the proposed storage checking in this paper is correct.
In order to present the efficiency of the proposed scheme, the computational cost at the different entity side in the system is analyzed. The symbols of TE.,TH.,TMul.,TAdd. and TP. are used to denote the time required to execute the operations of exponentiation, hash function, multiplication, addition and pairing, respectively. The computational at each entity side is shown in Table 1. Note that the symbols of ρ and z is the number of the challenged data blocks and the total number of data blocks in the system, respectively. From Table 1, we can find that the computational cost of the client side is determined by the number of the total data blocks. The computational cost of the server side and the Authority side will be increased with the growth of the challenged data blocks. Table 2 shows the computational cost of authentication and storage checking in the proposed scheme. From Table 2, we can see that the computational cost of the phase of Authentication is almost a constant, but the computational cost of the server side will be larger with the increase of the total data blocks and the challenged data blocks.
Entity Side | Computational Cost |
Client | (2z+4)TE+(3+z)TMul.+zTH.+1TAdd.+TP. |
Authority | ρ(2TMul.+TE.+TAdd.) |
Server | (4+ρ)TE.+(2ρ+3)TMul.+ρTH.+TP. |
*TE.,TH.,TMul.,TAdd.,TP.: Time required to perform the corresponding operations.
*ρ: The number of the challenged data blocks. *z: The total number of data blocks. |
Phase | Computational Cost |
Authentication | 7TE+5TMul.+1TAdd. |
Storage Checking | (2z+2ρ+1)TE.+(z+3ρ+1)TMul.+(z+ρ)TH.+ρTAdd.+2TP. |
*TE.,TH.,TMul.,TAdd.,TP.: Time required to perform the corresponding operations.
*ρ: The number of the challenged data blocks. *z: The total number of data blocks. |
In Table 3, the communication cost in the phase of authentication and storage checking is given. Here, we use the symbol of lG and lZ denotes the bit-length of parameters in group and integer field, respectively. From the comparison result in Table 3, we can find that the communication cost of Authentication and Storage Checking is 3lG+1lZ and (4ρ+z+2)lZ, respectively. That is to say, as the number of data blocks increases, the communication cost of Authentication remains unchanged but the communication cost of Storage Checking will be higher.
Phase | Computational Cost | ||
Authentication | 3lG+1lZ | ||
Storage Checking | (4ρ+z+2)lZ | ||
*lG,lZ: Time required to perform the corresponding operations.
*ρ: The number of the challenged data blocks. *z: The total number of data blocks. |
The proposed scheme is simulated on the PBC [26] and GMP [27] constructed experimental platform. The experimental platform is configured on a computer with 8GB RAM and Intel 2.60 GHZ CPU. In the simulation, the basis of total number of data blocks and the challenged data blocks is 100 and 50, respectively. From Figure 2, we can see that the computational time at the side of client, Authority and Server increases linearly with the number of data blocks. Moreover, the computational time at Authority side is the least and the computational time at Client side is the most under the condition of the same number of data blocks. Figure 3 is the simulation of computational time at the different entity side. From Figure 3, we can find that the computational time of Storage Checking phase growth linearly with the number of data blocks. The computational time of Authentication is a constant under the different number of data blocks. Hence, it can be determined that the simulation result is consistent with the result of the above theoretical analysis.
To enhance the security of server access and data storage, in this paper, we propose a secure mutual authentication scheme for cloud computing to implement the authentication between clients and cloud servers. The technique of chameleon hash signature is used to design the authentication mechanism, which makes the designed authentication have a variety of security performance such as tamper attack and replay attack resistance. Moreover, the proposed scheme supports data storage checking for clients. To reduce the local computational overhead, the storage checking tasks are delegated to the fully-trusted entity. Security analysis shows that the proposed mechanisms of authentication and storage checking can be proved to be correct. Performance analysis presents that the proposed scheme has a low computational overhead. Hence, the proposed scheme can be well used in practical cloud-assisted IoT environment for data storage.
This work is supported by the National Science Foundation of China under Grant No. 62102169, No. 62072249, the Natural Science Foundation of the Jiangsu Higher Education Institutions of China under Grant 21KJB520033, the Key Research and Development Program (Social Development) of Lianyungang under Grant SF2102, the Research Start-up Fund of JOU under Grant KQ20039.
The authors declare no conflict of interest.
[1] |
Lv Y (2023) Transitioning to sustainable energy: Opportunities, challenges, and the potential of blockchain technology. Front Energy Res, 11. https://doi.org/10.3389/fenrg.2023.1258044 doi: 10.3389/fenrg.2023.1258044
![]() |
[2] |
Østergaard PA, Duic N, Noorollahi Y, et al. (2020) Sustainable development using renewable energy technology. Renewable Energy 146: 2430–2437. https://doi.org/10.1016/j.renene.2019.08.094 doi: 10.1016/j.renene.2019.08.094
![]() |
[3] |
Rabaia MKH, Abdelkareem MA, Sayed ET, et al. (2021) Environmental impacts of solar energy systems: A review. Sci Total Environ 754: 141989. https://doi.org/10.1016/j.scitotenv.2020.141989 doi: 10.1016/j.scitotenv.2020.141989
![]() |
[4] |
El-Hadary MI, Senthilraja S, Zayed ME (2023) A hybrid system coupling spiral type solar photovoltaic thermal collector and electrocatalytic hydrogen production cell: Experimental investigation and numerical modeling. Process Saf Environ Prot 170: 1101–1120. https://doi.org/10.1016/j.psep.2022.12.079 doi: 10.1016/j.psep.2022.12.079
![]() |
[5] |
Debbarma M, Sudhakar K, Baredar P (2017) Thermal modeling, exergy analysis, performance of BIPV and BIPVT: A review. Renewable Sustainable Energy Rev 73: 1276–1288. https://doi.org/10.1016/j.rser.2017.02.035 doi: 10.1016/j.rser.2017.02.035
![]() |
[6] |
Abd Elaziz M, Senthilraja S, Zayed ME, et al. (2021) A new random vector functional link integrated with mayfly optimization algorithm for performance prediction of solar photovoltaic thermal collector combined with electrolytic hydrogen production system. Appl Therm Eng 193: 117055. https://doi.org/10.1016/j.applthermaleng.2021.117055 doi: 10.1016/j.applthermaleng.2021.117055
![]() |
[7] | Kalogirou SA (2023) Solar energy engineering: Processes and systems. Academic Press (3rd Edition). Avaialble from: https://shop.elsevier.com/books/solar-energy-engineering/kalogirou/978-0-323-99350-0. |
[8] |
Attia MEH, Zayed ME, Kabeel AE (2024) Design and performance optimization of a novel zigzag channeled solar photovoltaic thermal system: Numerical investigation and parametric analysis. J Clean Prod 434: 140220. https://doi.org/10.1016/j.jclepro.2023.140220 doi: 10.1016/j.jclepro.2023.140220
![]() |
[9] |
Bourisli RI, Zaher FA, Aldalali BS (2024) Reducing the operating temperature and improving the efficiency of PV modules using guiding vanes. Sol Energy 269: 112345. https://doi.org/10.1016/j.solener.2024.112345 doi: 10.1016/j.solener.2024.112345
![]() |
[10] |
Dhilipan J, Vijayalakshmi N, Shanmugam DB (2022) Performance and efficiency of different types of solar cell material—A review. Mater Today Proc 66: 1295–1302. https://doi.org/10.1016/j.matpr.2022.05.132 doi: 10.1016/j.matpr.2022.05.132
![]() |
[11] |
Dwivedi P, Sudhakar K, Soni A, et al. (2020) Advanced cooling techniques of PV modules: A state of art. Case Stud Therm Eng, 21. https://doi.org/10.1016/j.csite.2020.100674 doi: 10.1016/j.csite.2020.100674
![]() |
[12] |
Kazem HA, Al-Waeli AA, Chaichan MT, et al. (2023) Enhancement of photovoltaic module performance using passive cooling (Fins): A comprehensive review. Case Stud Therm Eng 49: 103316. https://doi.org/10.1016/j.csite.2023.103316 doi: 10.1016/j.csite.2023.103316
![]() |
[13] |
Maghrabie HM, Elsaid K, Sayed ET, et al. (2022) Phase change materials based on nanoparticles for enhancing the performance of solar photovoltaic panels: A review. J Energy Storage 48: 103937. https://doi.org/10.1016/j.est.2021.103937 doi: 10.1016/j.est.2021.103937
![]() |
[14] |
Tiwari S, Swaminathan M, Harender SES, et al. (2022) Performance enhancement of the photovoltaic system with different cooling methods. Environ Sci Pollut Res Int 29: 45107–45130. https://doi.org/10.1007/s11356-022-20330-x doi: 10.1007/s11356-022-20330-x
![]() |
[15] |
Sharaby MR, Younes M, Baz F, et al. (2024) State-of-the-Art review: Nanofluids for photovoltaic thermal systems. J Contemp Technol Aappl Eng 3: 11–24. https://doi.org/10.21608/jctae.2024.288445.1025 doi: 10.21608/jctae.2024.288445.1025
![]() |
[16] |
Sheik MA, Aravindan MK, Cuce E, et al. (2022) A comprehensive review on recent advancements in cooling of solar photovoltaic systems using phase change materials. Int J Low-Carbon Technol 17: 768–783. https://doi.org/10.1093/ijlct/ctac053 doi: 10.1093/ijlct/ctac053
![]() |
[17] | Solar radiation: What it is and how it is produced—Iberdrola. Accessed: Jun. 22, 2023. Available from: https://www.iberdrola.com/social-commitment/solar-radiation. |
[18] | Kumar Gupta A, Kakkar S, Chaudhary R, et al. (2021) Sun irradiance trappers for solar PV module to operate on maximum power: An experimental study. https://doi.org/10.17762/turcomat.v12i5.1759 |
[19] | Singh AK Singh RR (2021) An overview of factors influencing solar power efficiency and strategies for enhancing. 2021 Innovations Power Adv Comput Techno (i-PACT). https://doi.org/10.1109/i-PACT52855.2021.9696845 |
[20] |
Ramkiran B, Sundarabalan CK, Sudhakar K (2020) Performance evaluation of solar PV module with filters in an outdoor environment. Case Stud Therm Eng 21: 100700. https://doi.org/10.1016/j.csite.2020.100700 doi: 10.1016/j.csite.2020.100700
![]() |
[21] | Al-Maghalseh M (2018) Experimental study to investigate the effect of dust, wind speed and temperature on the PV module performance. Jordan J Mech Ind Eng 12: 123–129. |
[22] | Climate of Singapore (2024) Available from: https://www.weather.gov.sg/climate-climate-of-singapore/. |
[23] | Panjwani MK, Narejo GB (2014) Effect of humidity on the efficiency of solar cell (photovoltaic). Int J Eng Res Gen Sci, 2. |
[24] | Hosenuzzaman M, Hasanuzzaman M, Rahim NA, et al. (2014) Factors affecting the PV based power generation. 3rd IET International Conference on Clean Energy and Technology (CEAT) 2014, 20. https://doi.org/10.1049/cp.2014.1467 |
[25] |
Abderrezek M, Fathi M (2017) Experimental study of the dust effect on photovoltaic panels' energy yield. Sol Energy 142: 308–320. https://doi.org/10.1016/j.solener.2016.12.040 doi: 10.1016/j.solener.2016.12.040
![]() |
[26] |
Fouad MM, Shihata LA, Morgan ESI (2017) An integrated review of factors influencing the performance of photovoltaic panels. Renewable Sustainable Energy Rev 80: 1499–1511. https://doi.org/10.1016/j.rser.2017.05.141 doi: 10.1016/j.rser.2017.05.141
![]() |
[27] |
Kumar P, Shukla AK, Sudhakar K, et al. (2017) Experimental exergy analysis of water-cooled PV module. Int J Exergy 23: 197–209. https://doi.org/10.1504/IJEX.2017.085768 doi: 10.1504/IJEX.2017.085768
![]() |
[28] |
Bernadette D, Twizerimana M, Bakundukize A, et al. (2021) Analysis of shading effects in solar PV system. Int J Sustainable Green Energy 10: 47. https://doi.org/10.11648/j.ijrse.20211002.13 doi: 10.11648/j.ijrse.20211002.13
![]() |
[29] |
Bilen K, Erdoğan İ (2023) Effects of cooling on performance of photovoltaic/thermal (PV/T) solar panels: A comprehensive review. Sol Energy 262: 111829. https://doi.org/10.1016/j.solener.2023.111829 doi: 10.1016/j.solener.2023.111829
![]() |
[30] | Solanki R, Singh V, Varshney L, et al (2023) Advanced cooling technique to improve efficiency of Solar Panel. 2023 International Conference on IoT, Communication and Automation Technology (ICICAT), 1–6. https://doi.org/10.1109/ICICAT57735.2023.10263658 |
[31] |
Pomares-Hernández C, Zuluaga-García EA, Escorcia Salas GE, et al (2021) Computational modeling of passive and active cooling methods to improve PV panels efficiency. Appl Sci 11: 11370. https://doi.org/10.3390/app112311370 doi: 10.3390/app112311370
![]() |
[32] |
Ahmadi R, Monadinia F, Maleki M (2021) Passive/active photovoltaic-thermal (PVT) system implementing infiltrated phase change material (PCM) in PS-CNT foam. Sol Energy Mater Sol Cells 222: 110942. https://doi.org/10.1016/j.solmat.2020.110942 doi: 10.1016/j.solmat.2020.110942
![]() |
[33] |
Dida M, Boughali S, Bechki D, et al. (2021) Experimental investigation of a passive cooling system for photovoltaic modules efficiency improvement in hot and arid regions. Energy Convers Manage 243: 114328. https://doi.org/10.1016/j.enconman.2021.114328 doi: 10.1016/j.enconman.2021.114328
![]() |
[34] |
Ahmad EZ, Sopian K, Jarimiet H, et al. (2021) Recent advances in passive cooling methods for photovoltaic performance enhancement. Int J Electr Comput Eng 11: 146. https://doi.org/10.11591/ijece.v11i1.pp146-154 doi: 10.11591/ijece.v11i1.pp146-154
![]() |
[35] |
Rickric OG, Martes AS, Pagatpatan GI, et al. (2020) Hybrid cooling system for solar PV panel. Int J Emerging Trends Eng Res 8: 4079–4083. https://doi.org/10.30534/ijeter/2020/08882020 doi: 10.30534/ijeter/2020/08882020
![]() |
[36] |
Tiwari MK, Mishra V, Dev R, et al (2023) Effects of active cooling techniques to improve the overall efficiency of photovoltaic module—An updated review. E3S Web Conf 387: 01012. https://doi.org/10.1051/e3sconf/202338701012 doi: 10.1051/e3sconf/202338701012
![]() |
[37] |
Sharaf M, Yousef MS, Huzayyin AS (2022) Review of cooling techniques used to enhance the efficiency of photovoltaic power systems. Environ Sci Pollut Res Int 29: 26131–26159. https://doi.org/10.1007/s11356-022-18719-9 doi: 10.1007/s11356-022-18719-9
![]() |
[38] | Zohuri B (2016) Heat pipe design and technology: Modern applications for practical thermal managemen. Springer Cham. https://doi.org/10.1007/978-3-319-29841-2 |
[39] |
Basri MH, Yaakob Y, Kamaruzaman Z, et al. (2022) Heat pipe as a passive cooling device for PV panel performance enhancement. J Adv Res Appl Sci Eng Technol 28: 190–198. https://doi.org/10.37934/araset.28.2.190198 doi: 10.37934/araset.28.2.190198
![]() |
[40] | Eshghi H, Kahani M, Zamen M (2022) Cooling of photovoltaic panel equipped with single circular heat pipe: An experimental study. 3: 229–235. https://doi.org/10.22044/rera.2022.11523.1097 |
[41] |
Praveenkumar S, Gulakhmadov A, Agyekumet EB, et al. (2022) Experimental study on performance enhancement of a photovoltaic module incorporated with CPU heat pipe—A 5E analysis. Sensors, 22. https://doi.org/10.3390/s22176367 doi: 10.3390/s22176367
![]() |
[42] |
Al-Amri F, Maatallah TS, Al-Amri OF, et al. (2022) Innovative technique for achieving uniform temperatures across solar panels using heat pipes and liquid immersion cooling in the harsh climate in the Kingdom of Saudi Arabia. Alex Eng J 61: 1413–1424. https://doi.org/10.1016/j.aej.2021.06.046 doi: 10.1016/j.aej.2021.06.046
![]() |
[43] |
Kaneesamkandi Z, Almalki MJ, Sayeed A, et al. (2023) Passive cooling of PV modules using heat pipe thermosiphon with acetone: Experimental and theoretical study. Appl Sci 13: 3. https://doi.org/10.3390/app13031457 doi: 10.3390/app13031457
![]() |
[44] |
Sabry M, Lashin A (2023) Performance of a heat-pipe cooled concentrated photovoltaic/thermoelectric hybrid system. Energies 16: 1438. https://doi.org/10.3390/en16031438 doi: 10.3390/en16031438
![]() |
[45] |
Arifin Z, Tjahjana DDDP, Hadi S, et al. (2020) Numerical and experimental investigation of air cooling for photovoltaic panels using aluminum heat sinks. Int J Photoenergy. https://doi.org/10.1155/2020/1574274 doi: 10.1155/2020/1574274
![]() |
[46] |
Arifin Z, Suyitno S, Tjahjana DDDP, et al. (2020) The effect of heat sink properties on solar cell cooling systems. Appl Sci 10: 1–16. https://doi.org/10.3390/app10217919 doi: 10.3390/app10217919
![]() |
[47] |
Hudișteanu SV, Țurcanu FE, Cherecheș NC, et al. (2021) Enhancement of pv panel power production by passive cooling using heat sinks with perforated fins. Appl Sci 11: 23. https://doi.org/10.3390/app112311323 doi: 10.3390/app112311323
![]() |
[48] |
Salehi R, Jahanbakhshi A, Reza Golzarian M, et al. (2021) Evaluation of solar panel cooling systems using anodized heat sink equipped with thermoelectric module through the parameters of temperature, power and efficiency. Energy Convers Manage: X, 11. https://doi.org/10.1016/j.ecmx.2021.100102 doi: 10.1016/j.ecmx.2021.100102
![]() |
[49] |
Krstic M, Pantic L, Djordjevic S, et al. (2024) Passive cooling of photovoltaic panel by aluminum heat sinks and numerical simulation. Ain Shams Eng J, 15. https://doi.org/10.1016/j.asej.2023.102330 doi: 10.1016/j.asej.2023.102330
![]() |
[50] |
Elminshawy NAS, El-Damhogi DG, Ibrahim IA, et al. (2022) Assessment of floating photovoltaic productivity with fins-assisted passive cooling. Appl Energy 325: 119810. https://doi.org/10.1016/j.apenergy.2022.119810 doi: 10.1016/j.apenergy.2022.119810
![]() |
[51] |
Kirpichnikova IM, Sudhakar K, Makhsumov IB, et al. (2022) Thermal model of a photovoltaic module with heat-protective film. Case Stud Therm Eng, 30. https://doi.org/10.1016/j.csite.2021.101744 doi: 10.1016/j.csite.2021.101744
![]() |
[52] |
Yazdanifard F, Ameri M, Ebrahimnia-Bajestan E (2017) Performance of nanofluid-based photovoltaic/thermal systems: A review. Renewable Sustainable Energy Rev 76: 323–352. https://doi.org/10.1016/j.rser.2017.03.025 doi: 10.1016/j.rser.2017.03.025
![]() |
[53] |
Murtadha TK, Dil Hussein AA, Alalwany AAH, et al. (2022) Improving the cooling performance of photovoltaic panels by using two passes circulation of titanium dioxide nanofluid. Case Stud Therm Eng, 36. https://doi.org/10.1016/j.csite.2022.102191 doi: 10.1016/j.csite.2022.102191
![]() |
[54] |
Hamdan M (2022) Performance enhancement of a photovoltaic module by passive cooling using water-based aluminum oxide nano-fluid. J Ecol Eng 23: 276–286. https://doi.org/10.12911/22998993/146675 doi: 10.12911/22998993/146675
![]() |
[55] |
Ibrahim A, Ramadan MR, Khallaf AEM, et al. (2023) A comprehensive study for Al2O3 nanofluid cooling effect on the electrical and thermal properties of polycrystalline solar panels in outdoor conditions. Environ Sci Pollut Res Int 30: 106838–106859. https://doi.org/10.1007/s11356-023-25928-3 doi: 10.1007/s11356-023-25928-3
![]() |
[56] |
Jose JPA, Soni PK, Hemalatha N, et al. (2023) An analysis of the effects of nanofluid-based serpentine tube cooling enhancement in solar photovoltaic cells for green cities. J Nanomater 2023: 1–15. https://doi.org/10.1155/2023/3456536 doi: 10.1155/2023/3456536
![]() |
[57] |
Salehi R, Jahanbakhshi A, Ooi JB, et al. (2023) Study on the performance of solar cells cooled with heatsink and nanofluid added with aluminum nanoparticle. Int J Thermofluids, 20. https://doi.org/10.1016/j.ijft.2023.100445 doi: 10.1016/j.ijft.2023.100445
![]() |
[58] |
Abdollahi N, Rahimi M (2020) Potential of water natural circulation coupled with nano-enhanced PCM for PV module cooling. Renewable Energy 147: 302–309. https://doi.org/10.1016/j.renene.2019.09.002 doi: 10.1016/j.renene.2019.09.002
![]() |
[59] |
Velmurugan K, Karthikeyan V, Korukonda TB, et al. (2020) Experimental studies on PV module cooling with radiation source PCM matrix. IEEE Access 8: 145936–145949. https://doi.org/10.1109/ACCESS.2020.3012272 doi: 10.1109/ACCESS.2020.3012272
![]() |
[60] |
Rubaiee S, Fazal MA (2022) The influence of various solar radiations on the efficiency of a photovoltaic solar module integrated with a passive cooling system. Energies 15: 9584. https://doi.org/10.3390/en15249584 doi: 10.3390/en15249584
![]() |
[61] |
Agarwal N, Saxena A (2022) Performance characteristics of a photovoltaic panel integrated with PCM packed cylindrical tubes for cooling. Int J Sustainable Energy 41: 444–468. https://doi.org/10.1080/14786451.2021.1950721 doi: 10.1080/14786451.2021.1950721
![]() |
[62] |
Durez A, Ali M, Waqas A, et al. (2023) Modelling and optimization of phase change materials (PCM)-based passive cooling of solar PV panels in multi climate conditions. Front Energy Res, 11. https://doi.org/10.3389/fenrg.2023.1121138 doi: 10.3389/fenrg.2023.1121138
![]() |
[63] |
Sheikh Y, Jasim M, Qasim M, et al. (2024) Enhancing PV solar panel efficiency through integration with a passive Multi-layered PCMs cooling system: A numerical study. Int J Thermofluids 23: 100748. https://doi.org/10.1016/j.ijft.2024.100748 doi: 10.1016/j.ijft.2024.100748
![]() |
[64] |
Drebushchak VA (2008) Introduction the peltier effect. J Therm Anal Calorim 91: 311–315. https://doi.org/10.1007/s10973-007-8336-9 doi: 10.1007/s10973-007-8336-9
![]() |
[65] |
Metwally H, Mahmoud NA, Aboelsoud W, et al. (2021) Yearly performance of the photovoltaic active cooling system using the thermoelectric generator. Case Stud Therm Eng 27: 101252. https://doi.org/10.1016/j.csite.2021.101252 doi: 10.1016/j.csite.2021.101252
![]() |
[66] |
Khan MAI, Khan MI, Kazim AH, et al. (2021) An experimental and comparative performance evaluation of a hybrid photovoltaic-thermoelectric system. Front Energy Res, 9. https://doi.org/10.3389/fenrg.2021.722514 doi: 10.3389/fenrg.2021.722514
![]() |
[67] |
Praveenkumar S, Agyekum EB, Alwan NT, et al. (2022) Experimental assessment of thermoelectric cooling on the efficiency of PV module. Int J Renewable Energy Res 12: 1670–1681. https://doi.org/10.20508/ijrer.v12i3.13087.g8553 doi: 10.20508/ijrer.v12i3.13087.g8553
![]() |
[68] |
Faheem M, Abu Bakr M, Ali M, et al. (2024) Evaluation of efficiency enhancement in photovoltaic panels via integrated thermoelectric cooling and power generation. Energies 17: 2590. https://doi.org/10.3390/en17112590 doi: 10.3390/en17112590
![]() |
[69] |
Ramkiran B, Sundarabalan CK, Sudhakar K (2021) Sustainable passive cooling strategy for PV module: A comparative analysis. Case Stud Therm Eng, 27. https://doi.org/10.1016/j.csite.2021.101317 doi: 10.1016/j.csite.2021.101317
![]() |
[70] |
Dwivedi P, Ganesh SA, Sudhakar K (2023) Thermal and electrical performance of uncooled, nature-cooled, and photovoltaic thermal module. Int J Photoenergy. https://doi.org/10.1155/2023/4720545 doi: 10.1155/2023/4720545
![]() |
[71] |
Rukman, NS, Fudholi A, Utari PA, et al. (2021) Bi-fluid cooling effect on electrical characteristics of flexible photovoltaic panel. J Mechatron Electr Power Veh Technol 12: 51–56. https://doi.org/10.14203/j.mev.2021.v12.51-56 doi: 10.14203/j.mev.2021.v12.51-56
![]() |
[72] |
Agyekum EB, PraveenKumar S, Alwan NT (2021) Effect of dual surface cooling of solar photovoltaic panel on the efficiency of the module: Experimental investigation. Heliyon 7: e07920. https://doi.org/10.1016/j.heliyon.2021.e07920 doi: 10.1016/j.heliyon.2021.e07920
![]() |
[73] |
Chiang W, Permana I, Wang F, et al. (2022) Experimental investigation for an innovative hybrid photovoltaic/Thermal (PV/T) solar system. Energy Rep 8: 910–918. https://doi.org/10.1016/j.egyr.2022.10.264 doi: 10.1016/j.egyr.2022.10.264
![]() |
[74] |
Azmi MSFM, Hussain MH, Rahim SRA, et al. (2023) Hybrid cooling system for solar photovoltaic panel. J Phys Conf Ser 2550: 012004. https://doi.org/10.1088/1742-6596/2550/1/012004 doi: 10.1088/1742-6596/2550/1/012004
![]() |
[75] |
Madurai Elavarasan R, Nadarajah M, Pugazhendhi R, et al. (2024) An experimental investigation on coalescing the potentiality of PCM, fins and water to achieve sturdy cooling effect on PV panels. Appl Energy 356: 122371. https://doi.org/10.1016/j.apenergy.2023.122371 doi: 10.1016/j.apenergy.2023.122371
![]() |
[76] | Said Z, Ahmad FF, Radwan AM, et al. (2023) New thermal management technique for PV module using Mist/PCM/Husk: An experimental study. 401: 136798. https://doi.org/10.1016/j.jclepro.2023.136798 |
[77] |
Kumar R, Montero FJ, Lamba R, et al. (2023) Thermal management of photovoltaic-thermoelectric generator hybrid system using radiative cooling and heat pipe. Appl Therm Eng 227: 120420. https://doi.org/10.1016/j.applthermaleng.2023.120420 doi: 10.1016/j.applthermaleng.2023.120420
![]() |
[78] |
Hamed MH, Hassan H, Ookawara S, et al. (2024) Performance comparative study on passively cooled concentrated photovoltaic (PV) using adsorption/desorption and heat sink cooling methods: Experimental investigations. Energy 307: 132700. https://doi.org/10.1016/j.energy.2024.132700 doi: 10.1016/j.energy.2024.132700
![]() |
1. | Chengzhi Yan, Chen Wang, Jian Shen, Kapal Dev, Mohsen Guizani, Weizheng Wang, Edge-Assisted Hierarchical Batch Authentication Scheme for VANETs, 2024, 73, 0018-9545, 1253, 10.1109/TVT.2023.3305556 | |
2. | S. Milton Ganesh, S. Priya, Vinayakumar Ravi, Suliman A. Alsuhibany, A privacy preserving batch audit scheme for IoT based cloud data storage, 2025, 18, 1936-6442, 10.1007/s12083-024-01890-w | |
3. | Cherif Ghazel, Imen Merdassi, Leila Saidane, An efficient mobile cloud security method based on strong multi-factor authentication and smart card technology, 2025, 0003-4347, 10.1007/s12243-025-01076-2 |
Entity Side | Computational Cost |
Client | (2z+4)TE+(3+z)TMul.+zTH.+1TAdd.+TP. |
Authority | ρ(2TMul.+TE.+TAdd.) |
Server | (4+ρ)TE.+(2ρ+3)TMul.+ρTH.+TP. |
*TE.,TH.,TMul.,TAdd.,TP.: Time required to perform the corresponding operations.
*ρ: The number of the challenged data blocks. *z: The total number of data blocks. |
Phase | Computational Cost |
Authentication | 7TE+5TMul.+1TAdd. |
Storage Checking | (2z+2ρ+1)TE.+(z+3ρ+1)TMul.+(z+ρ)TH.+ρTAdd.+2TP. |
*TE.,TH.,TMul.,TAdd.,TP.: Time required to perform the corresponding operations.
*ρ: The number of the challenged data blocks. *z: The total number of data blocks. |
Phase | Computational Cost | ||
Authentication | 3lG+1lZ | ||
Storage Checking | (4ρ+z+2)lZ | ||
*lG,lZ: Time required to perform the corresponding operations.
*ρ: The number of the challenged data blocks. *z: The total number of data blocks. |
Entity Side | Computational Cost |
Client | (2z+4)TE+(3+z)TMul.+zTH.+1TAdd.+TP. |
Authority | ρ(2TMul.+TE.+TAdd.) |
Server | (4+ρ)TE.+(2ρ+3)TMul.+ρTH.+TP. |
*TE.,TH.,TMul.,TAdd.,TP.: Time required to perform the corresponding operations.
*ρ: The number of the challenged data blocks. *z: The total number of data blocks. |
Phase | Computational Cost |
Authentication | 7TE+5TMul.+1TAdd. |
Storage Checking | (2z+2ρ+1)TE.+(z+3ρ+1)TMul.+(z+ρ)TH.+ρTAdd.+2TP. |
*TE.,TH.,TMul.,TAdd.,TP.: Time required to perform the corresponding operations.
*ρ: The number of the challenged data blocks. *z: The total number of data blocks. |
Phase | Computational Cost | ||
Authentication | 3lG+1lZ | ||
Storage Checking | (4ρ+z+2)lZ | ||
*lG,lZ: Time required to perform the corresponding operations.
*ρ: The number of the challenged data blocks. *z: The total number of data blocks. |