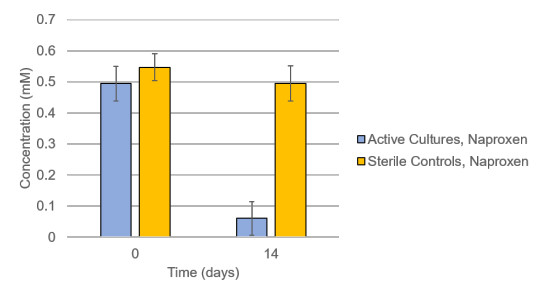
Citation: Abigail W. Porter, Sarah J. Wolfson, Lily. Young. Pharmaceutical transforming microbes from wastewater and natural environments can colonize microplastics[J]. AIMS Environmental Science, 2020, 7(1): 99-116. doi: 10.3934/environsci.2020006
The ubiquity of plastics in our environment extends to municipal waste treatment systems. Treated wastewater effluents were identified as one source of environmental microplastic deposition in freshwater environments [1]. These wastewater effluents also contain low concentrations of pharmaceuticals, personal care product constituents, and their transformation products that are not completely removed during the wastewater treatment process [2]. Thus, microplastic particles are in contact with a mixture of pharmaceutical and personal care products prior to release into the environment [3]. Furthermore, microplastics serve as a mechanism for transporting persistent organic pollutants [4], as well as microbes in the environment [5,6]. The interaction between microorganisms and common wastewater contaminants, including pharmaceuticals and personal care products, that may be occurring on the microplastic surface is less understood. Microplastics carrying microorganisms and wastewater contaminants eventually enter anoxic sediments since colonization by microorganisms can lead to decreased microplastic buoyancy [7,8]. Therefore, understanding the potential for biodegradation functions to be transported on microplastics can provide a mechanism for transforming pollutants that are associated with wastewater in downstream receiving waters and their environments.
As a widely used over-the-counter non-steroidal anti-inflammatory medication, naproxen is a frequent wastewater contaminant and is found at detectable levels in the environment [9]. We have previously shown that naproxen transformation occurs through O-demethylation by an anaerobic digester consortium and a highly enriched culture from estuarine sediment [10,11]. Using naproxen as a model, the current study examined pharmaceutical transformation by microplastics-associated biofilms that were incubated under methanogenic conditions. Both anaerobic digester sludge and urban-impacted freshwater sediment were used as inocula, to represent the microbial communities that would interact with the microplastics during wastewater treatment and those that would be in downstream environments.
We hypothesize that accumulated contaminants, naproxen in our case, on the microplastic surface will serve as a carbon source for microorganisms. This will create a microhabitat that selects for microorganisms capable of transforming contaminants. The microplastics would then carry that biofilm as it is transported from wastewater treatment into downstream environments, thus introducing the biofilm microorganisms into new environments. The work presented here demonstrates that the naproxen-transforming activity can be transported potentially from wastewater treatment into the receiving waters and within urban impacted sediments by the biofilm community present on microplastics.
Polyethylene microplastic beads were collected and separated from a consumer facial scrub by filtering through a coffee filter and a series of washes with first water, then 95% ethanol followed by 50% methanol, to remove soap and other additives. Microplastic beads were reported to range in size from 120–600 µm [12]. Washed microplastic beads were added to a sterile serum bottle and mixed with 64 mg naproxen sodium (Sigma-Aldrich, St. Louis, MO) per 1 g microplastic beads. The naproxen was dissolved in 5 mL methanol to completely cover the microplastic beads and allow contact between the dissolved naproxen and the microplastic surface. The serum bottle was flushed with N2 for several minutes until the methanol was completely evaporated, leaving behind microplastic beads that were coated with naproxen.
To determine the amount of naproxen that would remain associated with the microplastic beads when placed in an aqueous environment, abiotic experiments were established to monitor the concentration of naproxen on microplastics and in the background medium. Artificial wastewater used to mimic in situ conditions was made as previously described [13] under anaerobic conditions. Naproxen-coated microplastic beads described above were added to sterile artificial wastewater in serum bottles sealed with butyl rubber stoppers and aluminum crimps. To quantify naproxen concentration, samples were taken periodically using anaerobic techniques. The serum bottles were shaken to keep microplastic particles suspended in the artificial wastewater and allow uniform microplastic bead recovery with each sample. Then 0.5 mL was removed through the butyl rubber stopper using a plastic 1 mL syringe fitted with a 20-gauge needle to allow sampling of microplastic beads. The sample was placed into a 0.2 µm Spin-X nylon filter (Corning Costar, Corning, NY) and centrifuged to separate the aqueous dissolved naproxen fraction from the naproxen-sorbed microplastic fraction. The aqueous filtrate was transferred to a new vial and mixed with an equal volume of methanol to dissolve any insoluble particles. Separately, 0.5 mL methanol was added to the microplastic beads collected on the nylon filter and incubated at room temperature for 30 minutes to dissolve any naproxen that had adsorbed to the microplastic. The methanol extract, now containing dissolved naproxen, was filtered through a 0.2 µm Spin-X nylon filter to remove the microplastic particles. The filtrate was mixed with sterile artificial wastewater to mimic the conditions of the samples prepared from the aqueous fraction. Naproxen concentrations were quantified by HPLC as described below using authentic standards. The results of the separate fractions showed the amount of naproxen that was dissolved in the medium and the amount that was remained associated with the microplastic beads over time.
Anaerobic digester sludge was obtained from the Joint Meeting of Union and Essex Counties municipal wastewater treatment facility (Elizabeth, NJ) and stored for 24 hours at 35 ℃ prior to use. A 20% vol/vol mixture of anaerobic digester sludge and methanogenic medium [14] was stirred under a stream of 30% CO2: 70% N2 and dispensed in 40 mL aliquots into glass serum bottles. Naproxen-coated microplastic beads were prepared as described above and dispensed into cultures under a stream of 30% CO2: 70% N2. Each culture received 78.8 mg microplastic beads, calculated to contain 5.04 mg naproxen for a final concentration of 0.5 mM in each 40 mL culture. Triplicate active cultures and duplicate sterile controls received naproxen-coated microplastic beads, with sterile controls autoclaved daily for three consecutive days prior to the addition of the microplastic beads. Additionally, background controls, which contained 78.8 mg sterile microplastic beads but lacked naproxen, were also established. Cultures were sealed with butyl rubber stoppers and aluminum crimps and incubated at 35 ℃ in the dark. Samples were taken periodically as described below and naproxen was quantified by HPLC with authentic naproxen standards. Cultures were reamended after 30 days with 0.5 mM additional naproxen-coated microplastic beads. A subsequent transfer into an equal volume of fresh media and an additional naproxen-coated beads amendment was necessary to maintain the cultures.
Anaerobic sediment was collected from the Raritan River in Highland Park, NJ, during low tide and stored under an overlay of river water at 4 ℃ for 7 days before establishing enrichment cultures. A slurry of 20% vol/vol sediment in methanogenic medium was dispensed under 30% CO2: 70% N2 into sealed serum bottles and amended with naproxen-coated microplastic beads as described above. There were triplicate active cultures and two sterile controls that were autoclaved on three consecutive days. Additional background controls, which contained sterile microplastic beads that had not been treated with naproxen, were also included. Raritan River cultures were incubated at room temperature in the dark. These cultures were sampled as described below and analyzed by HPLC to determine naproxen concentrations. Cultures were reamended with naproxen-coated microplastic beads when the naproxen had completely disappeared.
To determine the naproxen-transformation capacity associated with the microplastic community and the planktonic community, microplastic and the planktonic fractions of the primary enrichments were transferred separately to new media. Avoiding the sludge or sediment from the inoculum, the microplastic beads were carefully removed from the culture with a sterilized metal spatula under a stream of 30% CO2: 70% N2 and transferred to serum bottles containing 10 mL sterile methanogenic media amended with neat naproxen. The serum bottles were sealed with butyl rubber stoppers and aluminum crimps and designated as microplastics transfer cultures. Planktonic phase transfer cultures consisted of equal volumes of fresh methanogenic media mixed with the liquid portion of the original enrichment culture. The culture was transferred using anaerobic techniques under 30% CO2: 70% N2, carefully avoiding microplastic particles by using 21-gauge needles that would be too small in diameter to allow microplastics to pass through. The planktonic transfer cultures were amended with neat naproxen. The same procedures were used to transfer naproxen-free microplastic beads and planktonic cultures from bottles designated as background controls in the primary enrichments. Samples were taken as described below and analyzed by HPLC to monitor naproxen loss.
Cultures were sampled periodically using strict anaerobic techniques to monitor naproxen loss and metabolite formation. Culture bottles were shaken well to uniformly sample suspended microplastic beads and 0.5 mL was removed under anaerobic conditions with a plastic syringe and 20-gauge needle (large enough to collect beads). Samples were put in a plastic vial. The syringe was rinsed with 0.5 mL 100% methanol to recover any remaining microplastic beads. The syringe plunger was submerged in this methanol for 15 minutes to extract any residual naproxen from microplastic beads that may have remained associated with the plunger. The methanol extract was then mixed with the sample in the vial for 15 minutes to dissolve any naproxen present in the entire sample. To prepare the sample for chemical analysis, it was then filtered through a 0.2 µm Spin-X nylon filter. The filtrate was analyzed by HPLC equipped with a UV-VIS detector (Shimadzu, Columbia, MD) and a C-18 reverse phase column (Phenomenex, Torrance, CA). Naproxen (Sigma-Aldrich) and 6-O-desmethylnaproxen (DMN) were detected at 280 nm using a mobile phase of 70% methanol: 28% water: 2% glacial acetic acid at a flow rate of 1 mL min−1. All solvents were HPLC-grade and purchased from Thermo Fisher Scientific (Waltham, MA, USA). The 6-O-desmethylnaproxen was synthesized as previously described [10].
The volume of excess headspace gas was measured using a degassed glass syringe fitted with an 18 gauge needle as described by Owen et al. [15]. The methane content present in the headspace was measured by injecting a sample of 0.5 mL headspace gas using a Pressure-Lok gas-tight syringe into a Fischer Hamilton 1200 gas partitioner with a 3.35 m x 4.76 mm column packed with 80/100 mesh PoraPak Q (Supelco, Bellefonte, PA). Methane production was calculated based on the standard curves generated from calibrated gases (Matheson Tri-Gas, Montgomeryville, PA).
Microplastic beads from replicate culture bottles were removed under a stream of 30% CO2: 70% N2 and pooled to reach a total of 0.3 mL of microplastic beads. These were gently rinsed in a vial of Solution 1 (25 mM Tris-Cl (pH 8), 10 mM EDTA, and 50 mM glucose) to remove any unattached cells before being transferred to a new vial and immediately frozen. Liquid samples from the planktonic transfer cultures were filtered through a 0.22 µm Spin-X nylon filter. The filter membrane was excised and frozen immediately. DNA was extracted from anaerobic digester samples using a modified phenol and chloroform method [16] with cetyl trimethylammonium bromide (CTAB). Raritan River samples were extracted in a similar fashion, although potential inhibitors were removed using a Nucleo-Spin Soil DNA extraction kit (Macherey-Nagel, Bethlehem, PA).
Barcoded amplicon sequencing was done for the V4 region of the 16S rRNA gene using the primers 515 F (5'-GTGYCAGCMGCCGCGGTAA-3') and 806 R (5'-RGGACTACNVGGGTWTCTAAT-3') with a barcode on the forward primer. The PCR was performed with HotStarTaq Plus Master Mix Kit (Qiagen, USA) under the following conditions: 94 ℃ for 3 minutes, followed by 30 cycles of 94 ℃ for 30 seconds, 53 ℃ for 40 seconds and 72 ℃ for 1 minute, followed by a final elongation step at 72 ℃ for 5 minutes. PCR products were pooled prior to preparing an Illumina DNA library. Sequencing was performed at MR DNA (Shallowater, TX, USA) on an Illumina MiSeq following the manufacturer's guidelines.
Sequence data were processed using MR DNA analysis pipeline (MR DNA, Shallowater, TX, USA). Briefly, sequences were joined, then barcodes, sequences less than 150 bp, and sequences with ambiguous base calls were removed. Sequences were then denoised and chimeras were removed. Operational taxonomic units (OTUs) were defined by clustering at 97% similarity and were taxonomically classified using BLASTn against a curated database derived from RDPII [17] and NCBI [18]. The nucleotide sequence data are available in the National Center for Biotechnology Information Sequence Read Archive under the BioSample accession numbers SAMN12861440, SAMN12861441, SAMN12861442, SAMN12861443, SAMN12861444, and SAMN12861445.
To test the amount of naproxen that would associate with microplastics, methanol washed microplastic beads were coated with naproxen and incubated in synthetic wastewater. Initial samples showed that naproxen desorption from the microplastic into the wastewater medium occurred within the time required to establish the experimental bottles and remove samples, yet 0.1 mM remained associated with the microplastics (Figure S1). Soluble and microplastic-associated naproxen reached equilibrium quickly and the concentrations did not change over the 7-day monitoring period. Therefore, while there may be soluble naproxen in the bulk liquid, a substantial portion remained adsorbed to the uncolonized microplastic beads and could serve as a source of carbon for any biofilms that later form.
Anaerobic digester enrichments (AD) and Raritan River (RR) enrichments under methanogenic conditions and amended with microplastic beads both showed naproxen loss. In Figure 1, AD cultures exhibited 0.43 mM (±0.0015) naproxen loss within 14 days in the primary enrichment. The metabolite 6-O-desmethylnaproxen (DMN) was not quantified in primary enrichments, although previous naproxen-transforming cultures inoculated with anaerobic digester sludge consistently produced DMN. DMN was not further transformed in those cultures [10].
RR primary enrichments were monitored for both naproxen loss and DMN metabolite formation. Naproxen O-demethylation to DMN is shown in Figure 2A. Figure 2B represents naproxen transformation by the RR enrichments, with complete disappearance of 0.64 mM (±0.042) naproxen (blue bars) within 20 days. DMN (green bars) concentrations increased by 0.70 mM (±0.035) within 20 days. The amount of naproxen disappearance and DMN formation is stoichiometric and demonstrates that naproxen is directly transformed into DMN as previously reported [10]. This indicates that further degradation is not occurring in RR enrichments within the timeframe during which the cultures were monitored. These results are consistent with our previous findings for both methanogenic anaerobic digester cultures and sulfate-rich marine consortia, in which naproxen was microbially transformed through a specific O-demethylation step forming DMN as a product that did not disappear with extended incubations [10,11]. Both AD and RR enrichment cultures were re-amended with additional naproxen-coated microplastic beads and naproxen loss continued. The presence of microplastic beads did not inhibit naproxen biotransformation in primary enrichment cultures.
To test our hypothesis that naproxen-transforming microbial communities are being selectively enriched using the naproxen sorbed to the microplastic beads, we investigated whether naproxen transformation activity was localized on the microplastic surface. First, the microplastic particles were separated from the bulk liquid culture. Then, the microplastic fraction and planktonic liquid culture were separately transferred to sterile media containing naproxen. Figure 3A shows naproxen transformation by AD microplastic-amended cultures. By Day 32, the active cultures showed 1.76 mM (±0.87) naproxen loss, which completely disappeared in one of the three replicates by Day 39. In addition, DMN was detected in these cultures by Day 32 and reached concentrations of 0.84 mM (±0.14) by the end of the incubation. The DMN yield corresponded to 36% of the naproxen added to these cultures, but is consistent with the decreased recovery in anaerobic digester sludge cultures [10,19]. No loss of naproxen was noted in the sterile controls, nor was any DMN detected.
In contrast, the AD planktonic cultures in Figure 3B did not show naproxen loss within the 39-day monitoring period. There was a basal level of DMN detected in all the bottles as a result of unmetabolized DMN that was present in the planktonic fraction from the metabolism of previous naproxen amendments. While there does appear to be a slightly decreasing trend in naproxen concentration for both the active cultures and sterile controls, DMN did not increase. In fact, the DMN also slightly decreased in these cultures and is likely due to the methods used for sample preparation. At Day 39, however, sterile controls showed loss of naproxen and DMN due to, instrumentation errors for those specific samples. These results show that naproxen metabolism is largely mediated by the microorganisms associated with the microplastics while those present in the planktonic phase of the culture showed little activity in anaerobic digester enrichments. It is important to note that the AD primary enrichment cultures had been maintained for a long period of time, which may have encouraged the increased localization of naproxen-transforming microbes on microplastic beads.
Unlike the anaerobic digester enrichments, the freshwater RR cultures showed a similar extent of naproxen transformation by both microplastic-associated and planktonic communities. Figures 4A and 4B show the loss of naproxen and DMN formation in microplastic and the planktonic phase, respectively. Both RR treatments showed complete naproxen loss and the equivalent formation of DMN within 14 days. Upon reamendment at Day 17, naproxen again rapidly disappeared and the amount of DMN further increased by Day 29. Both amendments of naproxen were less than the starting concentration in the AD cultures, thus the direct comparison of these cultures may be misleading. The total amount of naproxen transformed was 0.78 mM (±0.093) and 0.75 mM (±0.067) for the microplastic and planktonic communities, respectively. The corresponding DMN formation was 0.78 mM (±0.092) by the microplastic community and 0.63 mM (±0.13) by the planktonic community. While there was some inconsistency in naproxen concentration observed in the sterile controls, especially in the planktonic community presented in Figure 4B, DMN was never detected in the controls. Variations in the naproxen concentration were likely due to the sample preparation as described for the AD cultures above. Naproxen concentrations were higher in the planktonic transfer sterile control cultures, due to the carryover of unmetabolized naproxen from the original culture bottles.
One difference between the RR planktonic and microplastic cultures, however, can be observed in the ratio of between naproxen demethylation and methane formation (Table 1). The per mole formation of methane was 3-fold higher in microplastic cultures than was detected in planktonic cultures. This suggests that although the demethylation process was similar in both cultures, there are differences in the microbial community composition that are affecting methane production.
Microplastic-Amended Cultures | Naproxen | DMN | Methane |
Active Cultures | 7.91 ± 0.916 | 8.21 ± 0.924 | 5.38 ± 2.41 |
Sterile Controls | n.d. | n.d. | 1.45 ± 0.131 |
Background Controls | 0.0313 ± 0.024 | 0.0285 ± 0.021 | 3.00 ± 0.281 |
Planktonic Cultures | Naproxen | DMN | Methane |
Active Cultures | 7.55 ± 0.653 | 7.79 ± 0.24 | 1.65 ± 1.349 |
Sterile Controls | n.d. | n.d. | n.d. |
Background Controls | 0.0485 ± 0.0912 | 0.0158 ± 0.00115 | 3.32 ± 0.471 |
An analysis of the 16S rRNA gene found the number of archaeal sequences was much lower than the number of sequences for bacteria (Table S1). Figures 5A and 5B illustrate the percent family distributions for total archaeal sequences and total bacterial sequences, respectively, while the sequence distribution at the genus-level can be found in Figures S2A and S2B. As seen in Figure 5A, naproxen exposure substantially affected the archaeal community composition of hydrogenotrophic and acetoclastic methanogens in anaerobic digester cultures. The microplastic-associated archaeal community in unamended cultures was dominated by Methanomicrobiaceae and Methanosaetaceae, at 49% and 50%, respectively. Methanomicrobiaceae were also highly represented in naproxen-amended microplastics cultures at 46% and 34% of the sequences in the microplastics-associated community and planktonic community, respectively. In contrast, the acetoclastic methanogens Methanosaetaceae were only 15% of the archaeal community attached to microplastics and further reduced to 6.5% of the sequences in the planktonic community. Furthermore, Methanobacteriaceae sequences were greatly enriched in the naproxen-treated cultures, representing 37% of the total archaeal sequences in both planktonic and microplastics communities, although this family was less than 0.5% of the total sequences in the unamended microplastics culture. While the overall abundance of the family Methanobacteriaceae was the same in both naproxen amended cultures, the composition at the genus-level was different. DNA sequences with similarity to Methanobacterium were 10-fold greater than those related to Methanobrevibacter in microplastics cultures, whereas the Methanobacterium were 2-fold greater than the Methanobrevibacter sequences in the planktonic culture (Figure S2A). In the cultures containing naproxen, the H2-utilizing families Methanomicrobiaceae and Methanobacteriaceae [20] represented 84% of the total Archaea in the microplastics cultures and 70% in the planktonic cultures. This distribution is similar to what has been previously reported for a naproxen-transforming consortium enriched from anaerobic digester sludge, in which Methanomicrobiaceae and Methanobacteriaceae together comprised nearly 75% of the total sequences and the Methanosaetaceae were less abundant [10]. The change in the archaeal composition reflects differences in the metabolic function of the methanogens. Neither Methanomicrobiaceae nor Methanobacteriaceae are able to use acetate, yet the Methanosaetaceae can [20]. Therefore, the enrichment of hydrogenotrophic methanogens, specifically Methanomicrobiaceae and Methanobacteriaceae, in naproxen-amended cultures supports a model in which naproxen O-demethylation generates acetate that is not directly used as a substrate for methane production.
Figure 5B shows the relative abundance of bacterial sequences in the AD microplastic-associated and planktonic communities. The Desulfobacteraceae, specifically the genus Desulfofaba (Figure S2B), were highly enriched in the microplastics community, representing 42% of the total sequences associated with microplastic beads and less than 1% of the planktonic community. Desulfobacteraceae have been associated with polyethylene terephthalate biofilms in marine environments [21] and are capable of fermentative growth [22]. While this family was also detected in the microplastics controls without naproxen, the abundance was only 5.9%.
Previous work showed that naproxen demethylation involved both acetogens and syntrophic acetate-oxidizing bacteria [10]. Spirochaetaceae, which contains members that are acetogens [23], are equally represented in both the planktonic and microplastics communities. Similarly, these naproxen-amended treatments shared an equal abundance of sequences that were related to the genus Syntrophaceticus that was less than 0.05% of the sequences in the unamended control (Figure S2B). The detection of Syntrophaceticus is notable, as this genus has syntrophic acetate-oxidizing members [24]. Families containing syntrophic bacteria, including Syntrophaceae (17%) and Syntrophomonadaceae (5.5%), were enriched in the naproxen-amended microplastics community, whereas these families represented 4.6 and 0.27%, respectively, of the planktonic community. The unamended microplastic cultures, however, were highly enriched in the family Syntrophaceae, which represented 66% of the total bacterial sequences. This could suggest that the syntrophic bacteria are associated with the microplastic surface to be in close proximity to the methanogens and may explain the lack of naproxen demethylation by the planktonic community.
As seen in the archaeal community composition displayed in Figure 6A and Figure S3A, the planktonic and microplastic communities from a freshwater enrichment culture showed differences in the abundance of hydrogenotrophic and acetoclastic methanogens. The naproxen-amended microplastics-associated community was enriched in the families Methanomicrobiaceae (19%), Methanoregulaceae (38%), Methanospirillaceae (5.1%), Methermicoccaceae (5.2%), and Methanocalculaceae (4.6%). These same families were also enriched in the unamended microplastics community, although the relative abundances are different. The planktonic community was unique in that it was enriched in acetoclastic methanogens Methanosaetaceae (21%) and Methanosarcinaceae (5.3%), which had an abundance of only 4.1 and 0.39%, respectively, in the naproxen-amended microplastics-associated community. This suggests that the addition of microplastics to these freshwater communities provides conditions that allow the enrichment of hydrogenotrophic methanogens. With respect to naproxen transformation, the acetoclastic methanogens in the planktonic community may be directly using the acetate produced through O-demethylation, whereas the microplastics community involves syntrophic acetate oxidation to provide H2 for the hydrogenotrophic methanogens.
This is consistent with the bacterial community composition shown in Figure 6B and Figure S3B. Both the naproxen-amended microplastics and planktonic communities are composed of families that contain acetogens. The Eubacteriaceae, specifically Acetobacterium, was more highly represented in the planktonic community (43%) than the microplastics community (23%), yet this family was less than 1% of the bacterial sequences in the unamended control. Acetobacterium dehalogenans is an acetogen and the biochemistry of O-demethylation in this organism has been well-characterized [25]. Clostridiaceae, a family that also contains members that are acetogens [26], was also enriched in the planktonic community (7.3%) relative to the microplastics community (3.4%) and was less than 1% of the sequences in the unamended control. Syntrophic bacteria, however, were enriched in both naproxen-amended and unamended microplastics cultures, which is in agreement with the enrichment of hydrogenotrophic methanogens in these cultures. The family Syntrophaceae, including genera Smithella and Syntrophus, were 8.7% of the naproxen-amended microplastics community and 17% of the unamended microplastics control, yet represented less than 1% of the planktonic community. While both microplastics and planktonic communities showed similar naproxen transformation activity, the DNA sequence data show different microbial communities and support different models for the food web involved in naproxen demethylation under methanogenic conditions.
Microorganisms colonize surfaces in both natural and engineered environments. Biofilm communities, such as those found in membrane bioreactors, may be useful for removing organic micropollutants during wastewater treatment. Different types of matrices have been used to test the extent of removal [27] including plastic substrates [28,29], therefore identifying microplastics-associated biofilms that are able to transform wastewater contaminants is not surprising. Some micropollutants adsorb to microplastic surfaces, while there may be an accumulation of micropollutants within the polysaccharide matrix that surrounds the biofilms [30,31], and thus provide an available source of carbon for those microorganisms. Our results demonstrated that naproxen could sorb to the polyethylene microplastic beads and be one such carbon source.
Our previous work, which provided the foundation for this study, elucidated how an anaerobic digester community utilized the methyl group of naproxen as a carbon and energy source [10]. In those cultures, naproxen was provided as the sole carbon and electron source. Consistent methane generation of 0.8 mol per mol naproxen demonstrated that the one methyl carbon removed from naproxen does transfer through the community to the final metabolite methane [10]. This is similar to the proportion of methane generated from naproxen transformation by the RR microplastics-associated community. Methanogenesis is an energy-generating metabolism indicative of biosynthesis [32], however, methanogens are unable to directly remove the methyl carbon from naproxen. A model based upon 16S rRNA gene analysis demonstrated that the bacteria enriched in naproxen O-demethylating cultures were acetogens, meaning that they generate energy by synthesizing acetate from the methyl carbon of naproxen [10]. The acetate excreted by these bacteria is then free to be utilized by the community. A portion of this ends up being degraded to appropriate substrates for methanogens, thus the difference between the amount of methane produced and the amount of naproxen that was O-demethylated [10].
The findings presented here clearly show that naproxen-transformation is associated with the microbial community that colonized microplastic in both AD and RR enrichment cultures. Naproxen transformation was associated with microplastic in the AD cultures and was not observed in the planktonic community. The microbial community analysis of the microplastics-associated cultures supports a food web that follows the model described above. The AD microplastics-associated community was enriched in families that contain acetogens and would facilitate the O-demethylation process, as well as syntrophic acetate-oxidizing bacteria to utilize the acetate and provide H2 for hydrogenotrophic methanogens. In contrast, while the RR microplastics and planktonic cultures continued to demonstrate naproxen loss in RR enrichments, the RR microplastics community produced more methane. The RR microplastics-associated community was enriched with the types of microorganisms in the AD microplastics-associated community, yet the RR planktonic community showed a decreased abundance of syntrophic bacteria and was enriched in acetoclastic methanogens. The acetoclastic methanogens may not be as competitive for H2, thus resulting in decreased methane produced by the planktonic cultures [33]. These findings provide evidence that the microplastic may have provided a surface to enable biofilm formation, which would benefit syntrophic bacteria and hydrogenotrophic methanogens by allowing direct contact between the cells to enable the more efficient transfer of H2 between these organisms [34]. These communities could potentially demethylate other pharmaceutical or personal care products that contain a similar methyl ether moiety [11], thus expanding the range for microbial transformations linked to microplastic.
Most previous work has approached microplastic from a purely biological or chemical context by characterizing just the microbial community or focusing solely on chemical interactions, respectively. Others have studied at the microbial community on different types of plastic [35], individual organisms of specific interest, such as pathogens [36], or functions like antibiotic resistance that would be expected in an individual organism [37]. In contrast, the work shown here is a community-based approach to investigate a microbial food web. In this context, microplastic pollution may have an unintentional benefit by mobilizing useful microbial communities to new locations, potentially filling a needed metabolic niche to consume carbon that is available in pharmaceutical contaminants. Furthermore, contaminants like hexadecane or terephthalate are degraded by syntrophic communities under methanogenic conditions, which are the same types of microorganisms that we identified in the microplastic-associated communities [38,39].
Microplastic pollution is heterogeneous in particle size and type, so the composition of biofilms that form on these particles will be varied [35]. This study focused specifically on naproxen biotransformation under methanogenic conditions with a single type of microplastic in order to best understand differences between the planktonic and biofilm communities. Further research is necessary with less soluble compounds to better understand the transfer of the organic carbon sources to the biofilm microorganisms and the potential for biodegradation or biotransformation under anaerobic conditions.
This work uses a model system to examine the transformation of a single pharmaceutical in the presence of a specific type of plastic under one redox condition. Even in cases where there is a relatively soluble substrate, as with naproxen, some of it will associate with the plastic particle and serve as an available source of carbon to anaerobic biofilm communities. With more hydrophobic wastewater contaminants, including detergent metabolites or plasticizers, the substrate may not be available in the bulk liquid at all, but rather may be completely associated with the microplastic surface. This could have implications for the bioavailability to the microorganisms, as it may now provide a larger surface area for microbial biotransformation. This work also demonstrates that microplastic-associated microbial communities can be transferred with microplastic particles, thereby presenting a model for pharmaceutical transformation activity to be transported from wastewater treatment facilities into the environment.
The authors wish to thank J. Mendez for Raritan River sediment samples. This work was supported by the USDA National Institute of Food and Agriculture Hatch Multistate project 1007899 through the New Jersey Agricultural Experiment Station Hatch Multistate NJ07212. S.J.W. was supported by a U.S. National Science Foundation Fuels IGERT (NSF DGE 0903675) from Rutgers, the State University of New Jersey.
All authors declare no conflicts of interest.
[1] |
Mason SA, Garneau D, Sutton R, et al. (2016) Microplastic pollution is widely detected in US municipal wastewater treatment plant effluent. Environ Pollut 218: 1045-1054. doi: 10.1016/j.envpol.2016.08.056
![]() |
[2] | Kolpin DW, Furlong ET, Meyer MT, et al. (2002) Pharmaceuticals, hormones, and other organic wastewater contaminants in U.S. Streams, 1999-2000: a national reconnaissance. Environ Sci Technol 36: 1202-1211. |
[3] |
Wu C, Zhang K, Huang X, et al. (2016) Sorption of pharmaceuticals and personal care products to polyethylene debris. Environ Sci Pollut Res 23: 8819-8826. doi: 10.1007/s11356-016-6121-7
![]() |
[4] |
Bakir A, Rowland SJ, Thompson RC (2014) Transport of persistent organic pollutants by microplastics in estuarine conditions. Estuar Coast Shelf Sci 140: 14-21. doi: 10.1016/j.ecss.2014.01.004
![]() |
[5] |
Zettler ER, Mincer TJ, Amaral-Zettler LA (2013) Life in the "plastisphere": Microbial communities on plastic marine debris. Environ Sci Technol 47: 7137-7146. doi: 10.1021/es401288x
![]() |
[6] |
McCormick A, Hoellein TJ, Mason SA, et al. (2014) Microplastic is an Abundant and Distinct Microbial Habitat in an Urban River. Environ Sci Technol 48: 11863-11871. doi: 10.1021/es503610r
![]() |
[7] |
Chen X, Xiong X, Jiang X, et al. (2019) Sinking of floating plastic debris caused by biofilm development in a freshwater lake. Chemosphere 222: 856-864. doi: 10.1016/j.chemosphere.2019.02.015
![]() |
[8] |
Van Cauwenberghe L, Vanreusel A, Mees J, et al. (2013) Microplastic pollution in deep-sea sediments. Environ Pollut 182: 495-499. doi: 10.1016/j.envpol.2013.08.013
![]() |
[9] |
da Silva BF, Jelic A, López-serna R, et al. (2011) Occurrence and distribution of pharmaceuticals in surface water, suspended solids and sediments of the Ebro river basin, Spain. Chemosphere 85: 1331-1339. doi: 10.1016/j.chemosphere.2011.07.051
![]() |
[10] |
Wolfson SJ, Porter AW, Campbell JK, et al. (2018) Naproxen Is Transformed Via Acetogenesis and Syntrophic Acetate Oxidation by a Methanogenic Wastewater Consortium. Microb Ecol 76: 362-371. doi: 10.1007/s00248-017-1136-2
![]() |
[11] |
Wolfson SJ, Porter AW, Villani TS, et al. (2019) Pharmaceuticals and Personal Care Products can be Transformed by Anaerobic Microbiomes in the Environment and in Waste Treatment Processes. Environ Toxicol Chem 38: 1585-1593. doi: 10.1002/etc.4406
![]() |
[12] |
Chang M (2015) Reducing microplastics from facial exfoliating cleansers in wastewater through treatment versus consumer product decisions. Mar Pollut Bull 101: 330-333. doi: 10.1016/j.marpolbul.2015.10.074
![]() |
[13] |
He Z, Minteer SD, Angenent LT (2005) Electricity generation from artificial wastewater using an upflow microbial fuel cell. Environ Sci Technol 39: 5262-5267. doi: 10.1021/es0502876
![]() |
[14] |
Healy JB, Young LY (1979) Anaerobic Biodegradation of Eleven Aromatic Compounds Anaerobic Biodegradation of Eleven Aromatic Compounds to Methane. Appl Environ Microbiol 38: 84-89. doi: 10.1128/AEM.38.1.84-89.1979
![]() |
[15] |
Owen WF, Stuckey DC, Healy JB, et al. (1979) Bioassay for monitoring biochemical methane potential and anaerobic toxicity. Water Res 13: 485-492. doi: 10.1016/0043-1354(79)90043-5
![]() |
[16] |
Kerkhof L, Ward BB (1993) Comparison of Nucleic Acid Hybridization and Fluorometry for Measurement of the Relationship between RNA/DNA Ratio and Growth Rate in a Marine Bacterium. Appl Environ Microbiol 59: 1303-1309. doi: 10.1128/AEM.59.5.1303-1309.1993
![]() |
[17] |
Wang Q, Garrity G, Tiedje J, et al. (2007) Naïve Bayesian classifier for rapid assignment of rRNA sequences into the new bacterial taxonomy. Appl Environ Microbiol 73: 5261-5267. doi: 10.1128/AEM.00062-07
![]() |
[18] |
Altschul S, Gish W, Miller W, et al. (1990) Basic local alignment search tool. J Mol Biol 215: 403-410. doi: 10.1016/S0022-2836(05)80360-2
![]() |
[19] |
Lahti M, Oikari A (2011) Microbial transformation of pharmaceuticals Naproxen Bisoprolol, and Diclofenac in aerobic and anaerobic environments. Arch Environ Contam Toxicol 61: 202-210. doi: 10.1007/s00244-010-9622-2
![]() |
[20] | Hedderich R, Whitman WB (2006) Physiology and Biochemistry of the Methane-Producing Archaea. Prokaryotes 2: 1050-1079. |
[21] |
Pinnell LJ, Turner JW (2019) Shotgun metagenomics reveals the benthic microbial community response to plastic and bioplastic in a coastal marine environment. Front Microbiol 10: 1252. doi: 10.3389/fmicb.2019.01252
![]() |
[22] | Kuever J, Rainey FA, Widdel F (2014) The family Desulfobacteraceae. The Prokaryotes 10: 45-73. |
[23] |
Leadbetter JR, Schmidt TM, Graber JR, et al. (1999) Acetogenesis from H2 plus CO2 by spirochetes from termite guts. Science 283: 686-689. doi: 10.1126/science.283.5402.686
![]() |
[24] | Westerholm M, Roos S, Schnurer A (2010) Syntrophaceticus schinkii gen. nov., sp. nov., an anaerobic, syntrophic acetate-oxidizing bacterium isolated from a mesophilic anaerobic filter. FEMS Microbiol Lett 309: 100-104. |
[25] |
Kaufmann F, Wohlfarth G, Diekert G (1998) O-Demethylase from Acetobacterium dehalogenans Cloning, sequencing, and active expression of the gene encoding the corrinoid protein. Eur J Biochem 257: 515-521. doi: 10.1046/j.1432-1327.1998.2570515.x
![]() |
[26] | Drake H, Küsel K, Matthies C (2006) Acetogenic Prokaryotes. Prokaryotes 2: 354-420. |
[27] |
Zhang L, Lyu T, Ramírez Vargas CA, et al. (2018) New insights into the effects of support matrix on the removal of organic micro-pollutants and the microbial community in constructed wetlands. Environ Pollut 240: 699-708. doi: 10.1016/j.envpol.2018.05.028
![]() |
[28] |
Langer S, Schropp D, Bengelsdorf FR, et al. (2014) Dynamics of biofilm formation during anaerobic digestion of organic waste. Anaerobe 29: 44-51. doi: 10.1016/j.anaerobe.2013.11.013
![]() |
[29] |
Habouzit F, Gévaudan G, Hamelin J, et al. (2011) Influence of support material properties on the potential selection of Archaea during initial adhesion of a methanogenic consortium. Bioresour Technol 102: 4054-4060. doi: 10.1016/j.biortech.2010.12.023
![]() |
[30] |
Huerta B, Rodriguez-Mozaz S, Nannou C, et al. (2016) Determination of a broad spectrum of pharmaceuticals and endocrine disruptors in biofilm from a waste water treatment plant-impacted river. Sci Total Environ 540: 241-249. doi: 10.1016/j.scitotenv.2015.05.049
![]() |
[31] |
Zhu Z, Wang S, Zhao F, et al. (2019) Joint toxicity of microplastics with triclosan to marine microalgae Skeletonema costatum. Environ Pollut 246: 509-517. doi: 10.1016/j.envpol.2018.12.044
![]() |
[32] |
Zinder SH, Koch M (1984) Non-aceticlastic methanogenesis from acetate: acetate oxidation by a thermophilic syntrophic coculture. Arch Microbiol 138: 263-272. doi: 10.1007/BF00402133
![]() |
[33] |
Wüst PK, Horn MA, Drake HL (2009) Trophic links between fermenters and methanogens in a moderately acidic fen soil. Environ Microbiol 11: 1395-1409. doi: 10.1111/j.1462-2920.2009.01867.x
![]() |
[34] |
Bengelsdorf FR, Gabris C, Michel L, et al. (2015) Syntrophic microbial communities on straw as biofilm carrier increase the methane yield of a biowaste-digesting biogas reactor. AIMS Bioeng 2: 264-276. doi: 10.3934/bioeng.2015.3.264
![]() |
[35] |
Hossain MR, Jiang M, Wei Q, et al. (2019) Microplastic surface properties affect bacterial colonization in freshwater. J Basic Microbiol 59: 54-61. doi: 10.1002/jobm.201800174
![]() |
[36] | Kirstein I V, Kirmizi S, Wichels A, et al. (2016) Dangerous hitchhikers? Evidence for potentially pathogenic Vibrio spp. on microplastic particles. Mar Environ Res 120: 1-8. |
[37] |
Parrish K, Fahrenfeld NL (2019) Microplastic biofilm in fresh-and wastewater as a function of microparticle type and size class. Environ Sci Water Res Technol. 5: 495-505. doi: 10.1039/C8EW00712H
![]() |
[38] | Cheng L, Ding C, Li Q, et al. (2013) DNA-SIP Reveals That Syntrophaceae Play an Important Role in Methanogenic Hexadecane Degradation. PLoS One 8: 1-11. |
[39] |
Nobu MK, Narihiro T, Hideyuki T, et al. (2015) The genome of Syntrophorhabdus aromaticivorans strain UI provides new insights for syntrophic aromatic compound metabolism and electron flow. Environ Microbiol 17: 4861-4872. doi: 10.1111/1462-2920.12444
![]() |
1. | William Boni, Kathleen Parrish, Shreya Patil, Nicole L. Fahrenfeld, Total coliform and Escherichia coli in microplastic biofilms grown in wastewater and inactivation by peracetic acid , 2021, 93, 1061-4303, 334, 10.1002/wer.1434 | |
2. | A. Merline Sheela, B. Manimekalai, G. Dhinagaran, Review on the distribution of microplastics in the oceans and its impacts: Need for modeling-based approach to investigate the transport and risk of microplastic pollution, 2021, 27, 1226-1025, 210243, 10.4491/eer.2021.243 | |
3. | Xiaodan Wang, Xiaoli Zhang, Cheng Yao, Encui Shan, Xiaojing Lv, Jia Teng, Jianmin Zhao, Qing Wang, Impact of aged and virgin microplastics on sedimentary nitrogen cycling and microbial ecosystems in estuaries, 2023, 878, 00489697, 162977, 10.1016/j.scitotenv.2023.162977 | |
4. | Taishan Ran, Hongkai Liao, Yuxin Zhao, Juan Li, Soil plastisphere interferes with soil bacterial community and their functions in the rhizosphere of pepper (Capsicum annuum L.), 2024, 270, 01476513, 115946, 10.1016/j.ecoenv.2024.115946 | |
5. | Alina Dereszewska, Katarzyna Krasowska, Marzenna Popek, Determination of Selected Organic Contaminants in the Port of Gdynia Sediments: Towards Cleaner Baltic Ports, 2024, 16, 2071-1050, 5285, 10.3390/su16135285 | |
6. | Nicoly Subtil de Oliveira, Gabriel Pereira Lopes da Silva, Orozimbo Furlan, Lorena Carolina Peña, Luiz Fernando Bianchini, Nipuna Parahitiyawa, Edvaldo Antonio Ribeiro Rosa, The song remains the same. The lab bench dilemma of using shaken flasks in microbial biotransformation experiments, 2024, 42, 1024-2422, 565, 10.1080/10242422.2023.2284116 | |
7. | Elisa Clagnan, Mirko Cucina, Raveena Vilas Sajgule, Patrizia De Nisi, Fabrizio Adani, Microbial community acclimatization enhances bioplastics biodegradation and biogas production under thermophilic anaerobic digestion, 2023, 390, 09608524, 129889, 10.1016/j.biortech.2023.129889 |
Microplastic-Amended Cultures | Naproxen | DMN | Methane |
Active Cultures | 7.91 ± 0.916 | 8.21 ± 0.924 | 5.38 ± 2.41 |
Sterile Controls | n.d. | n.d. | 1.45 ± 0.131 |
Background Controls | 0.0313 ± 0.024 | 0.0285 ± 0.021 | 3.00 ± 0.281 |
Planktonic Cultures | Naproxen | DMN | Methane |
Active Cultures | 7.55 ± 0.653 | 7.79 ± 0.24 | 1.65 ± 1.349 |
Sterile Controls | n.d. | n.d. | n.d. |
Background Controls | 0.0485 ± 0.0912 | 0.0158 ± 0.00115 | 3.32 ± 0.471 |
Microplastic-Amended Cultures | Naproxen | DMN | Methane |
Active Cultures | 7.91 ± 0.916 | 8.21 ± 0.924 | 5.38 ± 2.41 |
Sterile Controls | n.d. | n.d. | 1.45 ± 0.131 |
Background Controls | 0.0313 ± 0.024 | 0.0285 ± 0.021 | 3.00 ± 0.281 |
Planktonic Cultures | Naproxen | DMN | Methane |
Active Cultures | 7.55 ± 0.653 | 7.79 ± 0.24 | 1.65 ± 1.349 |
Sterile Controls | n.d. | n.d. | n.d. |
Background Controls | 0.0485 ± 0.0912 | 0.0158 ± 0.00115 | 3.32 ± 0.471 |